- Research article
- Open access
- Published: 25 May 2023

Effects of ketogenic diet on health outcomes: an umbrella review of meta-analyses of randomized clinical trials
- Chanthawat Patikorn 1 , 2 ,
- Pantakarn Saidoung 1 ,
- Tuan Pham 3 ,
- Pochamana Phisalprapa 4 ,
- Yeong Yeh Lee 5 ,
- Krista A. Varady 6 ,
- Sajesh K. Veettil 1 &
- Nathorn Chaiyakunapruk 1 , 7
BMC Medicine volume 21 , Article number: 196 ( 2023 ) Cite this article
14k Accesses
15 Citations
88 Altmetric
Metrics details
Systematic reviews and meta-analyses of randomized clinical trials (RCTs) have reported the benefits of ketogenic diets (KD) in various participants such as patients with epilepsy and adults with overweight or obesity . Nevertheless, there has been little synthesis of the strength and quality of this evidence in aggregate.
To grade the evidence from published meta-analyses of RCTs that assessed the association of KD, ketogenic low-carbohydrate high-fat diet (K-LCHF), and very low-calorie KD (VLCKD) with health outcomes, PubMed, EMBASE, Epistemonikos, and Cochrane database of systematic reviews were searched up to February 15, 2023. Meta-analyses of RCTs of KD were included. Meta-analyses were re-performed using a random-effects model. The quality of evidence per association provided in meta-analyses was rated by the GRADE (Grading of Recommendations, Assessment, Development, and Evaluations) criteria as high, moderate, low, and very low.
We included 17 meta-analyses comprising 68 RCTs (median [interquartile range, IQR] sample size of 42 [20–104] participants and follow-up period of 13 [8–36] weeks) and 115 unique associations. There were 51 statistically significant associations (44%) of which four associations were supported by high-quality evidence (reduced triglyceride ( n = 2), seizure frequency ( n = 1) and increased low-density lipoprotein cholesterol (LDL-C) ( n = 1)) and four associations supported by moderate-quality evidence (decrease in body weight, respiratory exchange ratio (RER), hemoglobin A 1c , and increased total cholesterol). The remaining associations were supported by very low (26 associations) to low (17 associations) quality evidence. In overweight or obese adults, VLCKD was significantly associated with improvement in anthropometric and cardiometabolic outcomes without worsening muscle mass, LDL-C, and total cholesterol. K-LCHF was associated with reduced body weight and body fat percentage, but also reduced muscle mass in healthy participants.
Conclusions
This umbrella review found beneficial associations of KD supported by moderate to high-quality evidence on seizure and several cardiometabolic parameters. However, KD was associated with a clinically meaningful increase in LDL-C. Clinical trials with long-term follow-up are warranted to investigate whether the short-term effects of KD will translate to beneficial effects on clinical outcomes such as cardiovascular events and mortality.
Peer Review reports
Ketogenic diets (KD) have received substantial attention from the public primarily due to their ability to produce rapid weight loss in the short run [ 1 , 2 ]. The KD eating pattern severely restricts carbohydrate intake to less than 50 g/day while increasing protein and fat intake [ 3 , 4 , 5 , 6 ]. Carbohydrate deprivation leads to an increase in circulating ketone bodies by breaking down fatty acids and ketogenic amino acids. Ketones are an alternative energy source from carbohydrates that alter physiological adaptations. These adaptions have been shown to produce weight loss with beneficial health effects by improving glycemic and lipid profiles [ 7 , 8 ]. KD has also been recommended as a nonpharmacological treatment for medication-refractory epilepsy in children and adults [ 8 , 9 ]. Evidence suggests that KD has reduced seizure frequency in patients with medication-refractory epilepsy, and even allowing some patients to reach complete and sustained remission. 11 However, the exact anticonvulsive mechanism of KD remains unclear [ 10 , 11 ].
Several systematic reviews and meta-analyses of randomized clinical trials (RCTs) have reported on the use of KD in patients with obesity or type 2 diabetes mellitus (T2DM) to control weight and improve cardiometabolic parameters [ 1 , 12 , 13 , 14 , 15 ], in patients with refractory epilepsy to reduce seizure frequency [ 16 ], and in athletes to control weight and improve performance [ 17 ]. To date, there has been little synthesis of the strength and quality of this evidence in aggregate. This umbrella review therefore aims to systematically identify relevant meta-analyses of RCTs of KD, summarize their findings, and assess the strength of evidence of the effects of KD on health outcomes.
