- NAEYC Login
- Member Profile
- Hello Community
- Accreditation Portal
- Online Learning
- Online Store
Popular Searches: DAP ; Coping with COVID-19 ; E-books ; Anti-Bias Education ; Online Store

21st Century Teaching and Learning
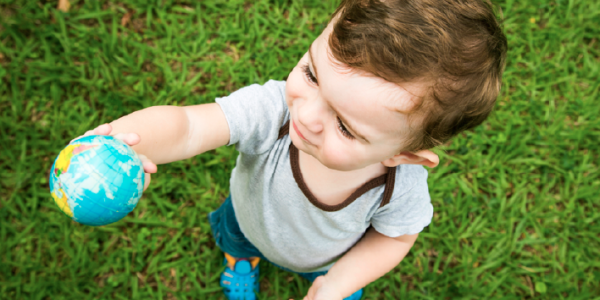
You are here
As a 20th century kid, I was awed by the mere mention of the twenty- first century. The optimist in me envisioned a 21st century that was sleek, modern, and bursting with gadgets that stretched the imagination. Although my dreams of flying cars and room-cleaning robots have not been realized just yet, the gadgets have not disappointed. The impact of abundant and ever-changing technology—particularly information and communication technology—frequently dominates conversations in and about modern society. The same holds true in the field of education, where technology integration and emphasis of the disciplines most closely associated with modern technologies (i.e., science, math, and engineering) are seen as vital. These are important considerations, but 21st century teaching and learning goes beyond technology integration and STEM content; it is also about fostering ways of thinking and promoting dispositions that support success in an age driven by rapidly changing and expanding technologies. Responsive 21st century teaching and caregiving requires educators to create environments and provide experiences that encourage exploration and inquiry, and nurture creativity and curiosity.
The July 2016 issue of Young Children celebrates and explores this 21st century approach to teaching and learning. The cluster articles provide a snapshot of the developmentally appropriate ways the needs of young children growing up today are being addressed.
Some of the most prominent components of 21st century education—problem solving, critical thinking, collaboration, authentic learning, appropriate use of technologies, and cross-disciplinary teaching—are the focus in “Integrating the Curriculum to Engage and Challenge Children.” Geared toward practice in kindergarten through third grade, the article by Barbara A. Bradley discusses the ways in which educators of primary school children can incorporate these components in their teaching.
Information and communication technology is changing the way we get information and interact with each other. This is particularly true about social media. In “Are You (P)Interested in 21st Century Teaching and Learning?,” Rachael Huber and C.C. Bates provide an introduction to this popular social media platform and explore one of the new ways teachers are locating and sharing information.
Tracey Hunter-Doniger demonstrates the power of creativity and arts infusion in “ Snapdragons and Math: Using Creativity to Inspire, Motivate, and Engage .” Tracey describes the successful efforts of a kindergarten teacher and art educator who designed a cross-curricular collaboration aimed at promoting children’s engagement and enhancing learning.
One of the benefits of the ever-increasing availability of new technology is a shrinking world. In light of our global society, it is essential that crosscultural understanding be fostered in 21st century early childhood education settings. In “Classroom Contexts That Support Young Children’s Intercultural Understanding,” María V. Acevedo explores the efforts used to bridge gaps between a group of preschool teachers’ existing practices and the needs of the children in their classrooms.
In “ Beyond Bouncing the Ball: Toddlers and Teachers Investigate Physics ,” Eric Bucher and Marcos Hernández show us how topics that were once considered beyond the bounds of early childhood classrooms are now being introduced in developmentally appropriate ways. Bucher and Hernández position teachers as reflective co-investigators, a departure from the more traditional view of teachers as disseminators of information. The authors describe the educators in their article as teacher researchers and present a process that was implemented to promote reflective practice.
The National Association for the Education of Young Children has long embraced teacher research—teachers’ systematic inquiry of their practice—and its potential for advancing the profession and promoting high-quality early childhood education. Since 2004, NAEYC has published Voices of Practitioners , the only teacher research journal dedicated to early childhood education. To bring teacher research to a wider audience, a new Voices of Practitioners article will be published regularly in Young Children, beginning with this issue. We are excited to continue promoting teacher research and to make this valuable resource available in print.
Finally, we are pleased that this particular cluster coincides with the debut of Growing in STEM, a new column focusing on developmentally appropriate practice related to early childhood science, technology, engineering, and mathematics. Reflecting a 21st century-minded pedagogy premised on inquiry and integration, the column promises to support early childhood educators at all levels as they seek to enhance STEM teaching and learning in their classrooms.
We hope you find something in this exciting issue that inspires the 21st century educator in you! – M. Deanna Ramey, Editor in Chief
Photograph: © iStock
M. Deanna Ramey was formerly editor-in-chief of Young Children .
Vol. 71, No. 3
Print this article
- Position paper
- Open access
- Published: 28 November 2019
Physics education research for 21 st century learning
- Lei Bao ORCID: orcid.org/0000-0003-3348-4198 1 &
- Kathleen Koenig 2
Disciplinary and Interdisciplinary Science Education Research volume 1 , Article number: 2 ( 2019 ) Cite this article
38k Accesses
74 Citations
2 Altmetric
Metrics details
Education goals have evolved to emphasize student acquisition of the knowledge and attributes necessary to successfully contribute to the workforce and global economy of the twenty-first Century. The new education standards emphasize higher end skills including reasoning, creativity, and open problem solving. Although there is substantial research evidence and consensus around identifying essential twenty-first Century skills, there is a lack of research that focuses on how the related subskills interact and develop over time. This paper provides a brief review of physics education research as a means for providing a context towards future work in promoting deep learning and fostering abilities in high-end reasoning. Through a synthesis of the literature around twenty-first Century skills and physics education, a set of concretely defined education and research goals are suggested for future research, along with how these may impact the next generation physics courses and how physics should be taught in the future.
Introduction
Education is the primary service offered by society to prepare its future generation workforce. The goals of education should therefore meet the demands of the changing world. The concept of learner-centered, active learning has broad, growing support in the research literature as an empirically validated teaching practice that best promotes learning for modern day students (Freeman et al., 2014 ). It stems out of the constructivist view of learning, which emphasizes that it is the learner who needs to actively construct knowledge and the teacher should assume the role of a facilitator rather than the source of knowledge. As implied by the constructivist view, learner-centered education usually emphasizes active-engagement and inquiry style teaching-learning methods, in which the learners can effectively construct their understanding under the guidance of instruction. The learner-centered education also requires educators and researchers to focus their efforts on the learners’ needs, not only to deliver effective teaching-learning approaches, but also to continuously align instructional practices to the education goals of the times. The goals of introductory college courses in science, technology, engineering, and mathematics (STEM) disciplines have constantly evolved from some notion of weed-out courses that emphasize content drilling, to the current constructivist active-engagement type of learning that promotes interest in STEM careers and fosters high-end cognitive abilities.
Following the conceptually defined framework of twenty-first Century teaching and learning, this paper aims to provide contextualized operational definitions of the goals for twenty-first Century learning in physics (and STEM in general) as well as the rationale for the importance of these outcomes for current students. Aligning to the twenty-first Century learning goals, research in physics education is briefly reviewed to provide a context towards future work in promoting deep learning and fostering abilities in high-end reasoning in parallel. Through a synthesis of the literature around twenty-first Century skills and physics education, a set of concretely defined education and research goals are suggested for future research. These goals include: domain-specific research in physics learning; fostering scientific reasoning abilities that are transferable across the STEM disciplines; and dissemination of research-validated curriculum and approaches to teaching and learning. Although this review has a focus on physics education research (PER), it is beneficial to expand the perspective to view physics education in the broader context of STEM learning. Therefore, much of the discussion will blend PER with STEM education as a continuum body of work on teaching and learning.
Education goals for twenty-first century learning
Education goals have evolved to emphasize student acquisition of essential “21 st Century skills”, which define the knowledge and attributes necessary to successfully contribute to the workforce and global economy of the 21st Century (National Research Council, 2011 , 2012a ). In general, these standards seek to transition from emphasizing content-based drilling and memorization towards fostering higher-end skills including reasoning, creativity, and open problem solving (United States Chamber of Commerce, 2017 ). Initiatives on advancing twenty-first Century education focus on skills that converge on three broad clusters: cognitive, interpersonal, and intrapersonal, all of which include a rich set of sub-dimensions.
Within the cognitive domain, multiple competencies have been proposed, including deep learning, non-routine problem solving, systems thinking, critical thinking, computational and information literacy, reasoning and argumentation, and innovation (National Research Council, 2012b ; National Science and Technology Council, 2018 ). Interpersonal skills are those necessary for relating to others, including the ability to work creatively and collaboratively as well as communicate clearly. Intrapersonal skills, on the other hand, reside within the individual and include metacognitive thinking, adaptability, and self-management. These involve the ability to adjust one’s strategy or approach along with the ability to work towards important goals without significant distraction, both essential for sustained success in long-term problem solving and career development.
Although many descriptions exist for what qualifies as twenty-first Century skills, student abilities in scientific reasoning and critical thinking are the most commonly noted and widely studied. They are highly connected with the other cognitive skills of problem solving, decision making, and creative thinking (Bailin, 1996 ; Facione, 1990 ; Fisher, 2001 ; Lipman, 2003 ; Marzano et al., 1988 ), and have been important educational goals since the 1980s (Binkley et al., 2010 ; NCET, 1987 ). As a result, they play a foundational role in defining, assessing, and developing twenty-first Century skills.
The literature for critical thinking is extensive (Bangert-Drowns & Bankert, 1990 ; Facione, 1990 ; Glaser, 1941 ). Various definitions exist with common underlying principles. Broadly defined, critical thinking is the application of the cognitive skills and strategies that aim for and support evidence-based decision making. It is the thinking involved in solving problems, formulating inferences, calculating likelihoods, and making decisions (Halpern, 1999 ). It is the “reasonable reflective thinking focused on deciding what to believe or do” (Ennis, 1993 ). Critical thinking is recognized as a way to understand and evaluate subject matter; producing reliable knowledge and improving thinking itself (Paul, 1990 ; Siegel, 1988 ).
The notion of scientific reasoning is often used to label the set of skills that support critical thinking, problem solving, and creativity in STEM. Broadly defined, scientific reasoning includes the thinking and reasoning skills involved in inquiry, experimentation, evidence evaluation, inference and argument that support the formation and modification of concepts and theories about the natural world; such as the ability to systematically explore a problem, formulate and test hypotheses, manipulate and isolate variables, and observe and evaluate consequences (Bao et al., 2009 ; Zimmerman, 2000 ). Critical thinking and scientific reasoning share many features, where both emphasize evidence-based decision making in multivariable causal conditions. Critical thinking can be promoted through the development of scientific reasoning, which includes student ability to reach a reliable conclusion after identifying a question, formulating hypotheses, gathering relevant data, and logically testing and evaluating the hypothesis. In this way, scientific reasoning can be viewed as a scientific domain instantiation of critical thinking in the context of STEM learning.
In STEM learning, cognitive aspects of the twenty-first Century skills aim to develop reasoning skills, critical thinking skills, and deep understanding, all of which allow students to develop well connected expert-like knowledge structures and engage in meaningful scientific inquiry and problem solving. Within physics education, a core component of STEM education, the learning of conceptual understanding and problem solving remains a current emphasis. However, the fast-changing work environment and technology-driven world require a new set of core knowledge, skills, and habits of mind to solve complex interdisciplinary problems, gather and evaluate evidence, and make sense of information from a variety of sources (Tanenbaum, 2016 ). The education goals in physics are transitioning towards ability fostering as well as extension and integration with other STEM disciplines. Although curriculum that supports these goals is limited, there are a number of attempts, particularly in developing active learning classrooms and inquiry-based laboratory activities, which have demonstrated success. Some of these are described later in this paper as they provide a foundation for future work in physics education.
Interpersonal skills, such as communication and collaboration, are also essential for twenty-first Century problem-solving tasks, which are often open-ended, complex, and team-based. As the world becomes more connected in a multitude of dimensions, tackling significant problems involving complex systems often goes beyond the individual and requires working with others who are increasingly from culturally diverse backgrounds. Due to the rise of communication technologies, being able to articulate thoughts and ideas in a variety of formats and contexts is crucial, as well as the ability to effectively listen or observe to decipher meaning. Interpersonal skills can be promoted by integrating group-learning experiences into the classroom setting, while providing students with the opportunity to engage in open-ended tasks with a team of peer learners who may propose more than one plausible solution. These experiences should be designed such that students must work collaboratively and responsibly in teams to develop creative solutions, which are later disseminated through informative presentations and clearly written scientific reports. Although educational settings in general have moved to providing students with more and more opportunities for collaborative learning, a lack of effective assessments for these important skills has been a limiting factor for producing informative research and widespread implementation. See Liu ( 2010 ) for an overview of measurement instruments reported in the research literature.
Intrapersonal skills are based on the individual and include the ability to manage one’s behavior and emotions to achieve goals. These are especially important for adapting in the fast-evolving collaborative modern work environment and for learning new tasks to solve increasingly challenging interdisciplinary problems, both of which require intellectual openness, work ethic, initiative, and metacognition, to name a few. These skills can be promoted using instruction which, for example, includes metacognitive learning strategies, provides opportunities to make choices and set goals for learning, and explicitly connects to everyday life events. However, like interpersonal skills, the availability of relevant assessments challenges advancement in this area. In this review, the vast amount of studies on interpersonal and intrapersonal skills will not be discussed in order to keep the main focus on the cognitive side of skills and reasoning.
The purpose behind discussing twenty-first Century skills is that this set of skills provides important guidance for establishing essential education goals for modern society and learners. However, although there is substantial research evidence and consensus around identifying necessary twenty-first Century skills, there is a lack of research that focuses on how the related subskills interact and develop over time (Reimers & Chung, 2016 ), with much of the existing research residing in academic literature that is focused on psychology rather than education systems (National Research Council, 2012a ). Therefore, a major and challenging task for discipline-based education researchers and educators is to operationally define discipline-specific goals that align with the twenty-first Century skills for each of the STEM fields. In the following sections, this paper will provide a limited vision of the research endeavors in physics education that can translate the past and current success into sustained impact for twenty-first Century teaching and learning.
Proposed education and research goals
Physics education research (PER) is often considered an early pioneer in discipline-based education research (National Research Council, 2012c ), with well-established, broad, and influential outcomes (e.g., Hake, 1998 ; Hsu, Brewe, Foster, & Harper, 2004 ; McDermott & Redish, 1999 ; Meltzer & Thornton, 2012 ). Through the integration of twenty-first Century skills with the PER literature, a set of broadly defined education and research goals is proposed for future PER work:
Discipline-specific deep learning: Cognitive and education research involving physics learning has established a rich literature on student learning behaviors along with a number of frameworks. Some of the popular frameworks include conceptual understanding and concept change, problem solving, knowledge structure, deep learning, and knowledge integration. Aligned with twenty-first Century skills, future research in physics learning should aim to integrate the multiple areas of existing work, such that they help students develop well integrated knowledge structures in order to achieve deep leaning in physics.
Fostering scientific reasoning for transfer across STEM disciplines: The broad literature in physics learning and scientific reasoning can provide a solid foundation to further develop effective physics education approaches, such as active engagement instruction and inquiry labs, specifically targeting scientific inquiry abilities and reasoning skills. Since scientific reasoning is a more domain-general cognitive ability, success in physics can also more readily inform research and education practices in other STEM fields.
Research, development, assessment, and dissemination of effective education approaches: Developing and maintaining a supportive infrastructure of education research and implementation has always been a challenge, not only in physics but in all STEM areas. The twenty-first Century education requires researchers and instructors across STEM to work together as an extended community in order to construct a sustainable integrated STEM education environment. Through this new infrastructure, effective team-based inquiry learning and meaningful assessment can be delivered to help students develop a comprehensive skills set including deep understanding and scientific reasoning, as well as communication and other non-cognitive abilities.
The suggested research will generate understanding and resources to support education practices that meet the requirements of the Next Generation Science Standards (NGSS), which explicitly emphasize three areas of learning including disciplinary core ideas, crosscutting concepts, and practices (National Research Council, 2012b ). The first goal for promoting deep learning of disciplinary knowledge corresponds well to the NGSS emphasis on disciplinary core ideas, which play a central role in helping students develop well integrated knowledge structures to achieve deep understanding. The second goal on fostering transferable scientific reasoning skills supports the NGSS emphasis on crosscutting concepts and practices. Scientific reasoning skills are crosscutting cognitive abilities that are essential to the development of domain-general concepts and modeling strategies. In addition, the development of scientific reasoning requires inquiry-based learning and practices. Therefore, research on scientific reasoning can produce a valuable knowledge base on education means that are effective for developing crosscutting concepts and promoting meaningful practices in STEM. The third research goal addresses the challenge in the assessment of high-end skills and the dissemination of effective educational approaches, which supports all NGSS initiatives to ensure sustainable development and lasting impact. The following sections will discuss the research literature that provides the foundation for these three research goals and identify the specific challenges that will need to be addressed in future work.
Promoting deep learning in physics education
Physics education for the twenty-first Century aims to foster high-end reasoning skills and promote deep conceptual understanding. However, many traditional education systems place strong emphasis on only problem solving with the expectation that students obtain deep conceptual understanding through repetitive problem-solving practices, which often doesn’t occur (Alonso, 1992 ). This focus on problem solving has been shown to have limitations as a number of studies have revealed disconnections between learning conceptual understanding and problem-solving skills (Chiu, 2001 ; Chiu, Guo, & Treagust, 2007 ; Hoellwarth, Moelter, & Knight, 2005 ; Kim & Pak, 2002 ; Nakhleh, 1993 ; Nakhleh & Mitchell, 1993 ; Nurrenbern & Pickering, 1987 ; Stamovlasis, Tsaparlis, Kamilatos, Papaoikonomou, & Zarotiadou, 2005 ). In fact, drilling in problem solving may actually promote memorization of context-specific solutions with minimal generalization rather than transitioning students from novices to experts.
Towards conceptual understanding and learning, many models and definitions have been established to study and describe student conceptual knowledge states and development. For example, students coming into a physics classroom often hold deeply rooted, stable understandings that differ from expert conceptions. These are commonly referred to as misconceptions or alternative conceptions (Clement, 1982 ; Duit & Treagust, 2003 ; Dykstra Jr, Boyle, & Monarch, 1992 ; Halloun & Hestenes, 1985a , 1985b ). Such students’ conceptions are context dependent and exist as disconnected knowledge fragments, which are strongly situated within specific contexts (Bao & Redish, 2001 , 2006 ; Minstrell, 1992 ).
In modeling students’ knowledge structures, DiSessa’s proposed phenomenological primitives (p-prim) describe a learner’s implicit thinking, cued from specific contexts, as an underpinning cognitive construct for a learner’s expressed conception (DiSessa, 1993 ; Smith III, DiSessa, & Roschelle, 1994 ). Facets, on the other hand, map between the implicit p-prim and concrete statements of beliefs and are developed as discrete and independent units of thought, knowledge, or strategies used by individuals to address specific situations (Minstrell, 1992 ). Ontological categories, defined by Chi, describe student reasoning in the most general sense. Chi believed that these are distinct, stable, and constraining, and that a core reason behind novices’ difficulties in physics is that they think of physics within the category of matter instead of processes (Chi, 1992 ; Chi & Slotta, 1993 ; Chi, Slotta, & De Leeuw, 1994 ; Slotta, Chi, & Joram, 1995 ). More details on conceptual learning and problem solving are well summarized in the literature (Hsu et al., 2004 ; McDermott & Redish, 1999 ), from which a common theme emerges from the models and definitions. That is, learning is context dependent and students with poor conceptual understanding typically have locally connected knowledge structures with isolated conceptual constructs that are unable to establish similarities and contrasts between contexts.
Additionally, this idea of fragmentation is demonstrated through many studies on student problem solving in physics and other fields. It has been shown that a student’s knowledge organization is a key aspect for distinguishing experts from novices (Bagno, Eylon, & Ganiel, 2000 ; Chi, Feltovich, & Glaser, 1981 ; De Jong & Ferguson-Hesler, 1986 ; Eylon & Reif, 1984 ; Ferguson-Hesler & De Jong, 1990 ; Heller & Reif, 1984 ; Larkin, McDermott, Simon, & Simon, 1980 ; Smith, 1992 ; Veldhuis, 1990 ; Wexler, 1982 ). Expert’s knowledge is organized around core principles of physics, which are applied to guide problem solving and develop connections between different domains as well as new, unfamiliar situations (Brown, 1989 ; Perkins & Salomon, 1989 ; Salomon & Perkins, 1989 ). Novices, on the other hand, lack a well-organized knowledge structure and often solve problems by relying on surface features that are directly mapped to certain problem-solving outcomes through memorization (Chi, Bassok, Lewis, Reimann, & Glaser, 1989 ; Hardiman, Dufresne, & Mestre, 1989 ; Schoenfeld & Herrmann, 1982 ).
This lack of organization creates many difficulties in the comprehension of basic concepts and in solving complex problems. This leads to the common complaint that students’ knowledge of physics is reduced to formulas and vague labels of the concepts, which are unable to substantively contribute to meaningful reasoning processes. A novice’s fragmented knowledge structure severely limits the learner’s conceptual understanding. In essence, these students are able to memorize how to approach a problem given specific information but lack the understanding of the underlying concept of the approach, limiting their ability to apply this approach to a novel situation. In order to achieve expert-like understanding, a student’s knowledge structure must integrate all of the fragmented ideas around the core principle to form a coherent and fully connected conceptual framework.
Towards a more general theoretical consideration, students’ alternative conceptions and fragmentation in knowledge structures can be viewed through both the “naïve theory” framework (e.g., Posner, Strike, Hewson, & Gertzog, 1982 ; Vosniadou, Vamvakoussi, & Skopeliti, 2008 ) and the “knowledge in pieces” (DiSessa, 1993 ) perspective. The “naïve theory” framework considers students entering the classroom with stable and coherent ideas (naïve theories) about the natural world that differ from those presented by experts. In the “knowledge in pieces” perspective, student knowledge is constructed in real-time and incorporates context features with the p-prims to form the observed conceptual expressions. Although there exists an ongoing debate between these two views (Kalman & Lattery, 2018 ), it is more productive to focus on their instructional implications for promoting meaningful conceptual change in students’ knowledge structures.
In the process of learning, students may enter the classroom with a range of initial states depending on the population and content. For topics with well-established empirical experiences, students often have developed their own ideas and understanding, while on topics without prior exposure, students may create their initial understanding in real-time based on related prior knowledge and given contextual features (Bao & Redish, 2006 ). These initial states of understanding, regardless of their origin, are usually different from those of experts. Therefore, the main function of teaching and learning is to guide students to modify their initial understanding towards the experts’ views. Although students’ initial understanding may exist as a body of coherent ideas within limited contexts, as students start to change their knowledge structures throughout the learning process, they may evolve into a wide range of transitional states with varying levels of knowledge integration and coherence. The discussion in this brief review on students’ knowledge structures regarding fragmentation and integration are primarily focused on the transitional stages emerged through learning.
The corresponding instructional goal is then to help students more effectively develop an integrated knowledge structure so as to achieve a deep conceptual understanding. From an educator’s perspective, Bloom’s taxonomy of education objectives establishes a hierarchy of six levels of cognitive skills based on their specificity and complexity: Remember (lowest and most specific), Understand, Apply, Analyze, Evaluate, and Create (highest and most general and complex) (Anderson et al., 2001 ; Bloom, Engelhart, Furst, Hill, & Krathwohl, 1956 ). This hierarchy of skills exemplifies the transition of a learner’s cognitive development from a fragmented and contextually situated knowledge structure (novice with low level cognitive skills) to a well-integrated and globally networked expert-like structure (with high level cognitive skills).
As a student’s learning progresses from lower to higher cognitive levels, the student’s knowledge structure becomes more integrated and is easier to transfer across contexts (less context specific). For example, beginning stage students may only be able to memorize and perform limited applications of the features of certain contexts and their conditional variations, with which the students were specifically taught. This leads to the establishment of a locally connected knowledge construct. When a student’s learning progresses from the level of Remember to Understand, the student begins to develop connections among some of the fragmented pieces to form a more fully connected network linking a larger set of contexts, thus advancing into a higher level of understanding. These connections and the ability to transfer between different situations form the basis of deep conceptual understanding. This growth of connections leads to a more complete and integrated cognitive structure, which can be mapped to a higher level on Bloom’s taxonomy. This occurs when students are able to relate a larger number of different contextual and conditional aspects of a concept for analyzing and evaluating to a wider variety of problem situations.
Promoting the growth of connections would appear to aid in student learning. Exactly which teaching methods best facilitate this are dependent on the concepts and skills being learned and should be determined through research. However, it has been well recognized that traditional instruction often fails to help students obtain expert-like conceptual understanding, with many misconceptions still existing after instruction, indicating weak integration within a student’s knowledge structure (McKeachie, 1986 ).
Recognizing the failures of traditional teaching, various research-informed teaching methods have been developed to enhance student conceptual learning along with diagnostic tests, which aim to measure the existence of misconceptions. Most advances in teaching methods focus on the inclusion of inquiry-based interactive-engagement elements in lecture, recitations, and labs. In physics education, these methods were popularized after Hake’s landmark study demonstrated the effectiveness of interactive-engagement over traditional lectures (Hake, 1998 ). Some of these methods include the use of peer instruction (Mazur, 1997 ), personal response systems (e.g., Reay, Bao, Li, Warnakulasooriya, & Baugh, 2005 ), studio-style instruction (Beichner et al., 2007 ), and inquiry-based learning (Etkina & Van Heuvelen, 2001 ; Laws, 2004 ; McDermott, 1996 ; Thornton & Sokoloff, 1998 ). The key approach of these methods aims to improve student learning by carefully targeting deficits in student knowledge and actively encouraging students to explore and discuss. Rather than rote memorization, these approaches help promote generalization and deeper conceptual understanding by building connections between knowledge elements.
Based on the literature, including Bloom’s taxonomy and the new education standards that emphasize twenty-first Century skills, a common focus on teaching and learning can be identified. This focus emphasizes helping students develop connections among fragmented segments of their knowledge pieces and is aligned with the knowledge integration perspective, which focuses on helping students develop and refine their knowledge structure toward a more coherently organized and extensively connected network of ideas (Lee, Liu, & Linn, 2011 ; Linn, 2005 ; Nordine, Krajcik, & Fortus, 2011 ; Shen, Liu, & Chang, 2017 ). For meaningful learning to occur, new concepts must be integrated into a learner’s existing knowledge structure by linking the new knowledge to already understood concepts.
Forming an integrated knowledge structure is therefore essential to achieving deep learning, not only in physics but also in all STEM fields. However, defining what connections must occur at different stages of learning, as well as understanding the instructional methods necessary for effectively developing such connections within each STEM disciplinary context, are necessary for current and future research. Together these will provide the much needed foundational knowledge base to guide the development of the next generation of curriculum and classroom environment designed around twenty-first Century learning.
Developing scientific reasoning with inquiry labs
Scientific reasoning is part of the widely emphasized cognitive strand of twenty-first Century skills. Through development of scientific reasoning skills, students’ critical thinking, open-ended problem-solving abilities, and decision-making skills can be improved. In this way, targeting scientific reasoning as a curricular objective is aligned with the goals emphasized in twenty-first Century education. Also, there is a growing body of research on the importance of student development of scientific reasoning, which have been found to positively correlate with course achievement (Cavallo, Rozman, Blickenstaff, & Walker, 2003 ; Johnson & Lawson, 1998 ), improvement on concept tests (Coletta & Phillips, 2005 ; She & Liao, 2010 ), engagement in higher levels of problem solving (Cracolice, Deming, & Ehlert, 2008 ; Fabby & Koenig, 2013 ); and success on transfer (Ates & Cataloglu, 2007 ; Jensen & Lawson, 2011 ).
Unfortunately, research has shown that college students are lacking in scientific reasoning. Lawson ( 1992 ) found that ~ 50% of intro biology students are not capable of applying scientific reasoning in learning, including the ability to develop hypotheses, control variables, and design experiments; all necessary for meaningful scientific inquiry. Research has also found that traditional courses do not significantly develop these abilities, with pre-to-post-test gains of 1%–2%, while inquiry-based courses have gains around 7% (Koenig, Schen, & Bao, 2012 ; Koenig, Schen, Edwards, & Bao, 2012 ). Others found that undergraduates have difficulty developing evidence-based decisions and differentiating between and linking evidence with claims (Kuhn, 1992 ; Shaw, 1996 ; Zeineddin & Abd-El-Khalick, 2010 ). A large scale international study suggested that learning of physics content knowledge with traditional teaching practices does not improve students’ scientific reasoning skills (Bao et al., 2009 ).
Aligned to twenty-first Century learning, it is important to implement curriculum that is specifically designed for developing scientific reasoning abilities within current education settings. Although traditional lectures may continue for decades due to infrastructure constraints, a unique opportunity can be found in the lab curriculum, which may be more readily transformed to include hands-on minds-on group learning activities that are ideal for developing students’ abilities in scientific inquiry and reasoning.
For well over a century, the laboratory has held a distinctive role in student learning (Meltzer & Otero, 2015 ). However, many existing labs, which haven’t changed much since the late 1980s, have received criticism for their outdated cookbook style that lacks effectiveness in developing high-end skills. In addition, labs have been primarily used as a means for verifying the physical principles presented in lecture, and unfortunately, Hofstein and Lunetta ( 1982 ) found in an early review of the literature that research was unable to demonstrate the impact of the lab on student content learning.
About this same time, a shift towards a constructivist view of learning gained popularity and influenced lab curriculum development towards engaging students in the process of constructing knowledge through science inquiry. Curricula, such as Physics by Inquiry (McDermott, 1996 ), Real-Time Physics (Sokoloff, Thornton, & Laws, 2011 ), and Workshop Physics (Laws, 2004 ), were developed with a primary focus on engaging students in cognitive conflict to address misconceptions. Although these approaches have been shown to be highly successful in improving deep learning of physics concepts (McDermott & Redish, 1999 ), the emphasis on conceptual learning does not sufficiently impact the domain general scientific reasoning skills necessitated in the goals of twenty-first Century learning.
Reform in science education, both in terms of targeted content and skills, along with the emergence of knowledge regarding human cognition and learning (Bransford, Brown, & Cocking, 2000 ), have generated renewed interest in the potential of inquiry-based lab settings for skill development. In these types of hands-on minds-on learning, students apply the methods and procedures of science inquiry to investigate phenomena and construct scientific claims, solve problems, and communicate outcomes, which holds promise for developing both conceptual understanding and scientific reasoning skills in parallel (Trowbridge, Bybee, & Powell, 2000 ). In addition, the availability of technology to enhance inquiry-based learning has seen exponential growth, along with the emergence of more appropriate research methodologies to support research on student learning.
Although inquiry-based labs hold promise for developing students’ high-end reasoning, analytic, and scientific inquiry abilities, these educational endeavors have not become widespread, with many existing physics laboratory courses still viewed merely as a place to illustrate the physical principles from the lecture course (Meltzer & Otero, 2015 ). Developing scientific ideas from practical experiences, however, is a complex process. Students need sufficient time and opportunity for interaction and reflection on complex, investigative tasks. Blended learning, which merges lecture and lab (such as studio style courses), addresses this issue to some extent, but has experienced limited adoption, likely due to the demanding infrastructure resources, including dedicated technology-intensive classroom space, equipment and maintenance costs, and fully committed trained staff.