The protocol of this study was registered with PROSPERO (CRD42022334717). We reported following the 2020 Preferred Reporting Items for Systematic Reviews and Meta-analyses (PRISMA) (Additional file 1 ) [ 18 ]. Difference from the original review protocol is described with rationale in Additional file 2 : Table S1.
Search strategy and eligibility criteria
We searched PubMed, EMBASE, Epistemonikos, and the Cochrane database of systematic reviews (CDSR) from the database inception to February 15, 2023 (Additional file 2 : Table S2). No language restriction was applied. Study selection was independently performed in EndNote by two reviewers (C.P. and PS). After removing duplicates, the identified articles' titles and abstracts were screened for relevance. Full-text articles of the potentially eligible articles were retrieved and selected against the eligibility criteria. Any discrepancies were resolved by discussion with the third reviewer (SKV).
We included studies that met the following eligibility criteria: systematic reviews and meta-analyses of RCTs investigating the effects of any type of KD on any health outcomes in participants with or without any medical conditions compared with any comparators. When more than 1 meta-analysis was available for the same research question, we selected the meta-analysis with the largest data set [ 19 , 20 , 21 ]. Articles without full-text and meta-analyses that provided insufficient or inadequate data for quantitative synthesis were excluded.
Data extraction and quality assessment
Two reviewers (CP and PS) independently performed data extraction and quality assessment (Additional file 2 : Method S1). Discrepancies were resolved with consensus by discussing with the third reviewer (SKV). We used AMSTAR- 2 -A Measurement Tool to Assess Systematic Reviews- to grade the quality of meta-analyses as high, moderate, low, or critically low by assessing the following elements, research question, a priori protocol, search, study selection, data extraction, quality assessment, data analysis, interpretation, heterogeneity, publication bias, source of funding, conflict of interest [ 22 ].
Data synthesis
For each association, we extracted effect sizes (mean difference [MD], the standardized mean difference [SMD], and risk ratio [RR]) of individual studies included in each meta-analysis and performed the meta-analyses to calculate the pooled effect sizes and 95% CIs using a random-effects model under DerSimonian and Laird [ 23 ], or the Hartung-Knapp- Sidik-Jonkman approach for meta-analyses with less than five studies [ 24 ]. p < 0.05 was considered statistically significant in 2-sided tests. Heterogeneity was evaluated using the I 2 statistic. The evidence for small-study effects was assessed by the Egger regression asymmetry test [ 25 ]. Statistical analyses were conducted using Stata version 16.0 (StataCorp). We presented effect sizes of statistically significant associations with the known or estimated minimally clinically important difference (MCID) thresholds for health outcomes [ 14 , 26 , 27 , 28 , 29 , 30 ].
We assessed the quality of evidence per association by applying the GRADE criteria (Grading of Recommendations, Assessment, Development, and Evaluations) in five domains, including (1) risk of bias in the individual studies, (2) inconsistency, (3) indirectness, (4) imprecision, and (5) publication bias [ 31 ]. We graded the strength of evidence (high, moderate, low, and very low) using GRADEpro version 3.6.1 (McMaster University).
Sensitivity analyses
Sensitivity analyses were performed by excluding small-size studies (< 25 th percentile) [ 32 ] and excluding primary studies having a high risk of bias rated by the Cochrane’s risk of bias 2 tool (RoB 2) for RCTs from the identified associations [ 19 , 20 , 21 , 33 ].
Seventeen meta-analyses were included (Fig. 1 and Additional file 2 : Table S3) [ 1 , 2 , 15 , 16 , 17 , 34 , 35 , 36 , 37 , 38 , 39 , 40 , 41 , 42 , 43 , 44 , 45 ]. These meta-analyses comprised 68 unique RCTs with a median (interquartile range, IQR) sample size per RCT of 42 (20–104) participants and a median (IQR) follow-up period of 13 (8–36) weeks. The quality of meta-analyses assessed using AMSTAR-2 found that none were rated as high confidence, 2 (12%) as moderate confidence, 2 (12%) as low confidence, and 13 (76.0%) as critically low confidence (Table 1 and Additional file 2 : Table S4).