Therefore, there is an immediate need to transform the existing standalone lab courses, within the constraints of the existing education infrastructure, into more inquiry-based designs, with one of its primary goals dedicated to developing scientific reasoning skills. These labs should center on constructing knowledge, along with hands-on minds-on practical skills and scientific reasoning, to support modeling a problem, designing and implementing experiments, analyzing and interpreting data, drawing and evaluating conclusions, and effective communication. In particular, training on scientific reasoning needs to be explicitly addressed in the lab curriculum, which should contain components specifically targeting a set of operationally-defined scientific reasoning skills, such as ability to control variables or engage in multivariate causal reasoning. Although effective inquiry may also implicitly develop some aspects of scientific reasoning skills, such development is far less efficient and varies with context when the primary focus is on conceptual learning.
Several recent efforts to enhance the standalone lab course have shown promise in supporting education goals that better align with twenty-first Century learning. For example, the Investigative Science Learning Environment (ISLE) labs involve a series of tasks designed to help students develop the “habits of mind” of scientists and engineers (Etkina et al., 2006 ). The curriculum targets reasoning as well as the lab learning outcomes published by the American Association of Physics Teachers (Kozminski et al., 2014 ). Operationally, ISLE methods focus on scaffolding students’ developing conceptual understanding using inquiry learning without a heavy emphasis on cognitive conflict, making it more appropriate and effective for entry level students and K-12 teachers.
Likewise, Koenig, Wood, Bortner, and Bao ( 2019 ) have developed a lab curriculum that is intentionally designed around the twenty-first Century learning goals for developing cognitive, interpersonal, and intrapersonal abilities. In terms of the cognitive domain, the lab learning outcomes center on critical thinking and scientific reasoning but do so through operationally defined sub-skills, all of which are transferrable across STEM. These selected sub-skills are found in the research literature, and include the ability to control variables and engage in data analytics and causal reasoning. For each targeted sub-skill, a series of pre-lab and in-class activities provide students with repeated, deliberate practice within multiple hypothetical science-based scenarios followed by real inquiry-based lab contexts. This explicit instructional strategy has been shown to be essential for the development of scientific reasoning (Chen & Klahr, 1999 ). In addition, the Karplus Learning Cycle (Karplus, 1964 ) provides the foundation for the structure of the lab activities and involves cycles of exploration, concept introduction, and concept application. The curricular framework is such that as the course progresses, the students engage in increasingly complex tasks, which allow students the opportunity to learn gradually through a progression from simple to complex skills.
As part of this same curriculum, students’ interpersonal skills are developed, in part, through teamwork, as students work in groups of 3 or 4 to address open-ended research questions, such as, What impacts the period of a pendulum? In addition, due to time constraints, students learn early on about the importance of working together in an efficient manor towards a common goal, with one set of written lab records per team submitted after each lab. Checkpoints built into all in-class activities involve Socratic dialogue between the instructor and students and promote oral communication. This use of directed questioning guides students in articulating their reasoning behind decisions and claims made, while supporting the development of scientific reasoning and conceptual understanding in parallel (Hake, 1992 ). Students’ intrapersonal skills, as well as communication skills, are promoted through the submission of individual lab reports. These reports require students to reflect upon their learning over each of four multi-week experiments and synthesize their ideas into evidence-based arguments, which support a claim. Due to the length of several weeks over which students collect data for each of these reports, the ability to organize the data and manage their time becomes essential.
Despite the growing emphasis on research and development of curriculum that targets twenty-first Century learning, converting a traditionally taught lab course into a meaningful inquiry-based learning environment is challenging in current reform efforts. Typically, the biggest challenge is a lack of resources; including faculty time to create or adapt inquiry-based materials for the local setting, training faculty and graduate student instructors who are likely unfamiliar with this approach, and the potential cost of new equipment. Koenig et al. ( 2019 ) addressed these potential implementation barriers by designing curriculum with these challenges in mind. That is, the curriculum was designed as a flexible set of modules that target specific sub-skills, with each module consisting of pre-lab (hypothetical) and in-lab (real) activities. Each module was designed around a curricular framework such that an adopting institution can use the materials as written, or can incorporate their existing equipment and experiments into the framework with minimal effort. Other non-traditional approaches have also been experimented with, such as the work by Sobhanzadeh, Kalman, and Thompson ( 2017 ), which targets typical misconceptions by using conceptual questions to engage students in making a prediction, designing and conducting a related experiment, and determining whether or not the results support the hypothesis.
Another challenge for inquiry labs is the assessment of skills-based learning outcomes. For assessment of scientific reasoning, a new instrument on inquiry in scientific thinking analytics and reasoning (iSTAR) has been developed, which can be easily implemented across large numbers of students as both a pre- and post-test to assess gains. iSTAR assesses reasoning skills necessary in the systematical conduct of scientific inquiry, which includes the ability to explore a problem, formulate and test hypotheses, manipulate and isolate variables, and observe and evaluate the consequences (see www.istarassessment.org ). The new instrument expands upon the commonly used classroom test of scientific reasoning (Lawson, 1978 , 2000 ), which has been identified with a number of validity weaknesses and a ceiling effect for college students (Bao, Xiao, Koenig, & Han, 2018 ).
Many education innovations need supporting infrastructures that can ensure adoption and lasting impact. However, making large-scale changes to current education settings can be risky, if not impossible. New education approaches, therefore, need to be designed to adapt to current environmental constraints. Since higher-end skills are a primary focus of twenty-first Century learning, which are most effectively developed in inquiry-based group settings, transforming current lecture and lab courses into this new format is critical. Although this transformation presents great challenges, promising solutions have already emerged from various research efforts. Perhaps the biggest challenge is for STEM educators and researchers to form an alliance to work together to re-engineer many details of the current education infrastructure in order to overcome the multitude of implementation obstacles.
This paper attempts to identify a few central ideas to provide a broad picture for future research and development in physics education, or STEM education in general, to promote twenty-first Century learning. Through a synthesis of the existing literature within the authors’ limited scope, a number of views surface.
Education is a service to prepare (not to select) the future workforce and should be designed as learner-centered, with the education goals and teaching-learning methods tailored to the needs and characteristics of the learners themselves. Given space constraints, the reader is referred to the meta-analysis conducted by Freeman et al. ( 2014 ), which provides strong support for learner-centered instruction. The changing world of the twenty-first Century informs the establishment of new education goals, which should be used to guide research and development of teaching and learning for present day students. Aligned to twenty-first Century learning, the new science standards have set the goals for STEM education to transition towards promoting deep learning of disciplinary knowledge, thereby building upon decades of research in PER, while fostering a wide range of general high-end cognitive and non-cognitive abilities that are transferable across all disciplines.
Following these education goals, more research is needed to operationally define and assess the desired high-end reasoning abilities. Building on a clear definition with effective assessments, a large number of empirical studies are needed to investigate how high-end abilities can be developed in parallel with deep learning of concepts, such that what is learned can be generalized to impact the development of curriculum and teaching methods which promote skills-based learning across all STEM fields. Specifically for PER, future research should emphasize knowledge integration to promote deep conceptual understanding in physics along with inquiry learning to foster scientific reasoning. Integration of physics learning in contexts that connect to other STEM disciplines is also an area for more research. Cross-cutting, interdisciplinary connections are becoming important features of the future generation physics curriculum and defines how physics should be taught collaboratively with other STEM courses.
This paper proposed meaningful areas for future research that are aligned with clearly defined education goals for twenty-first Century learning. Based on the existing literature, a number of challenges are noted for future directions of research, including the need for:
clear and operational definitions of goals to guide research and practice
concrete operational definitions of high-end abilities for which students are expected to develop
effective assessment methods and instruments to measure high-end abilities and other components of twenty-first Century learning
a knowledge base of the curriculum and teaching and learning environments that effectively support the development of advanced skills
integration of knowledge and ability development regarding within-discipline and cross-discipline learning in STEM
effective means to disseminate successful education practices
The list is by no means exhaustive, but these themes emerge above others. In addition, the high-end abilities discussed in this paper focus primarily on scientific reasoning, which is highly connected to other skills, such as critical thinking, systems thinking, multivariable modeling, computational thinking, design thinking, etc. These abilities are expected to develop in STEM learning, although some may be emphasized more within certain disciplines than others. Due to the limited scope of this paper, not all of these abilities were discussed in detail but should be considered an integral part of STEM learning.
Finally, a metacognitive position on education research is worth reflection. One important understanding is that the fundamental learning mechanism hasn’t changed, although the context in which learning occurs has evolved rapidly as a manifestation of the fast-forwarding technology world. Since learning is a process at the interface between a learner’s mind and the environment, the main focus of educators should always be on the learner’s interaction with the environment, not just the environment. In recent education developments, many new learning platforms have emerged at an exponential rate, such as the massive open online courses (MOOCs), STEM creative labs, and other online learning resources, to name a few. As attractive as these may be, it is risky to indiscriminately follow trends in education technology and commercially-incentivized initiatives before such interventions are shown to be effective by research. Trends come and go but educators foster students who have only a limited time to experience education. Therefore, delivering effective education is a high-stakes task and needs to be carefully and ethically planned and implemented. When game-changing opportunities emerge, one needs to not only consider the winners (and what they can win), but also the impact on all that is involved.
Based on a century of education research, consensus has settled on a fundamental mechanism of teaching and learning, which suggests that knowledge is developed within a learner through constructive processes and that team-based guided scientific inquiry is an effective method for promoting deep learning of content knowledge as well as developing high-end cognitive abilities, such as scientific reasoning. Emerging technology and methods should serve to facilitate (not to replace) such learning by providing more effective education settings and conveniently accessible resources. This is an important relationship that should survive many generations of technological and societal changes in the future to come. From a physicist’s point of view, a fundamental relation like this can be considered the “mechanics” of teaching and learning. Therefore, educators and researchers should hold on to these few fundamental principles without being distracted by the surfacing ripples of the world’s motion forward.
Availability of data and materials
Not applicable.
Abbreviations
American Association of Physics Teachers
Investigative Science Learning Environment
Inquiry in Scientific Thinking Analytics and Reasoning
Massive open online course
New Generation Science Standards
- Physics education research
Science Technology Engineering and Math
Alonso, M. (1992). Problem solving vs. conceptual understanding. American Journal of Physics , 60 (9), 777–778. https://doi.org/10.1119/1.17056 .
Article Google Scholar
Anderson, L. W., Krathwohl, D. R., Airasian, P. W., Cruikshank, K. A., Mayer, R. E., Pintrich, P. R., … Wittrock, M. C. (2001). A taxonomy for learning, teaching, and assessing: A revision of Bloom’s taxonomy of educational objectives, abridged edition . White Plains: Longman.
Ates, S., & Cataloglu, E. (2007). The effects of students’ reasoning abilities on conceptual understandings and problem-solving abilities in introductory mechanics. European Journal of Physics , 28 , 1161–1171.
Bagno, E., Eylon, B.-S., & Ganiel, U. (2000). From fragmented knowledge to a knowledge structure: Linking the domains of mechanics and electromagnetism. American Journal of Physics , 68 (S1), S16–S26.
Bailin, S. (1996). Critical thinking. In J. J. Chambliss (Ed.), Philosophy of education: An encyclopedia , (vol. 1671, pp. 119–123). Routledge.
Bangert-Drowns, R. L., & Bankert, E. (1990). Meta-analysis of effects of explicit instruction for critical thinking. Research report. ERIC Number: ED328614.
Google Scholar
Bao, L., Cai, T., Koenig, K., Fang, K., Han, J., Wang, J., … Wu, N. (2009). Learning and scientific reasoning. Science , 323 , 586–587. https://doi.org/10.1126/science.1167740 .
Bao, L., & Redish, E. F. (2001). Concentration analysis: A quantitative assessment of student states. American Journal of Physics , 69 (S1), S45–S53.
Bao, L., & Redish, E. F. (2006). Model analysis: Representing and assessing the dynamics of student learning. Physical Review Special Topics-Physics Education Research , 2 (1), 010103.
Bao, L., Xiao, Y., Koenig, K., & Han, J. (2018). Validity evaluation of the Lawson classroom test of scientific reasoning. Physical Review Physics Education Research , 14 (2), 020106.
Beichner, R. J., Saul, J. M., Abbott, D. S., Morse, J. J., Deardorff, D., Allain, R. J., … Risley, J. S. (2007). The student-centered activities for large enrollment undergraduate programs (SCALE-UP) project. Research-Based Reform of University Physics , 1 (1), 2–39.
Binkley, M., Erstad, O., Herman, J., Raizen, S., Ripley, M., & Rumble, M. (2010). Draft White paper defining 21st century skills . Melbourne: ACTS.
Bloom, B. S., Engelhart, M. D., Furst, E. J., Hill, W. H., & Krathwohl, D. R. (1956). Taxonomy of educational objectives: Handbook 1: Cognitive domain . New York: Longman.
Bransford, J. D., Brown, A. L., & Cocking, R. R. (2000). How people learn , (vol. 11). Washington, DC: National Academy Press.
Brown, A. (1989). Analogical learning and transfer: What develops? In S. Vosniadu, & A. Ortony (Eds.), Similarity and analogical reasoning , (pp. 369–412). New York: Cambridge U.P.
Chapter Google Scholar
Cavallo, A. M. L., Rozman, M., Blickenstaff, J., & Walker, N. (2003). Learning, reasoning, motivation, and epistemological beliefs: Differing approaches in college science courses. Journal of College Science Teaching , 33 (3), 18–22.
Chen, Z., & Klahr, D. (1999). All other things being equal: Acquisition and transfer of the control of variables strategy. Child Development , 70 , 1098–1120.
Chi, M. T., Bassok, M., Lewis, M. W., Reimann, P., & Glaser, R. (1989). Self-explanations: How students study and use examples in learning to solve problems. Cognitive Science , 13 (2), 145–182.
Chi, M. T., Feltovich, P. J., & Glaser, R. (1981). Categorization and representation of physics problems by experts and novices. Cognitive Science , 5 (2), 121–152.
Chi, M. T., & Slotta, J. D. (1993). The ontological coherence of intuitive physics. Cognition and Instruction , 10 (2–3), 249–260.
Chi, M. T., Slotta, J. D., & De Leeuw, N. (1994). From things to processes: A theory of conceptual change for learning science concepts. Learning and Instruction , 4 (1), 27–43.
Chi, M. T. H. (1992). Conceptual change within and across ontological categories: Examples from learning and discovery in science. In R. N. Giere (Ed.), Cognitive models of science . Minneapolis: University of Minnesota Press.
Chiu, M. H. (2001). Algorithmic problem solving and conceptual understanding of chemistry by students at a local high school in Taiwan. Proceedings-National Science Council Republic of China Part D Mathematics Science and Technology Education , 11 (1), 20–38.
Chiu, M.-H., Guo, C. J., & Treagust, D. F. (2007). Assessing students’ conceptual understanding in science: An introduction about a national project in Taiwan. International Journal of Science Education , 29 (4), 379–390.
Clement, J. (1982). Students’ preconceptions in introductory mechanics. American Journal of Physics , 50 (1), 66–71.
Coletta, V. P., & Phillips, J. A. (2005). Interpreting FCI scores: Normalized gain, preinstruction scores, and scientific reasoning ability. American Journal of Physics , 73 (12), 1172–1182.
Cracolice, M. S., Deming, J. C., & Ehlert, B. (2008). Concept learning versus problem solving: A cognitive difference. Journal of Chemical Education , 85 (6), 873.
De Jong, T., & Ferguson-Hesler, M. G. M. (1986). Cognitive structure of good and poor problem solvers in physics. Journal of Educational Psychology , 78 , 279–288.
DiSessa, A. A. (1993). Toward an epistemology of physics. Cognition and Instruction , 10 (2–3), 105–225.
Duit, R., & Treagust, D. F. (2003). Conceptual change: A powerful framework for improving science teaching and learning. International Journal of Science Education , 25 (6), 671–688.
Dykstra Jr., D. I., Boyle, C. F., & Monarch, I. A. (1992). Studying conceptual change in learning physics. Science Education , 76 (6), 615–652.