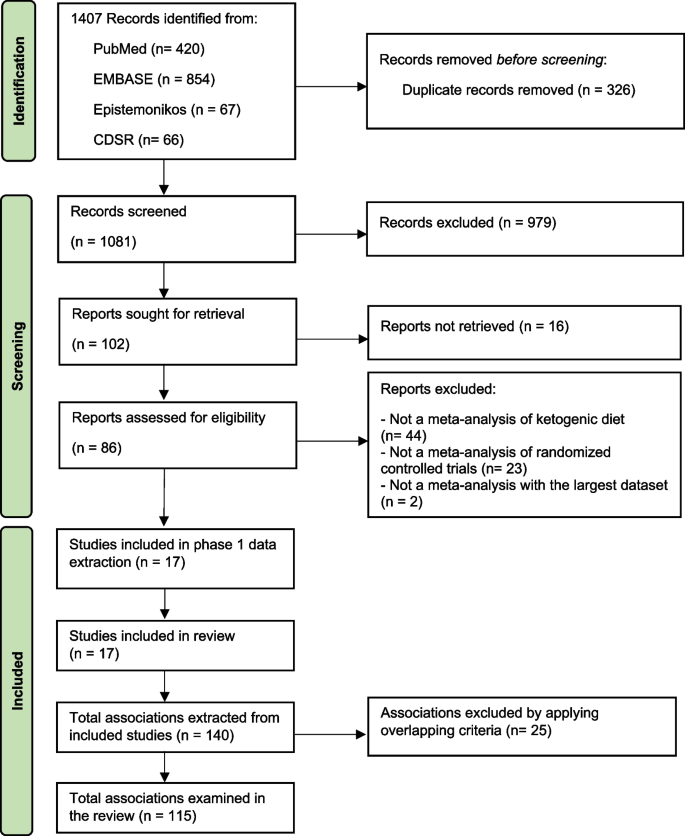
Study selection flow of meta-analyses. Abbreviation: CDSR, Cochrane database of systematic review
Types of KD identified in this umbrella review were categorized as (1) KD, which limits carbohydrate intake to < 50 g/day or < 10% of the total energy intake (TEI) [ 35 ], (2) ketogenic low-carbohydrate, high-fat diet (K-LCHF), which limits carbohydrate intake to < 50 g/day or < 10% of TEI with high amount of fat intake (60–80% of TEI) [ 38 , 46 ], (3) very low-calorie KD (VLCKD), which limits carbohydrate intake to < 30–50 g/day or 13–25% of TEI with TEI < 700–800 kcal/day, and (4) modified Atkins diet (MAD), which generally limits carbohydrate intake to < 10 g/day while encouraging high-fat foods [ 15 , 47 ]. Meta-analyses of long-chain triglyceride KD, medium-chain triglyceride KD, and low glycemic index treatment were not identified.
Description and summary of associations
We identified 115 unique associations of KD with health outcomes (Additional file 2 : Table S5). The median (IQR) number of studies per association was 3 [ 4 , 5 , 6 ], and the median (IQR) sample size was 244 (127–430) participants. Outcomes were associated with KD types, including 40 (35%) KD, 18 (16%) K-LCHF, 13 (11%) VLCKD, 25 (22%) KD or K-LCHF, 5 (4%) KD or VLCKD, 1 (1%) KD or MAD, and 13 (11%) KD, K-LCHF, or VLCKD.
The associations involved 40 (35%) anthropometric measures (i.e., body weight, body mass index [BMI] [calculated as weight in kilograms divided by height in meters squared], waist circumference, muscle mass, fat mass, body fat percentage, and visceral adipose tissue), 37 (32%) lipid profile outcomes (i.e., triglyceride, total cholesterol, high-density lipoprotein cholesterol [HDL-C], and low-density lipoprotein cholesterol [LDL-C]), 22 (19%) glycemic profile outcomes (i.e., hemoglobin A 1c [HbA 1c ], fasting plasma glucose, fasting insulin, and homeostatic model assessment of insulin resistance [HOMA-IR]), 6 (5%) exercise performance (i.e., maximal heart rate, respiratory exchange ratio [RER], maximal oxygen consumption (VO 2 max), 5 (4%) blood pressure outcomes (i.e., systolic blood pressure [SBP], diastolic blood pressure [DBP], and heart rate), 1 (1%) outcome associated with seizure frequency reduction ≥ 50% from baseline, and 3 other outcomes (i.e., serum creatinine, C-peptide, and C-reactive protein). In addition, there is 1 association (1%) of adverse events.