Ennis, R. (1993). Critical thinking assessment. Theory Into Practice , 32 (3), 179–186.
Etkina, E., & Van Heuvelen, A. (2001). Investigative science learning environment: Using the processes of science and cognitive strategies to learn physics. In Proceedings of the 2001 physics education research conference , (pp. 17–21). Rochester.
Etkina, E., Van Heuvelen, A., White-Brahmia, S., Brookes, D. T., Gentile, M., Murthy, S., … Warren, A. (2006). Scientific abilities and their assessment. Physical Review Special Topics-Physics Education Research , 2 (2), 020103.
Eylon, B.-S., & Reif, F. (1984). Effects of knowledge organization on task performance. Cognition and Instruction , 1 (1), 5–44.
Fabby, C., & Koenig, K. (2013). Relationship of scientific reasoning to solving different physics problem types. In Proceedings of the 2013 Physics Education Research Conference, Portland, OR .
Facione, P. A. (1990). Critical thinking: A statement of expert consensus for purposes of educational assessment and instruction – The Delphi report . Millbrae: California Academic Press.
Ferguson-Hesler, M. G. M., & De Jong, T. (1990). Studying physics texts: Differences in study processes between good and poor solvers. Cognition and Instruction , 7 (1), 41–54.
Fisher, A. (2001). Critical thinking: An introduction . Cambridge: Cambridge University Press.
Freeman, S., Eddy, S. L., McDonough, M., Smith, M. K., Okoroafor, N., Jordt, H., & Wenderoth, M. P. (2014). Active learning increases student performance in science, engineering, and mathematics. Proceedings of the National Academy of Sciences , 111 (23), 8410–8415.
Glaser, E. M. (1941). An experiment in the development of critical thinking . New York: Teachers College, Columbia University.
Hake, R. R. (1992). Socratic pedagogy in the introductory physics laboratory. The Physics Teacher , 30 , 546.
Hake, R. R. (1998). Interactive-engagement versus traditional methods: A six-thousand-student survey of mechanics test data for introductory physics courses. American Journal of Physics , 66 (1), 64–74.
Halloun, I. A., & Hestenes, D. (1985a). The initial knowledge state of college physics students. American Journal of Physics , 53 (11), 1043–1055.
Halloun, I. A., & Hestenes, D. (1985b). Common sense concepts about motion. American Journal of Physics , 53 (11), 1056–1065.
Halpern, D. F. (1999). Teaching for critical thinking: Helping college students develop the skills and dispositions of a critical thinker. New Directions for Teaching and Learning , 80 , 69–74. https://doi.org/10.1002/tl.8005 .
Hardiman, P. T., Dufresne, R., & Mestre, J. P. (1989). The relation between problem categorization and problem solving among experts and novices. Memory & Cognition , 17 (5), 627–638.
Heller, J. I., & Reif, F. (1984). Prescribing effective human problem-solving processes: Problem description in physics. Cognition and Instruction , 1 (2), 177–216.
Hoellwarth, C., Moelter, M. J., & Knight, R. D. (2005). A direct comparison of conceptual learning and problem solving ability in traditional and studio style classrooms. American Journal of Physics , 73 (5), 459–462.
Hofstein, A., & Lunetta, V. N. (1982). The role of the laboratory in science teaching: Neglected aspects of research. Review of Educational Research , 52 (2), 201–217.
Hsu, L., Brewe, E., Foster, T. M., & Harper, K. A. (2004). Resource letter RPS-1: Research in problem solving. American Journal of Physics , 72 (9), 1147–1156.
Jensen, J. L., & Lawson, A. (2011). Effects of collaborative group composition and inquiry instruction on reasoning gains and achievement in undergraduate biology. CBE - Life Sciences Education , 10 , 64–73.
Johnson, M. A., & Lawson, A. E. (1998). What are the relative effects of reasoning ability and prior knowledge on biology achievement in expository and inquiry classes? Journal of Research in Science Teaching , 35 (1), 89–103.
Kalman, C., & Lattery, M. (2018). Three active learning strategies to address mixed student epistemologies and promote conceptual change. Frontiers in ICT , 5 (19), 1–9.
Karplus, R. (1964). The science curriculum improvement study. Journal of College Science Teaching , 2 (4), 293–303.
Kim, E., & Pak, S.-J. (2002). Students do not overcome conceptual difficulties after solving 1000 traditional problems. American Journal of Physics , 70 (7), 759–765.
Koenig, K., Schen, M., & Bao, L. (2012). Explicitly targeting pre-service teacher scientific reasoning abilities and understanding of nature of science through an introductory science course. Science Educator , 21 (2), 1–9.
Koenig, K., Schen, M., Edwards, M., & Bao, L. (2012). Addressing STEM retention through a scientific thought and methods course. Journal of College Science Teaching , 41 , 23–29.
Koenig, K., Wood, K., Bortner, L., & Bao, L. (2019). Modifying traditional labs to target scientific reasoning. Journal of College Science Teaching , 48 (5), 28-35.
Kozminski, J., Beverly, N., Deardorff, D., Dietz, R., Eblen-Zayas, M., Hobbs, R., … Zwickl, B. (2014). AAPT recommendations for the undergraduate physics laboratory curriculum , (pp. 1–29). American Association of Physics Teachers Retrieved from https://www.aapt.org/Resources/upload/LabGuidlinesDocument_EBendorsed_nov10.pdf .
Kuhn, D. (1992). Thinking as argument. Harvard Educational Review , 62 (2), 155–178.
Larkin, J., McDermott, J., Simon, D. P., & Simon, H. A. (1980). Expert and novice performance in solving physics problems. Science , 208 (4450), 1335–1342.
Laws, P. W. (2004). Workshop physics activity guide, module 4: Electricity and magnetism. In Workshop physics activity guide . Wiley-VCH.
Lawson, A. E. (1978), The development and validation of a classroom test of formal reasoning, Journal of Research in Science Teaching , 15 (1), 11–24.
Lawson, A. E. (1992). The development of reasoning among college biology students - a review of research. Journal of College Science Teaching , 21 , 338–344.
Lawson, A. E. (2000). Classroom test of scientific reasoning: Multiple choice version, based on Lawson, A. E. 1978. Development and validation of the classroom test of formal reasoning. Journal of Research in Science Teaching , 15 (1), 11–24.
Lee, H. S., Liu, O. L., & Linn, M. C. (2011). Validating measurement of knowledge integration in science using multiple-choice and explanation items. Applied Measurement in Education , 24 (2), 115–136.
Linn, M. C. (2005). The knowledge integration perspective on learning and instruction. In R. K. Sawyer (Ed.), The Cambridge handbook of the learning sciences , (pp. 243–264). Cambridge: Cambridge University Press. https://doi.org/10.1017/CBO9780511816833.016 .
Lipman, M. (2003). Thinking in education , (2nd ed., ). Cambridge: Cambridge University Press.
Liu, X. (2010). Science and engineering education sources. Using and developing measurement instruments in science education: A Rasch modeling approach . Charlotte: IAP Information Age Publishing.
Marzano, R. J., Brandt, R. S., Hughes, C. S., Jones, B. F., Presseisen, B. Z., Rankin, S. C., et al. (1988). Dimensions of thinking, a framework for curriculum and instruction . Alexandria: Association for Supervision and Curriculum Development.
Mazur, E. (1997). Peer instruction: A user’s manual . Upper Saddle River: Prentice Hall.
McDermott, L. C. (1996). Physics by Inquiry: An Introduction to the Physical Sciences . John Wiley & Sons, New York, NY.
McDermott, L. C., & Redish, E. F. (1999). Resource letter: PER-1: Physics education research. American Journal of Physics , 67 (9), 755–767.
McKeachie, W. J. (1986). Teaching and learning in the college classroom: A review of the research literature . Ann Arbor: National Center for Research to Improve Postsecondary Teaching and Learning.
Meltzer, D. E., & Otero, V. K. (2015). A brief history of physics education in the United States. American Journal of Physics , 83 (5), 447–458.
Meltzer, D. E., & Thornton, R. K. (2012). Resource letter ALIP-1: Active-learning instruction in physics. American Journal of Physics , 80 (6), 478–496.
Minstrell, J. (1992). Facets of students’ knowledge and relevant instruction. In R. Duit, F. Goldberg, & H. Niedderer (Eds.), Proceedings of the international workshop: Research in physics learning- theoretical issues and empirical studies , (pp. 110–128). The Institute for Science Education.
Nakhleh, M. B. (1993). Are our students conceptual thinkers or algorithmic problem solvers? Identifying conceptual students in general chemistry. Journal of Chemical Education , 70 (1), 52. https://doi.org/10.1021/ed070p52 .
Nakhleh, M. B., & Mitchell, R. C. (1993). Concept learning versus problem solving: There is a difference. Journal of Chemical Education , 70 (3), 190. https://doi.org/10.1021/ed070p190 .
National Research Council (2011). Assessing 21st century skills: Summary of a workshop . Washington, DC: The National Academies Press. https://doi.org/10.17226/13215 .
Book Google Scholar
National Research Council (2012a). Education for life and work: Developing transferable knowledge and skills in the 21st century . Washington, DC: The National Academies Press.
National Research Council (2012b). A framework for K-12 science education: Practices, crosscutting concepts, and core ideas . Washington, DC: National Academies Press.
National Research Council (2012c). Discipline-based education research: Understanding and improving learning in undergraduate science and engineering . Washington, DC: National Academies Press.
National Science & Technology Council (2018). Charting a course for success: America’s strategy for STEM education . Washington, DC: Office of Science and Technology Policy.
NCET. (1987). Critical thinking as defined by the National Council for excellence in critical thinking, statement by Michael Scriven & Richard Paul, presented at the 8th annual conference on critical thinking and education reform. Retrieved December 4, 2018, from http://www.criticalthinking.org/pages/defining-critical-thinking/766 .
Nordine, J., Krajcik, J., & Fortus, D. (2011). Transforming energy instruction in middle school to support integrated understanding and future learning. Science Education , 95 (4), 670–699.
Nurrenbern, S. C., & Pickering, M. (1987). Concept learning versus problem solving: Is there a difference? Journal of Chemical Education , 64 (6), 508.
Paul, R. (1990). Critical thinking: What every person needs to survive in a rapidly changing world . Rohnert Park: Center for Critical Thinking and Moral Critique.
Perkins, D. N., & Salomon, G. (1989). Are cognitive skills context-bound? Educational Researcher , 18 (1), 16–25.
Posner, G., Strike, K., Hewson, P., & Gertzog, W. (1982). Accommodation of a scientific conception: Toward a theory of conceptual change. Science Education , 66 (2), 211–227.
Reay, N. W., Bao, L., Li, P., Warnakulasooriya, R., & Baugh, G. (2005). Toward the effective use of voting machines in physics lectures. American Journal of Physics , 73 (6), 554–558.
Reimers, F. M., & Chung, C. K. (Eds.) (2016). Teaching and learning for the twenty-first century: Educational goals, policies and curricula from six nations . Cambridge: Harvard Education Press.
Salomon, G., & Perkins, D. N. (1989). Rocky roads to transfer: Rethinking mechanism of a neglected phenomenon. Educational Psychologist , 24 (2), 113–142.
Schoenfeld, A. H., & Herrmann, D. J. (1982). Problem perception and knowledge structure in expert and novice mathematical problem solvers. Journal of Experimental Psychology: Learning, Memory, and Cognition , 8 (5), 484.
Shaw, V. F. (1996). The cognitive processes in informal reasoning. Thinking and Reasoning , 2 (1), 51–80.
She, H., & Liao, Y. (2010). Bridging scientific reasoning and conceptual change through adaptive web-based learning. Journal of Research in Science Teaching , 47 (1), 91–119.
Shen, J., Liu, O. L., & Chang, H.-Y. (2017). Assessing students’ deep conceptual understanding in physical sciences: An example on sinking and floating. International Journal of Science and Mathematics Education , 15 (1), 57–70. https://doi.org/10.1007/s10763-015-9680-z .
Siegel, H. (1988). Educating reason: Rationality, critical thinking and education , (vol. 1). New York: Routledge.
Slotta, J. D., Chi, M. T., & Joram, E. (1995). Assessing students’ misclassifications of physics concepts: An ontological basis for conceptual change. Cognition and Instruction , 13 (3), 373–400.
Smith III, J. P., DiSessa, A. A., & Roschelle, J. (1994). Misconceptions reconceived: A constructivist analysis of knowledge in transition. The Journal of the Learning Sciences , 3 (2), 115–163.
Smith, M. U. (1992). Expertise and organization of knowledge: Unexpected differences among genetic counselors, faculty members and students on problem categorization tasks. Journal of Research in Science Teaching , 29 (2), 179–205.
Sobhanzadeh, M., Kalman, C. S., & Thompson, R. I. (2017). Labatorials in introductory physics courses. European Journal of Physics , 38 , 1–18.
Sokoloff, D. R., Thornton, R. K., & Laws, P. W. (2011). RealTime physics: Active learning laboratories . New York: Wiley.
Stamovlasis, D., Tsaparlis, G., Kamilatos, C., Papaoikonomou, D., & Zarotiadou, E. (2005). Conceptual understanding versus algorithmic problem solving: Further evidence from a national chemistry examination. Chemistry Education Research and Practice , 6 (2), 104–118.
Tanenbaum, C. (2016). STEM 2026: A vision for innovation in STEM education . Washington, DC: US Department of Education.
Thornton, R. K., & Sokoloff, D. R. (1998). Assessing student learning of Newton’s laws: The force and motion conceptual evaluation and the evaluation of active learning laboratory and lecture curricula. American Journal of Physics , 66 (4), 338–352.
Trowbridge, L. W., Bybee, R. W., & Powell, J. C. (2000). Teaching secondary school science: Strategies for developing scientific literacy . Upper Saddle River: Merrill-Prentice Hall.
United States Chamber of Commerce (2017). Bridging the soft skills gap: How the business and education sectors are partnering to prepare students for the 21 st century workforce . Washington DC: Center for Education and Workforce, U.S. Chamber of Commerce Foundation.
Veldhuis, G. H. (1990). The use of cluster analysis in categorization of physics problems. Science Education , 74 (1), 105–118.
Vosniadou, S., Vamvakoussi, X., & Skopeliti, I. (2008). The framework theory approach to the problem of conceptual change. In S. Vosniadou (Ed.), International handbook of research on conceptual change . New York: Routledge.
Wexler, P. (1982). Structure, text, and subject: A critical sociology of school knowledge. In M. W. Apple (Ed.), Cultural and economic reproduction in education: Essays on class, ideology and the state . London: Routledge & Regan Paul.
Zeineddin, A., & Abd-El-Khalick, F. (2010). Scientific reasoning and epistemological commitments: Coordination of theory and evidence among college science students. Journal of Research in Science Teaching , 47 (9), 1064–1093.
Zimmerman, C. (2000). The development of scientific reasoning skills. Developmental Review , 20 (1), 99–149.
Download references
Acknowledgements
The research is supported in part by NSF Awards DUE-1431908 and DUE-1712238. Any opinions, findings, and conclusions or recommendations expressed in this paper are those of the author(s) and do not necessarily reflect the views of the National Science Foundation.
The research is supported in part by NSF Awards DUE-1431908 and DUE-1712238.
Author information
Authors and affiliations.
The Ohio State University, Columbus, OH, 43210, USA
University of Cincinnati, Cincinnati, OH, 45221, USA
Kathleen Koenig
You can also search for this author in PubMed Google Scholar
Contributions
LB developed the concept, wrote a significant portion of the review and position, and synthesized the paper. KK wrote and edited a significant portion of the paper. Both authors read and approved the final manuscript.
Corresponding author
Correspondence to Lei Bao .
Ethics declarations
Competing interests.
The authors declare that they have no competing interests.
Additional information
Publisher’s note.
Springer Nature remains neutral with regard to jurisdictional claims in published maps and institutional affiliations.
Rights and permissions
Open Access This article is distributed under the terms of the Creative Commons Attribution 4.0 International License ( http://creativecommons.org/licenses/by/4.0/ ), which permits unrestricted use, distribution, and reproduction in any medium, provided you give appropriate credit to the original author(s) and the source, provide a link to the Creative Commons license, and indicate if changes were made.
Reprints and permissions
About this article
Cite this article.
Bao, L., Koenig, K. Physics education research for 21 st century learning. Discip Interdscip Sci Educ Res 1 , 2 (2019). https://doi.org/10.1186/s43031-019-0007-8
Download citation
Received : 17 April 2019
Accepted : 13 June 2019
Published : 28 November 2019
DOI : https://doi.org/10.1186/s43031-019-0007-8
Share this article
Anyone you share the following link with will be able to read this content:
Sorry, a shareable link is not currently available for this article.
Provided by the Springer Nature SharedIt content-sharing initiative
- Twenty-first century learning
- STEM education
- Scientific reasoning
- Deep learning
- Our Mission
Deeper Learning: Defining Twenty-First Century Literacy
Only a decade and a few years in, how can we fully describe the twenty-first century learner? So far, this we do know: She is a problem solver, critical thinker, and an effective collaborator and communicator. We also know that a deeper learning environment is required in order to nurture and grow such a learner.
Watch a high school student talk about developing 21st-century skills through deeper learning.
How about that last characteristic of a 21st-century learner, effective communicator? Being literate means one who is advanced at reading, writing, speaking, and listening. And, in all schools -- deeper learning driven or not -- literacy is a curriculum fundamental. Its importance President Obama explained in a speech a few years back:
In today's world, being literate requires much, much more than the traditional literacy of yesterday. According to the NCTE (National Council of Teachers of English), twenty-first century readers and writers need to:
- Gain proficiency with tools of technology
- Develop relationships with others and confront and solve problems collaboratively and cross-culturally
- Design and share information for global communities to meet a variety of purposes
- Manage, analyze, and synthesize multiple streams of simultaneous information
- Create, critique, analyze, and evaluate multimedia texts
- Attend to the ethical responsibilities required by these complex environments
In the Classroom
When it comes to effective communicator, we can no longer consider college and career ready as simply sending students out as good readers and writers. As the definition of literacy evolves in our world, so does the charge of schools in helping students work towards and gain mastery of the skills and abilities NCTE has outlined above. Let's take a look at some of those:
Gain proficiency with tools of technology. The greatest tech tool of all? The Internet. It undoubtedly gives access to an unending wealth of information and has revolutionized our lives. I remember years ago trying to fix my lawnmower. I went to three public libraries trying to find a lawnmower manual that was close enough to my machine to be useful. Now? Go to YouTube and someone will take you step-by-step through repairing or making just about anything and everything.
But for all the amazing, valuable stuff on the Web, there's loads of misinformation, half-truths, and misquoted, useless stuff. In preparing children to be literate in today's world, it's urgent that we teach the skill of scoping websites with a critical eye. Kids need to be explicitly trained in spotting everything from blatant pseudo-facts to slightly questionable content. This ability to "filter" will be required of them in both university and work.
Create, critique, analyze, and evaluate multimedia texts and manage, analyze, and synthesize multiple streams of simultaneous information. The term multimedia, which means using more than one medium of expression or creation to communicate, encompasses a great deal more than it did 10, or even five years ago. To convey their deeper learning of a new concept, I remember having students include with their written analysis a printed graph, chart or image, and later, I required they add some audio or video element or links from websites. Now, students are creating websites as the task itself. A flat, inanimate thing like a written report or essay -- or a book or newspaper, for that matter -- is no longer the paradigm of communication.
Communication today is like a living thing, morphing continuously -- a hybrid of script, interactive, audio, and moving images. What does this mean for teachers and students? Schools need to be preparing students to masterfully navigate, judge, and create this type of sophisticated communication -- and do so quickly and efficiently.
What's Next?
It's true that, through deeper learning, many teachers and schools are already guiding students into this new century well-equipped to think critically on their feet, problem solve, and communicate effectively. But as we enter our thirteenth year, we need to stay mindful that the definition for 21st century learner -- and literacy -- will continue to change.
Want to create or adapt books like this? Learn more about how Pressbooks supports open publishing practices.
13 21st-Century Teachers and Learners – Meeting the Needs of All Learners
‘If a child can’t learn the way we teach, maybe we should teach the way they learn.” –Ignacio Estrada
Essential Questions
- What is the central goal of 21st-century learning?
- How are the skills of the 21st-century teacher different than those in times past?
- Why is it important for teachers to consider universal design when designing curriculum or lessons for all students?
- How can a teacher’s relationship with a student have a positive impact on self-concept and the future?

Introduction
There is now greater diversity than in times past, and a “one-size-fits-all” classroom is no longer appropriate. In the past, the emphasis was on the “3R’s” (reading, writing, arithmetic) as well as social studies, science and language. The model was teacher-centered with an emphasis on teaching strategies that focused on repetition, memorization, and lecturing; and tests were given at the end of the learning cycle to assess student learning. Today, curriculum developers realize the importance of developing educational goals and teaching methods that prepare students for college and future careers (Alismail & McGuire, 2015).
The Educational Landscape in the 21st Century
The term 21st-century skills encompasses a broad set of knowledge and skills that are not easy to define because the use of the term is often inconsistent, but they are generally considered to be those outlined in Figure 13.1.
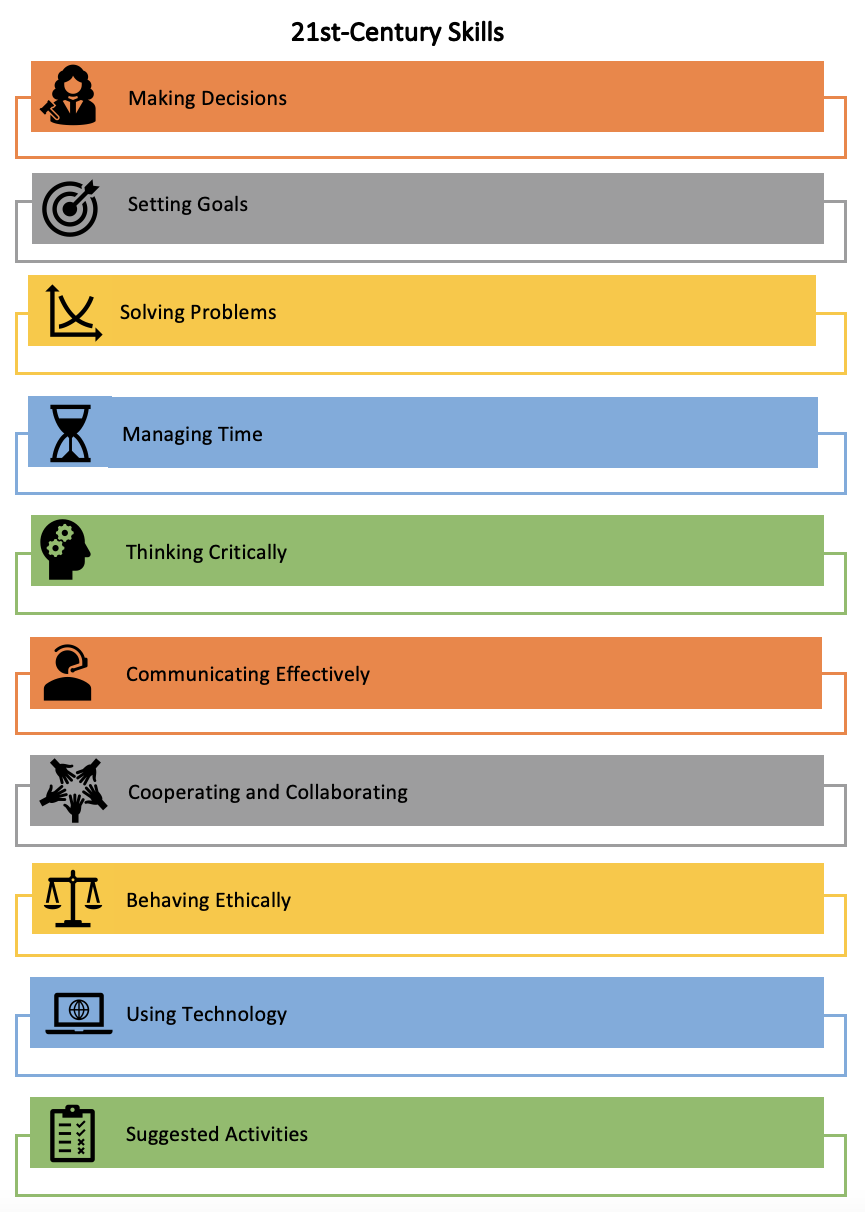
21st-Century-Skills-Graphic
The 21st-century skills were developed because it is often thought that students in this century need a wide variety of skills in addition to the academic standards that have been adopted in many states. The 21st-century skills ideally work in tandem with academic or content standards and can be taught in or out of school. They also lend themselves well to an integrated curriculum, project-based learning, and authentic learning experiences. For more details about the skills, access the link above.
The Common Core State Standards (CCSS) and Partnership for 21st Century Skills integrated the framework prepared by The Partnership for 21st Century Skills. This plan advocated for the integration of core academic knowledge, critical thinking, and social skills in teaching and learning to support students in mastering the multi-dimensional abilities required in the 21st century. These skills include the “ New 3Rs ” (Relationships, Routines, and Resilience) of core academic content mastery (Cantor, 2021) and the 4Cs of Critical Thinking, Communication, Collaboration, and Creativity (Stauffer, 2021). By integrating cognitive learning and skills into the curriculum, students can gain a deeper understanding of the subject as well as ways to solve complex problems in the real world.
The Partnership for 21st Century Skills prepared educational standards for the next generation to present an appropriate strategy to apply them. The 21st-century standards:
- focus on 21st-century skills, content knowledge, and expertise
- build understanding across and among core subject areas as well as 21st-century interdisciplinary themes
- emphasize deep understanding rather than shallow knowledge.
- engage students with real-world data, tools, and experts they will encounter in college, on the job, and in life
- allow for multiple measures of mastery (Alismail & McGuire, 2015)
By adopting a 21st-century curriculum, there can be a blend of knowledge, thinking, innovation skills, media, literacy, information, and communication technology coupled with real-life experiences and authentic learning that are integrated into the academic subjects (Lombardi, 2007). The central goal for curriculum in the 21st century is a focus on the construction of knowledge that encourages students to create information that has value for them and helps them gain new skills. Developing curriculum that is based in the real world also encourages student participation and supports them in understanding the knowledge rooted in the core subjects. Additionally, this will provide students with the opportunity to develop civic, financial, environmental, and health literacies as well as global awareness.
Curriculum that emphasizes the construction of knowledge and is rooted in the core subjects is the starting point. The question becomes: how do we reach all the learners with diverse needs?
The 21st-Century Teacher
To meet the needs of learners, teachers should possess additional skills, including those of technology. Palmer (2015) describes 15 Characteristics of a 21st-Century Teacher that include: a learner-centered classroom, students as learners, users, and producers of digital content, and project-based learning. A more complete description of these skills can be found in the article: 15 Characteristics of a 21st-Century Teacher .
Universal Design for Learning
Universal Design for Learning (UDL) re-conceptualizes curriculum design by placing student diversity at the forefront and designing flexible and accommodating curriculums to meet the needs of diverse students (Strangeman, et. al, 2020). This is a relatively new approach to curriculum that is grounded in the belief that each learner is unique and brings different strengths and areas of weaknesses to the classroom (Rose and Meyer, 2002). In classrooms today, students come from diverse backgrounds, cultures, socioeconomic, and disability groups. Many traditional curriculums are designed to meet the needs of a “typical” or “average” student. This can be a significant disadvantage for students not in these categories and can lead to barriers that make access and progress more difficult.
UDL is based on the same principles as universal design in architecture which began as a movement to design structures with all users in mind and includes features like ramps and elevators to make access easier (Connell, et al., 1997). Not only does the design allow access for students with disabilities, but an unexpected side effect of this process is that it provided accessibility for all individuals, therefore, usability benefitted more people. The next step was to apply UDL to curriculum by considering the needs of all students, beginning at the planning stage. By maximizing access to information, it provided additional access to learning (Rose & Meyer, 2002).
Application of UDL during the Curriculum Design Process
Neuroscience has also contributed to the guidance of UDL in curriculum design. Research in neuroscience includes three broad neural networks in the brain that oversee three avenues of learning: UDL classifies these three avenues as recognition, strategic, and affective networks (Cytowic, 1996; Luria, 1973; Rose & Meyer, 2002). UDL stresses that these three abilities differ from student to student. As a way to meet this challenge there are three UDL principles that guide a flexible curriculum design process:
- to support recognition learning, provide multiple, flexible methods of presentation,
- to support strategic learning, provide multiple, flexible methods of expression and apprenticeship, and
- to support effective learning, provide multiple, flexible options for engagement (Rose & Meyer, 2002).
The three principles can be included in the design of goals, the inclusion of strategies and resources, flexible presentations and assessments. Using an assessment as an example, curriculum designers can include a range of media, formats, and response options so that the student’s knowledge and skills are assessed and not their ability to cope with the format and presentation. This is true for both formative and summative assessments.
Multimedia tools and peer reviews provide feedback to students that can improve their work and increase team collaboration. Technology also has the advantage of allowing students to gain information and knowledge as well as the development of different literacies even if they are working by themselves. This can encourage students to pursue individual passions for learning about specific topics that support creative thinking and innovation skills.
21st-Century Students as Consumers of Information
According to Carol Ann Tomlinson, 21st-century schools must prepare students to be wise consumers of information, and confident producers of knowledge. The 21st-century student populations are more heterogeneous than in the past, which means schools need to become more responsive to diverse cultures, languages, experiences, economics, and interests—and do this in ways that provide equity of access to dynamic learning experiences to meet the needs of all learners.
Current research suggests that the best vision of a 21st-century classroom is centered on the learners, knowledge, assessment, instruction, and the classroom community (National Research Council, 2000). Technology, when used effectively, can support teachers and students in a variety of ways, including curriculum planning, differentiated learning opportunities, assessment development and meeting the needs of a diverse student population.
There is agreement among educators and the public that we must establish certain “core skills” that should be included in this framework (Binkley, et al, 2012). The challenge is that there is no single framework for what is included in the list of skills. The Partnership for 21st Century Skills framework (Dede, 2010) has two categories of skill groups. The first is “perennial” skills, or those retained from the 20th century, but are still valuable in the 21st century. These skills include communication, creativity, and critical thinking. The second category is “contextual skills,” or those unique to the 21st century that includes the ability to manage large quantities of digital information and data that are important for decision-making.
The Metiri Group and the North Central Regional Education Laboratory (NCREL) developed the 21st Century Skills Framework (Burkhardt, et al. 2003 ), with the following four, key categories of skills (refer to the P21 Framework Definitions for a full description of these skills):
- digital age literacies,
- inventive thinking,
- effective communication, and
- high productivity.
It seems that the needs of the 21st-century classroom are different than they were in the past. One key difference is that teachers are facilitators of learning, and it is their responsibility to have a curriculum that supports students in developing skills for an academic program and eventually the workplace. Another key difference is the emphasis on a project-based curriculum that encourages higher-order thinking skills, effective communication, and technology skills. One common thread has become the need for successful collaboration as a part of student learning. To accomplish these things, it is important to move beyond the skills of the 20th century and master those of the 21st century. The 21st Century Skill: Rethinking How Students Learn discusses some strategies for doing just that.
“Teaching Up” and the 21st-Century Teacher
Another plan that is designed to maximize learning for all students in mixed-ability classrooms is “Teaching Up” which has been developed by Tomlinson and Javious (2012). In developing differentiated or responsive instruction, teachers begin by planning with a specific group of students in mind. They may also choose to plan from the academic standards using grade-level expectations and differentiate “up” or “down” from that point.