Participants in the identified associations included 68 (59%) associations in adults with overweight or obesity with or without T2DM or dyslipidemia, 15 (13%) athletes or resistance-trained adults, 12 (10%) adults with T2DM, 11 (10%) healthy participants ≥ 16 years old, 8 (7%) cancer patients, and 1 (1%) in children and adolescents with epilepsy.
Using GRADE, 115 associations were supported by very low strength of evidence ( n = 66, 57%), with the remaining being low ( n = 36, 31%), moderate ( n = 9, 8%), and high quality of evidence ( n = 4, 3%) (Additional file 2 : Table S5). Almost half, or 44% (51 associations), were statistically significant based on a random-effects model, of which 51% (26 associations) were supported by a very low level of evidence, followed by low (17 associations [33%]), moderate (4 associations [8%]), and high (4 associations [8%]) levels of evidence. Overall beneficial outcomes associated with KD were BMI [ 37 , 42 ], body weight [ 1 , 2 , 35 , 36 , 37 , 41 ], waist circumference [ 37 , 42 ], fat mass [ 37 , 42 ], body fat percentage [ 38 , 40 ], visceral adipose tissue [ 37 ], triglyceride [ 1 , 2 , 36 , 42 ], HDL-C [ 1 , 2 , 42 ], HbA 1c [ 2 , 34 , 35 ], HOMA-IR [ 2 , 42 ], DBP [ 1 ], seizure frequency reduction ≥ 50% from baseline [ 16 ], and respiratory exchange ratio [ 17 , 39 ]. Adverse outcomes associated with KD were reduced muscle mass [ 37 , 38 ], and increased LDL-C [ 2 , 35 ], and total cholesterol [ 2 , 17 ]. In terms of safety, one association showed no significant increase in adverse events (e.g., constipation, abdominal pain, and nausea) with KD [ 44 ].
Eight out of 13 associations supported by moderate to high-quality evidence were statistically significant (Table 2 ). There were 4 statistically significant associations supported by high-quality evidence, including the following: (1) KD or MAD for 3–16 months was associated with a higher proportion of children and adolescents with refractory epilepsy achieving seizure frequency reduction ≥ 50% from baseline compared with regular diet (RR, 5.11; 95% CI, 3.18 to 8.21) [ 16 ], (2) KD for 3 months was associated with reduced triglyceride in adults with T2DM compared with regular diet (MD, -18.36 mg/dL; 95% CI, -24.24 to -12.49, MCID threshold 7.96 mg/dL) [ 14 , 35 ], (3) KD for 12 months was associated with reduced triglyceride in adults with T2DM compared with regular diet (MD, -24.10 mg/dL; 95% CI, -33.93 to -14.27, MCID threshold 7.96 mg/dL) [ 14 , 35 ], and (4) KD for 12 months was associated with increased LDL-C in adults with T2DM compared with regular diet (MD, 6.35 mg/dL; 95% CI, 2.02 to 10.69, MCID threshold 3.87 mg/dL) [ 14 , 35 ]. In addition, there were 4 statistically significant associations supported by moderate-quality evidence: (1) KD for 3 months was associated with reduced HbA 1c in adults with T2DM compared with regular diet (MD, -0.61%; 95% CI, -0.82 to -0.40, MCID threshold 0.5%) [ 14 , 35 ], (2) VLCKD for 4–6 weeks was associated with reduced body weight in T2DM adults with overweight or obesity compared with a low-fat diet or regular diet (MD, -9.33 kg; 95% CI, -15.45 to -3.22, MCID threshold 4.40 kg) [ 14 , 15 ], (3) K-LCHF for 4–6 weeks was associated with reduced respiratory exchange ratio in athletes compared with a high-carbohydrate diet (SMD, -2.66; 95% CI, -3.77 to -1.54) [ 39 ], and (4) K-LCHF for 11–24 weeks was associated with increased total cholesterol in athletes compared with regular diet (MD, 1.32 mg/dL; 95% CI, 0.64 to 1.99) [ 14 , 17 ].