Other teachers may want to begin by developing learning experiences that focus on the essentials for students who have more difficulty with particular content to help clarify the essentials and to differentiate from that beginning position. “Teaching up” requires that the teachers begin by developing tasks that are a challenge for high performing learners, and then to differentiate or scaffold learning in ways that support a broad range of students in working with “advanced” levels of knowledge, understandings, and skills. “Teaching up” is rooted in six key principles as shown in Figure 13.2 (Tomlinson, 2015).
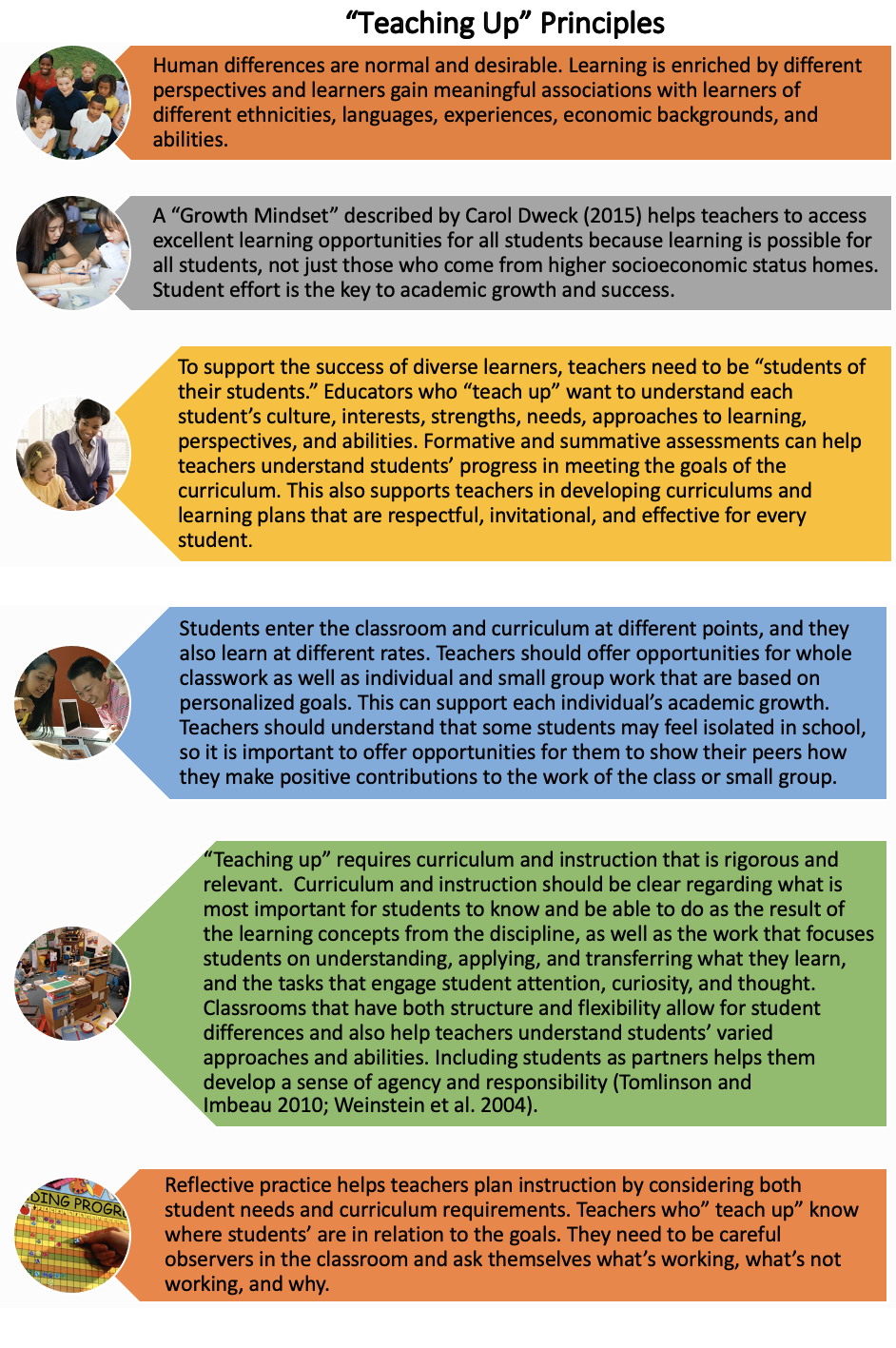
Teaching Up Principles
Developing Inclusive Practices in Literacy
Anita Nigam, author of the proceeding article, was born in India and taught elementary students there and in the U.S. She is currently a faculty member at a University in Illinois where she specializes in literacy and English language learning (ELL).
Classrooms now more than ever need to reflect the diverse cultures present as they provide a range of perspectives and experiences that can help shape the minds of all the students in mainstream classes. Previously, if there were students needing special attention, they were provided assistance outside the classroom; however, the focus now is to have the teacher meet the needs of all learners within the classroom, leading to the birth of inclusive classrooms. Therefore, the onus of developing culturally responsive classrooms falls on the shoulders of all classroom teachers. However, many teachers grew up without much exposure to cultural diversity and do not see the potential of all students nor the many issues and challenges that some students face (Sleeter, 2008). Teachers of color tend to recognize the literate potential of students of color and have high expectations for them but are not always aware of how to use students’ cultural knowledge to inform their teaching practices (Villegas & Davis, 2008).
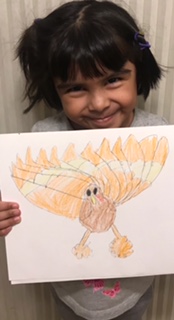
The summary of this qualitative research study is aimed at promoting awareness and implementation of developing culturally responsive literacy practices among preservice teachers who were part of the Elementary Education Program in a private university in the Midwest region of the United States. All 16 preservice teachers had a broad definition of diversity, i.e., the inclusion of students from special population which included ELLs and learners from diverse populations.
Throughout the semester the preservice teachers worked in an elementary school which was their clinical placement and also at an after-school program that offered tutoring services for students from grades one through five of the elementary school. Preliminary results and findings from the study show four major themes that emerged from the analysis of the data:
- creating differentiated assignments for students to do after they finish their work to meet the needs the different abilities that students have,
- pairing mainstream students with students from diverse backgrounds to talk about a family or a public event and encourage nurturing relationships and a welcoming environment,
- using the trading card strategy for vocabulary where students are given the choice to write the definition in their native language,
- reading “ First You, Then Together ” where the teacher would have students read a page or two to themselves before reading it together while having the students follow along with their fingers or reading aloud with the teacher while going over the same page, and
- using technology such as Chromebook to enable dictation so students are able to demonstrate their knowledge and writing abilities without having to jump through extra hurdles to complete the writing tasks required of them within the lesson.
These findings, though modest, are an attempt to create awareness of social equity literacy practices among preservice teachers teaching at a local elementary school with a diverse population.
A Place at the Table: Create a Community of Learners
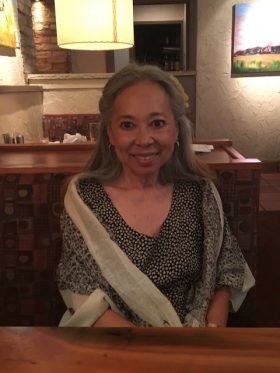
In the following article, A Place at the Table: Create a Community of Learners , Yvonne Siu-Runyan, a former classroom teacher, university professor, and past president of the National Council of Teachers of English, has summed up what is needed to help all learners gain literacy skills.
In order to learn, all students need to take risks. In order to take risks, all students need a safe place to learn and to be seen. That is, your students need to know that in your classroom, they each have a seat at the table and have voices and stories to share.
- Books and stories provide common experiences for students.
- Books and stories nurture an understanding of self and others.
- Books and stories shape our understanding of the world around us and ourselves.
- Listen to your students’ conversations in various settings . This simple action will provide you with useful information to guide your instruction and conversations with your students.
- Your best and most important ally are the parents/guardians.
- Never doubt the power of having a good relationship with parents/guardians.
- Thus, your first contact with parents/guardians should be a positive, not a negative one.
- Do call the parents/guardians of your students and make sure you have positive things to say? Most parents/guardians expect the worst.
- Before you end your conversation, be sure to tell the parent/guardian to share the positive information with the child.
- The next day watch — this child will stand taller, do more, and take more learning risks because this child will know that you care, and learning involves caring and LOVE.
- Students like it when their teachers read and write with them and share their reading and writing.
- By sharing your literacy experiences with students, they get an inside-out look at the power of reading and writing and you become a member of the literacy club in your classroom.
- Show the students your “inside out” process and make it visible.
- Students learn when their teachers model and show their inside out process.
- What do I do that helps you as a reader, writer, and thinker?
- What do I do that does not help you as a reader, writer, and thinker?
- When I asked these two questions of my students, I learned that my students liked it when I read and wrote alongside them for it made visible the processes involved in reading, writing, and thinking.
- Have a classroom library and showcase books. If we want our students to read, then it makes good sense that books must be accessible.
Books fall open; we fall in! I love this saying, “Ah … the power of the printed page.” Do create a classroom learning environment where students are surrounded by books. For if there are a wide variety of books in your classroom, your students will find books that speak to them. There is a plethora of stunning books written for young people like never before. Be sure to have books in which our young can see themselves and to which our students can relate. In this way, our young will be better equipped to reach back in order to reach out, and then reach out in order to reach forward with courage and love in our quest to make this place we call Earth a better place for all for: Education should liberate, not indoctrinate.
I end with this old Chinese Proverb: “Yin Zhen Zhi Ke,” or “Drinking poison to quench thirst.” This Chinese saying warns us that measures taken in the short term to solve a problem may be more damaging than the problem.
Be wary of narrow standards and standardized tests. Learning is much more.
Homeschooling
For those parents and students who want to differentiate based on individual needs, homeschooling is becoming one of the “go-to” alternatives.
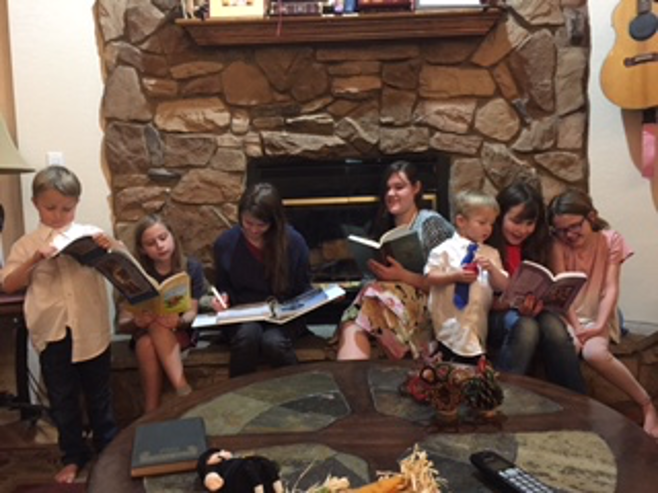
Home-based education led by families has been practiced by cultures around the world and down through history, and it has played an influential role in Western civilization (Gordon & Gordon). Homeschooling today is the parent-led practice of teaching their children rather than attending a public school (Ray, 2000 ).
During American Colonial times, children were taught at home using the Bible for reading. Writing, math, and vocational skills were usually taught by the father (Ray, 2017). The children of missionary families or families who traveled were taught using mail-order curricula that they could take to different parts of the world. Homeschooling at that time was often a joint effort between parents, tutors, older children, and families. At times, parents or groups would sometimes pool their resources to employ a teacher for more support (Hill, 2000 ). At the end of the 19th century, homeschooling was widely practiced, and public or formal school attendance was voluntary (Tyack, 1974 ).
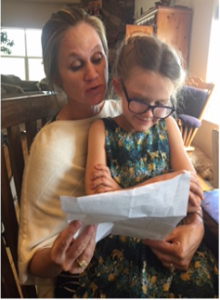
More than two million children today are homeschooled (Ray, 2017). The number of children in homeschools is now larger than the New York City public school system and even larger than the Los Angeles and Chicago public school systems combined (Hill, 2000 ; Ray, 2017). As a result, there is a rising need for support, collaboration, and professional expertise. Groups for home educators and networks are growing and becoming a new type of educational institution (Hill, 2000 ). They are usually identified as associations, co-ops, or charter schools (Collom, 2005 ; Hill, 200 0 ). Home school parents and supporters of this alternative form of education have developed new teaching models and curriculums to help parents become educators (Tilhou, 2020).
Common beliefs, motivations, and the choice to homeschool attract homeschool parents/educators who want mutual support and collaboration. These families do not want to be isolated, so they make use of services and resources from experts, and they form teams that gain others’ support, often through organized groups (Collom, 2005 ; Hill, 2000 ). The advantages of this collaborative effort can extend beyond families that collaborate with the support of public funds for materials and resources, facilities, and administrator’s time (Hill, 2000 ).
Collom ( 2005 ) found that the newest homeschoolers are highly motivated by academic reasons. The decision to home school can be categorized into four areas and include those who:
- are critical of public schools,
- attracted to home-based public charter,
- have ideological reasons, and
- focused on family and children’s needs.
Results also indicate that the home-based charter has been the most popular motivation, and criticism of public schools had a slightly lower score.
Homeschool Partnerships with Public Education and the Future
Thomas ( 2016 ) has stated that if home parents/educators see value in the resources in their community, public school parents may likely feel the same way. Additionally, if homeschooling parents make educational decisions based on the goal of providing high-quality learning experiences, teaching faith, setting student goals, and meeting family needs, public school parents may also have similar goals. Can public schools provide a variety of choices parents are wanting? Is it possible for public schools to provide more customized schedules that fit the changing interests and needs of public-school families? Thomas ( 2016 ) argues, after examining homeschool families’ instructional decisions and motivations, that public education ought to find more ways to listen to parents’ needs and understand the educational goals they have for their children.
By considering alternative educational models and parental motivations for forming and joining the homeschool movement, public education may gain valuable insights about meeting the needs of families in the 21st century (Tihou, 2020). There are currently school districts that engage in partnerships with homeschool educators. Partnerships allow homeschool students to access classes and programs while maintaining the respect that the child’s primary teacher is his or her parent (Dahlquist, et al. ( 2006 ). It may be advantageous for public schools to adopt inclusive policies that offer a range of partnership options with homeschool families as a way to improve communication and create more responsive public spaces where responsibilities for raising the next generation are shared (Thomas, 2016).
The prevalence of homeschool support groups and associations is growing across the United States. Members of these groups come from a variety of backgrounds, but all have a shared interest in providing quality education for their children. What has emerged from this like-mindedness is a movement of millions across the United States not only to educate one’s own child but to come together and form groups which lead to a greater impact on children’s learning and parents’ teaching. What is still unknown is the impact this movement may ultimately have.
Insight 13.0
Gone are the days when a teacher could lecture to a class for 30 minutes to an hour and keep their attention.
To be effective, teachers today must be knowledgeable about different content areas, as well as models and strategies for engaging all students in meaningful learning experiences. Parents make choices about their children’s education, and public schools are, in effect, competing with charter schools and homeschools for students.
Several excellent resources for meeting the challenges of 21st-century learning are available. For this ILA, view two or more of the preceding videos and post your biggest “takeaways” using in the ILA Response Group in Hypothesis.
Connecting to the 21st-Century Student
Cultural Diversity: Dr. Geneva Gay
Dr. Geneva Gay from the University of Washington, Seattle answers the question “Why is it important for faculty to employ culturally responsive teaching practices?”
The Power of a Teacher | Adam Saenz | TedEx Yale
Teachers and students in the 21st century must have broader and more varied skills than their counterparts of the past. Incorporating Universal Design as a part of curriculum planning can ease access to learning for many students. Some parents have chosen homeschooling as a way to meet the educational needs of their children. Carol Tomlinson and others find that differentiating instruction through “teaching up” can meet the needs of a diverse classroom.
Curriculum Essentials: A Journey Copyright © 2021 by Linda J. Button, Ed.D. is licensed under a Creative Commons Attribution-ShareAlike 4.0 International License , except where otherwise noted.
Share This Book
The Importance of Curiosity and Questions in 21st-Century Learning
- Share article
Editor’s Note: Today, Andrew P. Minigan, Education Project and Research Coordinator, The Right Question Institute, shares why he thinks the 4 Cs are missing a critical 5th C: Curiosity and Questioning.
In their Framework for 21 st Century Learning , P21 recognizes the importance of teachers developing their students’ learning and innovation skills. These skills, which are often referred to as “the 4 Cs,” stand for creativity, critical thinking, communication, and collaboration. The 4 Cs capture the skills students should hone during their formal education to complement and drive knowledge acquisition and content mastery. Yet, there is an additional C that may be just as fundamental for learning and innovation: curiosity and question formulation.
Asking questions is not simply a means to gather information. Rather, by asking questions, students can identify their own knowledge gaps and think critically about what they are learning, assess information from individuals and other sources of information, think creatively and divergently, and work constructively with others. Curiosity and the skill of formulating questions supports the 4 Cs while also tapping into a skill that is not highlighted in the Framework for 21 st Century Learning.
Curiosity and Question Formulation
It seems that fostering student curiosity can have a direct impact on student engagement, interest, and assist students in driving their learning . For instance, neuroscientists from the University of California at Davis found that high curiosity may improve individual’s memory for information they acquire, and they suggest “stimulating curiosity ahead of knowledge acquisition could enhance learning success.” And a meta-analysis of over 200 studies found that curiosity influences academic achievement and curiosity with conscientiousness has as much of an impact on achievement as intelligence.
If student curiosity is so beneficial to learning then why has it been so often overlooked in the classroom? In his book, A More Beautiful Question , Warren Berger mentions that product-driven schools are byproducts of the industrial age, but the modern-day entrepreneurial work environment necessitates a question-asking model of education.
There is a lot expected of classroom teachers as they juggle curricula and evolving standards, all while attempting to address the individual needs of their students. One way to pivot to a curiosity-infused learning environment is by deliberately teaching students how to formulate questions with simple strategies that support the work educators are already doing.
Teaching students how to ask and use their own questions can have a significant positive impact on students’ curiosity according to initial research findings from Boston University education researcher Shelby Clark. Students’ questions engage them in their own learning, can be useful for addressing teaching and learning objectives , and help students develop a skill that lends itself to innovation and original thinking.
In a piece for American Psychologist , Todd Kashdan and Frank Fincham suggest that “high curiosity is necessary, though not sufficient, for creativity.” They posit that curiosity may be a link between an individual’s experiences and an individual’s creativity skills. Thinking in questions can help students make meaningful connections between what they are learning in the classroom with their interests. Producing questions allows students to make these connections in an unlimited amount of ways and to think divergently—in many different directions.
Developing divergent thinking skills is essential for sparking creativity and is especially beneficial when followed sequentially by convergent thinking. The Question Formulation Technique (QFT) , for example, is a step-by-step process that helps students ask as many questions as they can and then transitions into students prioritizing their questions according to the educator’s instructions. This process honors the creativity and ingenuity of students while having them carefully consider which of their questions will be most relevant as the lesson progresses.
Allowing students to be curious can allow creativity to flow. As author Erik Wahl writes, “In our early years, you and I consistently embodied the key traits that drive constant creativity. Curiosity ruled our senses. Enthusiasm ignited our actions. We did not fear what we did not know—instead we thrived on the process of discovery.” Harnessing student curiosity can fuel productive divergent thinking while enhancing creative and innovative thinking. One student at Mt. San Antonio College just outside of Los Angeles learned the QFT in Professor Sun Ezzell’s class. The student realized that they were not only able to, “come up with questions very quickly” but also that their, “thinking and creativity is improving and growing” as a result.
Critical Thinking
Students can use questions to challenge their own assumptions and other students’ assumptions, consider what they know and what they do not know, and assess sources and information they are consuming. P21 highlights the importance of asking questions in their work on critical thinking and they believe that to solve problems students should be able to “identify and ask significant questions that clarify various points of view and lead to better solutions.”
Students who learn to ask better questions are able to recognize the importance of the process of asking questions for problem solving. An 11th grader in the Los Angeles Unified School District reflected that “questions can help focus on a problem and think of solutions.” Similarly, an undergraduate student at Brandeis University found that "[asking questions] helped me think more critically and deeply about the topics presented to us and about how to utilize different ideas to think about each one.” Not only do students think differently when they begin to ask questions, they also recognize the process of asking questions as the mechanism that allows them to think and problem solve.
Communication
Being able to ask questions is also an effective way to target and gather information from other individuals. Curiosity can lead students to consider what they do not know or are still wondering about, and questions are a way to communicate this lack of knowledge to target and gather information from others who may have the answer. Listening to other individuals’ questions can help one consider their perspective and learn from their thoughts. When students carefully listen to what their peers are curious about, it can stimulate their own curiosity and thinking.

An eighth grader at Gus Garcia Young Men’s Leadership Academy in Austin, Texas, captured the importance of articulating one’s own questions and hearing other students’ questions. The eighth grader said that by generating questions with other students, “it helps me by getting me to think about questions on my own. Also, it gets my mind in motion to think about the questions other people make.”
A kindergartner from Kentucky that participated in a question-asking activity with peers shared that “sometimes some stuff surprises me, like, about the questions. They surprise me that I haven’t thought of ... [what] someone else thought of that I didn’t know.” Carving out space for students to collaboratively ask questions contributes to more equitable classrooms where all students can play an active role in their education and appreciate that their peers have interesting questions to ask as well.
Collaboration
When students produce questions collaboratively, they become co-constructors in their inquiries. One student may shed light on an idea or concept through their questions that then sparks the thinking of another student, leading down interesting paths for learning.
Dr. Adyemi Stembridge , an educator known for his work in the field of equity and student engagement, recently recalled an instance where students in a 4th grade classroom in Cherry Creek School District in Colorado were grappling with a classroom discussion. One student had a suggestion for how to tackle this—use the Question Formulation Technique. After this suggestion, Dr. Stembridge asked the student why a QFT might help. The student quickly responded, “Because I have a lot of questions in my head and I’m sure a lot of us do.”
Through asking questions together, students gain new insight into their own thinking and the thinking of others and they learn to value the inquiry process. They build off one another’s questions to create new questions. Students can work together to reach a consensus on which questions they will seek answers to or explore further and as a team they can establish next steps for their inquiry.
Curiosity in the Age of Information
In the 21st century, there is an urgent need for students to be able craft their own questions, strategize on their inquiry, and harness their curiosity to drive their own learning. As Picasso once famously quipped, “computers are useless; they can only give you answers.” With the development of new technologies, there is no shortage of information to be found through a simple Google search.
This has placed a newfound value on the ability to think nimbly and to use curiosity to drive innovation by asking new questions that have yet to be explored. As journalist and author Clive Thompson put it, “How should you respond when you get powerful new tools for finding answers? Think of harder questions.” Curiosity and learning to ask better questions is fundamental to the development of the 5 Cs, for learning, and for life.
Connect with Andrew , RQI , and the Center for Global Education on Twitter.
Image credit: The Holler . Caption, “Sheila Varney’s kindergarten students at Southside Elementary School in Belfry, Kentucky eagerly asking questions during the Question Formulation Technique.”
The opinions expressed in Global Learning are strictly those of the author(s) and do not reflect the opinions or endorsement of Editorial Projects in Education, or any of its publications.
Sign Up for EdWeek Update
Edweek top school jobs.

Sign Up & Sign In

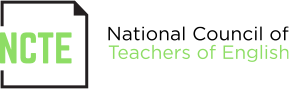
- Career Center
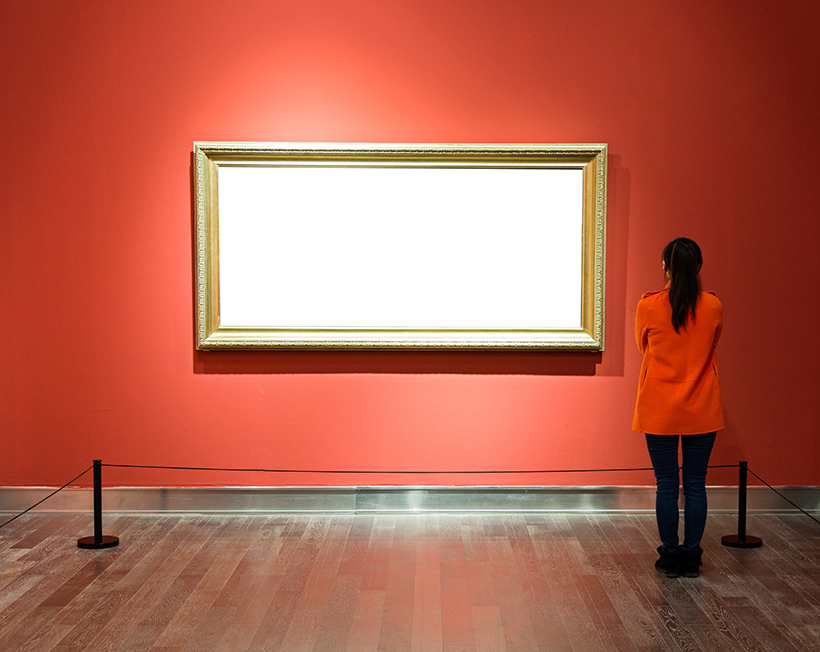
Visual Literacy Is Critical for 21st Century Learners
NCTE 01.13.21 Multimodal Literacies
This post was written by NCTE member Dianna Minor.
One of the major tasks I’ve embarked upon since my initial National Board Certification (NBCT) is collaborating with colleagues to integrate visual literacy in secondary classrooms, giving students opportunities to look beyond the printed text.
Visual literacy builds stronger readers, readers who are able to think about texts in numerous ways through a different lens, an important skill for critical readers and thinkers in the 21st century. Students skilled in visual literacy are able to create meaning from images, which in turn improves their writing proficiency and critical thinking skills. By integrating visual literacy into classrooms, we help students learn to collaborate and to discuss a wide range of ideas while expressing their own.
It is critical for students to be able to evaluate content/texts presented in diverse formats and media, a skill that can require much teacher modeling and independent practice. As students gain experience in interpreting works of art, infographics, film, videos, political cartoons, photographs, maps, advertisements, slide show presentations, and so on, they learn that they can use their imagination to see and think between and beyond the lines to draw inferences and conclusions. Visual literacy encourages student reflection, analysis, and evaluative thinking skills.
I’ve used visual literacy lessons to give students practice in analyzing tone, mood, and details in works of art. For example, in poetry lessons, I’ve modeled the Visual Thinking Strategy (VTS) when looking at photographs from the civil rights era. With this strategy, students focus on key questions:
- What’s going on in the photograph/art piece? (making inferences)
- What evidence do you see to support this? (looking for supporting evidence)
- What more can we find? (analyzing details to see how they connect as a whole)
Through these questions, students have discovered themes and identified main ideas, helping them understand the stories from the photographs.
In addition to photographs, I’ve integrated more works of art and paintings into my classroom so students have opportunities to analyze how two texts are similar and different and to discuss and compare the different approaches the author or artist takes.
Integrating visual literacy also gives quiet or reluctant students more opportunities to feel comfortable in the classroom; these lessons tend to be in small groups, allowing students to practice their own analysis through viewing, listening, and contributing.
With short stories and major literary works (essays, novels, longer pieces of text), teachers can pair texts with photographs and then ask students to draw evidence from informational texts to support analysis, reflection, and research. One useful tool for analyzing visual texts is the OPTIC strategy, in which the O stands for an overview , a general statement describing the photograph; P stands for important parts of the image, and could include inferences about what they contribute; T stands for how the title (or text) contributes to the meaning; I stands for interrelationships in the image—how the elements work together to create mood or meaning; C stands for conclusion , a statement that interprets the overall meaning. Using this framework, students can discuss the idea of claims and use detail and imagery to identify the central message of the photograph.
Visual literacy is invaluable to reader development in so many ways. It allows gradual development of the student reader’s understanding, slowing down the analysis process by making it more deliberate, and enabling students to build their own interpretation, to rely on their own powers of critical thinking.
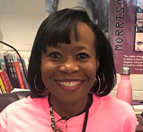
Dianna Minor is an educator, writer, and consultant. Her professional experience includes literacy and curriculum and instruction. Twitter: @diminor1
It is the policy of NCTE in all publications, including the Literacy & NCTE blog, to provide a forum for the open discussion of ideas concerning the content and the teaching of English and the language arts. Publicity accorded to any particular point of view does not imply endorsement by the Executive Committee, the Board of Directors, the staff, or the membership at large, except in announcements of policy, where such endorsement is clearly specified.

Readiness for Autonomy Among CFL Learners: A Malaysian Perspective
- Mizhe Xi Universiti Putra Malaysia
- Ng Chwee Fang Universiti Putra Malaysia
- Mohd Azidan Abdul Jabar Universiti Putra Malaysia
- Ilyana Jalaluddin Universiti Putra Malaysia
Learner autonomy is paramount in 21st-century education, representing a crucial qualification for lifelong learning. In the area of Chinese as a second/foreign language (CSL/CFL), it is also an important objective of curriculum instruction. However, there is a dearth of empirical research on learner autonomy in a wide range of cultural contexts and distinct domains of language use, especially in the context of CFL. This study aims to scrutinize the readiness for autonomy of CFL learners within the Malaysian settings. The research utilized a quantitative approach to analyze the data. A closed-ended questionnaire survey was conducted with 600 participants. Specifically, the data were synthesized through descriptive statistical analysis to concretely consider the respondents' readiness for learner autonomy in terms of perception of responsibility, assessment of ability, and engagement in the activities in Chinese learning. Findings indicated that Malaysian CFL learners demonstrated a medium readiness for autonomy in Chinese learning. While participants exhibit a relatively high perception of responsibility for autonomous learning, their ability to assess autonomy in decision-making within Chinese learning is somewhat unsatisfactory. Additionally, they express less enthusiasm about engaging in autonomous learning activities inside and outside the classroom. The current study illuminates theoretical and practical dimensions concerning the readiness for autonomy in Chinese learning among Malaysian CFL learners. Moreover, it is anticipated to offer implications for further studies on autonomy development among CFL learners in analogous contexts.
Author Biographies
Mizhe xi, universiti putra malaysia.
Department of Foreign Languages, Faculty of Modern Languages and Communication
Ng Chwee Fang, Universiti Putra Malaysia
Mohd azidan abdul jabar, universiti putra malaysia, ilyana jalaluddin, universiti putra malaysia.
Alrabai, F. (2017). From teacher dependency to learner independence: a study of Saudi learners’ readiness for autonomous learning of English as a Foreign Language. Learning and Teaching in Higher Education: Gulf Perspectives, 14(1), 70-97.
Abdel Razeq, A. A. (2014). University EFL learners’ perceptions of their autonomous learning responsibilities and abilities. RELC Journal, 45(3), 321-336.
Boekaerts, M., & Corno, L. (2005). Self-Regulation in the Classroom: A Perspective on Assessment and Intervention. Applied Psychology: An International Review, 54(2), 199–231.
Badak, E. F., & Şenel, M. (2022). The relationship between learner autonomy attitudes and lifelong learning tendencies of prospective English language teachers. Gazi Üniversitesi Gazi Eğitim Fakültesi Dergisi, 1(1), 339-362.
Benson, P. (2001). Teaching and Researching Autonomy in Language Learning. Longman.
Benson, P. (2005). (Auto)biography and learner diversity. In P. Benson, & D. Nunan (Eds.), Learners stories: difference and diversity in language learning (pp. 4-21). Cambridge University Press.