Types of KD showed different effects on health outcomes with changes more than the MCID thresholds in different populations (Fig. 2 ). KD or MAD for 3–16 months was associated with a 5-times higher proportion of children and adolescents with refractory epilepsy achieving seizure frequency reduction ≥ 50% from baseline compared with a regular diet (RR, 5.11; 95% CI, 3.18 to 8.21) [ 16 ]. In healthy participants, K-LCHF for 3–12 weeks could reduce body weight by 3.68 kg (95% CI, -4.45 to -2.90) but also significantly reduced muscle mass by 1.27 kg (95% CI, -1.83 to -0.70, MCID threshold 1.10 kg) [ 14 , 26 , 38 ]. In adults with T2DM, KD for 3–12 months was found to have significant associations with changes more than the MCID thresholds, including reduction of triglyceride and HbA 1c ; however, KD for 12 months led to a clinically meaningful increase in LDL-C by 6.35 mg/dL (95% CI, 2.02 to 10.69, MCID threshold 3.87 mg/dL) [ 14 , 35 ]. In adults with overweight or obesity and/or metabolic syndrome, VLCKD for 4–6 weeks demonstrated a clinically meaningful weight loss of 9.33 kg (95% CI, -15.45 to -3.22, MCID threshold 4.40 kg) [ 14 , 15 ]. VLCKD for 3–96 weeks led to a clinically meaningful improvement in BMI, body weight, waist circumference, triglyceride, fat mass, and insulin resistance, while preserving muscle mass [ 42 ].
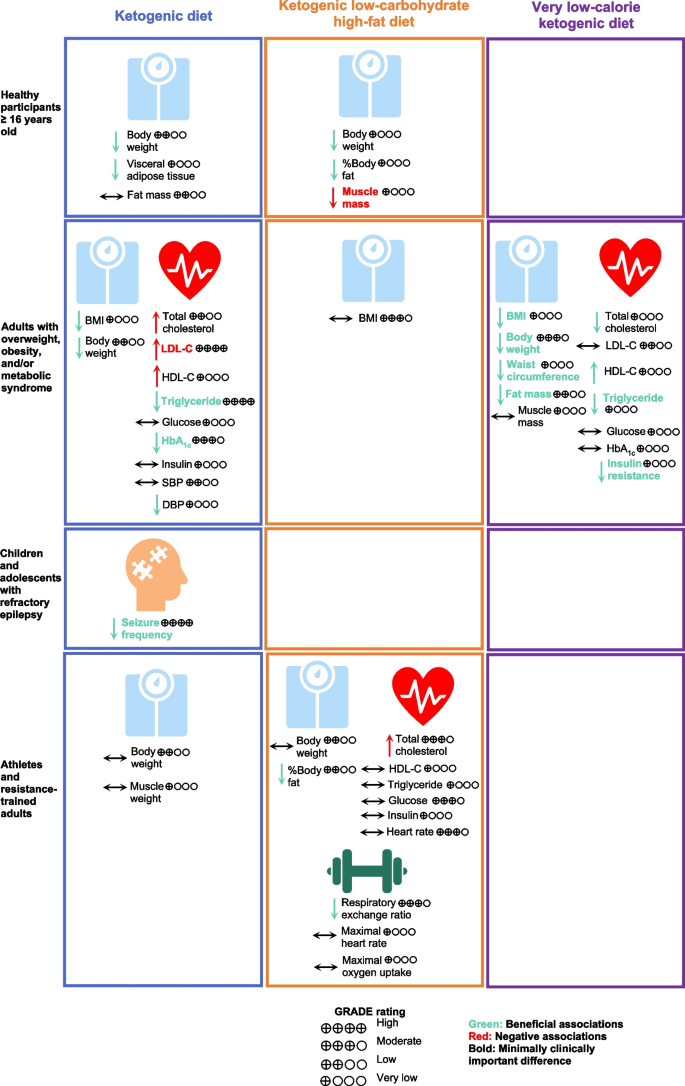
Associations of Types of Ketogenic Diet with Health Outcomes. Abbreviations: BMI, body mass index, DBP, diastolic blood pressure; GRADE, Grading of Recommendations, Assessment, Development, and Evaluations; HbA 1c , hemoglobin A 1c ; HDL-C, high-density lipoprotein cholesterol; HOMA-IR, homeostatic model of insulin resistance; LDL-C, low-density lipoprotein cholesterol; SBP, systolic blood pressure; TEI, total energy intake
Excluding RCTs with small sizes in 7 associations found that the strength of evidence of one association was downgraded to very low quality, i.e., KD for 12 months, and the increase of LDL-C in adults with T2DM compared with a control diet. Another association was downgraded to low quality, i.e., KD for 12 months and the reduction of triglyceride in adults with T2DM compared with the control diet (Additional file 2 : Table S6). The remaining associations retained the same rank.