Benson, P. (2007). Autonomy in language teaching and learning. Language Teaching, 40(1), 21–40. https://doi.org/10.1017/ S0261444806003958
Benson, P. (2011). Teaching and Researching: Autonomy in Language Learning. Routledge. https://doi.org/https://doi.org/ 10.4324/9781315833767
Candy, P. (1991). Self-direction for lifelong learning: A comprehensive guide to theory and practice. Jossey-Bass Publishers.
Cotterall, S. (1995). Readiness for autonomy: Investigating learner beliefs. System, 23(2), 195-205.
Chan, V. (2001). Readiness for learner autonomy: What do our learners tell us?. Teaching in higher education, 6(4), 505-518.
Chan, V., M. Spratt & G. Humphreys. (2002). Autonomous language learning: Hong Kong tertiary students’ attitudes and behaviours. Evaluation and Research in Education, 16(1), 1–18.
Chan, V. (2003). Autonomous language learning: The teachers' perspectives. Teaching in Higher Education, 8(1), 33-54.
Cirocki, A., Anam, S., & Retnaningdyah, P. (2019). Readiness for autonomy in English language learning: The case of Indonesian high school students. Iranian Journal of Language Teaching Research, 7(2), 1-18.
Confucius Institute Headquarters & Han Ban. (2014). International Curriculum for Chinese Language Education, Beijing Language and Culture University Press.
Cheong, K. M., Jano, Z., Salam, S., Bareed, N. A., Ithnin, F., & Suraidi, N. (2019). Motivational Factors Among Undergraduates Learning Chinese: A Qualitative Perspective. International Journal of Academic Research in Progressive Education and Development, 8(2), 187–199.
Dam, L. (1995). Learner autonomy 3: From theory to classroom practice. Authentik.
Dickinson, L. (1995). Autonomy and motivation: A literature review. System, 23(2), 165–174.
Gavrilyuk, O. A. (2015). Autonomy as a Core Value of Lifelong Learning. Journal of Siberian Federal University. Humanities & Social Sciences, 8(11), 2283–2290. https://doi.org/10.17516/1997-1370-2015-8-11-2283-2290
Gremmo, M. J., & Riley, P. (1995). Autonomy, self-direction and self access in language teaching and learning: The history of an idea. System, 23(2), 151-164. https://doi.org/10.1016/0346-251X(95)00002-2
Holec, H. (1981). Autonomy in foreign language learning. Pergamon.
Ho, J., & Crookall, D. (1995). Breaking with Chinese cultural traditions: Learner autonomy in English language teaching. System, 23(2), 235-243.
Hossain, M. M., & Mustapha, S. M. B. (2020). The relationship between learner autonomy readiness and English language performance of undergraduates at public universities in Bangladesh. Journal of Education and Social Sciences, 16(1), 82-92.
Hedge, T. (2000). Teaching and Learning in the Language Classroom. Oxford university press.
Ismail, F., Yunus, M. M., & Zahidi, A. M. (2020). Promotion of Learner Autonomy: Beliefs and Practices from the ESL Teachers’ Perspectives. International Journal of Academic Research in Progressive Education and Development, 9(2), 309–317.
Jones, J.F. (1995). Self-access and Culture Retreating from Autonomy. ELT Journal, 49(3), 228-234. https://doi.org/10.1093/elt/49.3.228 .
Khairallah, M., Fleonova, O., & Nicolas, M. O. (2020). Understanding Students' Resistance to Autonomous Learning in an L2 English Language Course at a University in Lebanon. European Journal of Education (EJE), 3(1), 20-38.
Khalymon, I., & Shevchenko, S. (2017). Readiness for Learner Autonomy of Prospective Teachers Minoring in English. Advanced Education, 4(8), 65–71. https://doi.org/10.20535/2410-8286.107296
Krejcie, R. V. and Morgan, D.W. (1970). Determining sample size for research activities. Educational and Psychological measurement, 30(3), 607-610.
Little, D. (1995). Learning as dialogue: The dependence of learner autonomy on teacher autonomy. System, 23(2), 175– 182.
Little, D. (2004). Learner autonomy, teacher autonomy and the European Language Portfolio. UNTELE, Université de Compiègne, 17-20.
Littlewood, W. (1996). “Autonomy”: An anatomy and a framework. System, 24(4), 427-435.
Littlewood, W. (1999). Defining and developing autonomy in East Asian contexts. Applied Linguistic, 20(1), 71-94. https://doi.org/10.1093/applin/20.1.71
Lin, L., & Reinders, H. (2019). Students’ and teachers’ readiness for autonomy: Beliefs and practices in developing autonomy in the Chinese context. Asia Pacific Education Review, 20, 69-89.
Liu, H. J. (2015). Learner autonomy: The role of motivation in foreign language learning. Journal of Language Teaching and Research, 6(6), 1165-1174.
Malik, F. A., Asraf, R. M., Othman, K., & Muhamad, A. J. (2017). EFL Pre-University Students’ Readiness for Learner Autonomy: Does culture matter? Asian EFL Journal, (101), 83-95.
Nguyen, S. Van, & Habók, A. (2022). Non-English major students’ perceptions of aspects of their autonomous language learning. Language Learning in Higher Education, 12(1), 231–253.
Oxford, R. L. (1990). Language learning strategies: What every teacher should know (1sted.). Heinle ELT.
Orawiwatnakul, W., & Wichadee, S. (2017). An investigation of undergraduate students’ beliefs about autonomous language learning. International Journal of Instruction, 10(1), 117–132. https://doi.org/10.12973/iji.2017.1018a
Reinders, H. & White, C. (2011). Learner Autonomy and New Learning Environments. Language Learning & Technology, 15(3), 1–3.
Şenbayrak, M., Ortaçtepe, D., & Trimble, K. (2019). An exploratory study on Turkish EFL learners’ readiness for autonomy and attitudes toward self-access centers. TESOL Journal, 10(2), 1-17. https://doi.org/10.1002/tesj.401
Sim, M. S., Khin, L. S., & K. Sankaran, S. S. A. (2019). The Error Analysis of Learning Chinese Endocentric Phrases among the Malay Students in Malaysia. International Journal of Modern Languages and Applied Linguistics, 3(2), 49-62.
Sakai, S., Takagi, A, & Chu, M.P. (2010). Promoting Learner Autonomy: Student Perceptions of Responsibilities in a Language Classroom in East Asia. Educational Perspectives, 43(1), 12–27.
Sheng, L & Zhang,Y. H.(2021). Han guo han yu xue xi zhe er yu zi wo dui zi zhu xue xi xing wei ying xiang de shi zheng yan jiu[An empirical study on the efficient of Chinese learners’L2 self on autonomous learning behavior in Korea]. Language Teaching and Research, 209(3), 45-57.
Scharle, A., & Szabó, A. (2000). Learner Autonomy: A Guide to Developing Learner Responsibility. Cambridge University Press.
Thavenius, C. (1999). Teacher autonomy for learner autonomy. In S. Cotterall, & D. Crabbe (Eds.), Learner autonomy in language learning: Defining the field and effecting change (pp. 159-163). Peter Lang.
Thang, S.M. (2001). Malaysian learners’ conceptions of their learning processes and their perceptions of their English as a Second Language courses in a tertiary distance learning context. [Unpublished PhD thesis]. University of Nottingham.
Thang, S. M. (2005). Investigating Malaysian distance learners’ perceptions of their English Proficiency Courses, Open Learning, 20(3), 243-256.
Thang, S. M. & Alias, A. (2007). Investigating readiness for autonomy: a comparison of Malaysian ESL undergraduates of three public universities. Reflections on English Language Teaching, 6(1), 1-18.
Thang, S. M. (2009). Investigating Autonomy of Malaysian ESL Learners: Comparison between Public and Private Universities. 3L Language, Linguistics and Literature. The South East Asia Journal of English Language Studies. Vol 15, 97-124.
Tuan, D. M. (2021). Learner autonomy in English language learning: Vietnamese EFL students’ perceptions and practices. Indonesian Journal of Applied Linguistics, 11(2), 307-317.
Tan, T. G., Ismail, H. N., Hoe, F. T., & Ho, C. C. (2015). The motivation of undergraduates learning Chinese as a foreign language. Academia Journal UiTMT, 4(1), 1-16.
Üstünlüoğlu, E. (2009). Autonomy in language learning: Do student take responsibility for their learning? Journal of Theory and Practice in Education, 5(2), 148-169.
Wenden, A. (1991). Learner strategies for learner autonomy. Prentice Hall.
Win, C. C. (2022). Myanmar English majors’ perceptions of learner autonomy in Learning English at tertiary level. Journal of Adult Learning, Knowledge and Innovation, 5(2), 79–90. https://doi.org/10.1556/2059.2022.00059
YILDIRIM, Ö. (2008). Turkish EFL learners’ readiness for learner autonomy. Journal of Language and Linguistic Studies, 4(1),65-80.
Yıldırım, S. (2012). Teacher support, motivation, learning strategy use, and achievement: A multilevel mediation model. The Journal of Experimental Education, 80(2), 150-172.
Zakaria, N. L., Aziz, A. A., & Ramayah, K. (2017). Language learning strategies and learner autonomy in learning Japanese. Journal of Advanced Research in Social and Behavioural Sciences, 9(3), 50-60.
Zimmerman, B. J. (2000). Attaining self-regulation: A social cognitive perspective. In M. Boekaerts, P. R. Pintrich, & M. Zeidner (Eds.), Handbook of self-regulation (pp. 13–39). Academic Press.
Copyright © 2015-2024 ACADEMY PUBLICATION — All Rights Reserved
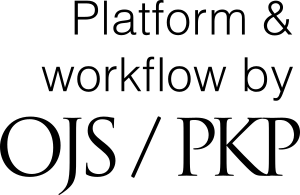

IMAGES
VIDEO
COMMENTS
21st-century learning is more than just the 3Rs (reading, writing, and arithmetic). It emphasizes the importance of critical thinking, creativity, collaboration, and communication - skills essential for students to thrive in the 21st century. What is also clear is that 21st-century learning cannot occur in a traditional classroom setting.
"Learning in the 21st Century: Research, Innovation and Policy" 21st Century Learning: Research, Innovation and Policy ... A set of key findings has emerged from learning sciences research: the importance of learning deeper conceptual understanding, rather than superficial facts and procedures, the importance of learning ...
The 21st-century skillset is generally understood to encompass a range of competencies, including critical thinking, problem solving, creativity, meta-cognition, communication, digital and technological literacy, civic responsibility, and global awareness (for a review of frameworks, see Dede, 2010).And nowhere is the development of such competencies more important than in developing country ...
These labels include both cognitive and non-cognitive skills- such as critical thinking, problem solving, collaboration, effective communication, motivation, persistence, and learning to learn. 21st century skills also include creativity, innovation, and ethics that are important to later success and may be developed in formal or informal ...
Research has shown that students applying problem-based learning increase their participation in class activities ... 21st century learning skills. When teachers applied this strategy, they supported students' capacities in critical thinking, self-directed ... linking knowledge with the real world is an important component in the 21st century ...
Recognising the importance of minimising the discrepancies between changes in the world and the type of education available to children, many countries worked together to develop the OECD Learning Compass for 2030, a new learning framework for the future . It was co-created as a globally shared vision for the types of competencies (knowledge ...
Towards a 21st Century Curriculum For the first time, the OECD Future of Education and Skills 2030 project conducted comprehensive curriculum analyses through the co-creation of new knowledge with a wide range of stakeholders including policy makers, academic experts, school leaders, teachers, NGOs, other social partners and, most importantly ...
E-learning is the subject of M.Phil. and doctorate research in a variety of subjects. Few Education is the only focus of international research publications. e-learning is the most common form of ...
development of learning environments for the 21st century. Learners at the centre ~ Learners are the central players in the environment and therefore activities centre on their cognition and growth. ~ Learning activities allow students to construct their learning through engagement and active exploration.
These are important considerations, but 21st century teaching and learning goes beyond technology integration and STEM content; it is also about fostering ways of thinking and promoting dispositions that support success in an age driven by rapidly changing and expanding technologies. Responsive 21st century teaching and caregiving requires ...
Education goals have evolved to emphasize student acquisition of essential "21 st Century skills", which define the knowledge and attributes necessary to successfully contribute to the workforce and global economy of the 21st Century (National Research Council, 2011, 2012a).In general, these standards seek to transition from emphasizing content-based drilling and memorization towards ...
iStock/Getty. The term "21st-century skills" is generally used to refer to certain core competencies such as collaboration, digital literacy, critical thinking, and problem-solving that ...
How about that last characteristic of a 21st-century learner, effective communicator? Being literate means one who is advanced at reading, writing, speaking, and listening. And, in all schools -- deeper learning driven or not -- literacy is a curriculum fundamental. Its importance President Obama explained in a speech a few years back:
The challenge is that there is no single framework for what is included in the list of skills. The Partnership for 21st Century Skills framework (Dede, 2010) has two categories of skill groups. The first is "perennial" skills, or those retained from the 20th century, but are still valuable in the 21st century.
Abstract. The term '21st century learner' emerged at the turn of the millennium and evoked a certain type of digitally-agile and self-driven learner. These ideas about 21st century learners ...
In their Framework for 21 st Century Learning , P21 recognizes the importance of teachers developing their students' learning and innovation skills. These skills, which are often referred to as ...
As well as literacy and numeracy skills, critical thinking, problem solving, decision making, creativity and innovation, communication, self-management, social responsibility and digital fluency are the interconnected skills viewed as critical to promoting the Albertan 21st Century Learner. While most systems have been active in developing ...
Classrooms with 21st Century learning environments are well‐equipped with computer hardware, software, electronic whiteboards and rich digital and online curricular resources. When you enter a 21st Century learning environment, you quickly see that interactive learning, higher level thinking skills, and student engagement are pervasive ...
This becomes increasingly complex in 21st century settings, calling for innovation and going beyond the heroics of individual leaders. Many need to be involved, bringing in diverse partners at different levels. This is all explored in this volume. It clarifies the concepts and the dimensions of learning leadership, relating it to extensive ...
Visual literacy is invaluable to reader development in so many ways. It allows gradual development of the student reader's understanding, slowing down the analysis process by making it more deliberate, and enabling students to build their own interpretation, to rely on their own powers of critical thinking. Dianna Minor is an educator, writer ...
The "21st century learner skills use scale", used to measure pre-service teachers' 21st century . Contemporary Research in Education 2022 21 century Century Learner Skills and Metacognitive Awareness 21st century learner skills Metacognitive awareness.
Toro, William Xavier, "21st CENTURY LEARNING SKILLS IN EDUCATION AND EMPLOYABILITY" (2019). Theses and Dissertations. 74. ... The purpose of this mixed-methods study was to research a diverse school in Westchester County, New York to analyze whether it is aligned to 21 ... Table 4.3 Most Important 21. st. Century Skills ...
The results of this research show a statistically significant influence between the use of learning models on students' 21st-century skills posttest scores. Post-hoc testing revealed that students in the STEM-EDELCY group had a higher level of 21st-century skills than students in the 5E-learning cycle group and the guided inquiry group.
Therefore, learning in the 21st century is expected to open wider employment opportunities and expand employment opportunities for Indonesian people as quality and superior human resources ...
Learner autonomy is paramount in 21st-century education, representing a crucial qualification for lifelong learning. In the area of Chinese as a second/foreign language (CSL/CFL), it is also an important objective of curriculum instruction. However, there is a dearth of empirical research on learner autonomy in a wide range of cultural contexts and distinct domains of language use, especially ...
The role of technology in landscape architecture (TLA) has significantly evolved since the 19th century, increasingly integrating with digital tools and technologies in the 21st century. Despite its growing importance, there is a notable deficiency in the scholarly literature regarding the progression of TLA trends and their interplay with the core domains and research themes within landscape ...
AP Seminar and AP Research students to submit performance tasks as final and their presentations to be scored by their AP Seminar or AP Research teachers. AP Computer Science Principles students to submit their Create performance task as final. Late Testing . Occasionally, circumstances make it necessary for students to test late.