This umbrella review was performed to systematically assess the potential associations of KD and health outcomes by summarizing the evidence from meta-analyses of RCTs. Sensitivity analyses were performed to provide additional evidence from high-quality RCTs, which further increased the reliability of results. We identified 115 associations of KD with a wide range of outcomes. Most associations were rated as low and very low evidence according to the GRADE criteria because of serious imprecision and large heterogeneity in findings, and indirectness due to a mix of different interventions and comparators.
Our findings showed that KD or MAD resulted in better seizure control in children and adolescents with medication-refractory epilepsy (approximately a third of cases) for up to 16 months [ 10 , 11 , 16 ]. Anti-epileptic mechanisms of KD remain unknown but are likely multifactorial. Enhanced mitochondrial metabolism and an increase in ketone bodies or reduction in glucose across the blood–brain barrier resulted in synaptic stabilization [ 48 , 49 , 50 ]. Other mechanisms include an increase in gamma-aminobutyric acid (GABA) [ 51 ], more beneficial gut microbiome [ 52 ], less pro-inflammatory markers [ 53 ], and epigenetic modifications (e.g. beta-hydroxybutyrate [beta-OHB]) [ 54 ].
In adults, KD was associated with improved anthropometric measures, cardiometabolic parameters, and exercise performance. Our findings, however, demonstrated differences in the level of associations with type of KD. On the one hand, VLCKD is very effective in producing weight loss while preserving muscle mass in adults with overweight or obesity, with specific benefits on anthropometric and cardiometabolic parameters [ 15 , 42 ]. On the other hand, a significant portion of the weight loss seen in K-LCHF was due to muscle mass loss [ 17 , 38 ]. Overall KD was negatively associated with reduced muscle mass and increased LDL-C and total cholesterol.
Our findings demonstrated that KD could induce a rapid weight loss in the initial phase of 6 months, after which time further weight loss was hardly achieved [ 35 ]. Furthermore, weight loss induced by KD is relatively modest and appears comparable to other dietary interventions that are effective for short-term weight loss, e.g., intermittent fastingand Mediterranean diet [ 55 , 56 , 57 ].
KD is one of the dietary interventions employed by individuals to achieve rapid weight loss, which usually comes with reduced muscle mass [ 58 ]. However, KD has been hypothesized to preserve muscle mass following weight loss based on several mechanisms, including the protective effect of ketones and its precursors on muscle tissue [ 59 , 60 , 61 ], and increased growth hormone secretion stimulated by low blood glucose to increase muscle protein synthesis [ 58 , 62 , 63 ].
With regards to KD effects on lipid profiles, our results demonstrate an effective reduction in serum triglyceride levels with 3 months of lowered dietary carbohydrate intake, with even further reduction by month 12 [ 35 ]. Triglyceride levels are consistently shown to decrease after KD. Acute ketosis (beta-OHB ≈ 3 mM) due to ketone supplementation also shows decreases in triglycerides, indicating a potential effect of ketones on triglycerides independent of weight loss. One possible mechanism is the decreased very low-density lipoprotein content in the plasma due to low insulin levels. Due to a lack of insulin, lipolysis increases in fat cells [ 2 , 13 , 15 ]. Of note, the converse has also been observed as a phenomenon known as carbohydrate-induced hypertriglyceridemia, whereby higher dietary carbohydrate intake leads to higher serum triglycerides levels, potentially mediated by changes in triglyceride clearance and hepatic de novo lipogenesis rates [ 64 ]. Though our aggregate results also confirm an increase in LDL-C and total cholesterol with KD and K-LCHF, respectively, it is important to note that an increase in either of these levels does not necessarily signify a potentially deleterious cardiovascular end-point. This qualification derives from the fact that LDL particles are widely heterogeneous in composition and size, with small dense LDL particles being significantly more atherogenic than larger LDL particles [ 65 ]. Our observed aggregate effect of KD on cholesterol levels does not account for the difference in LDL particle size, nor does it distinguish the sources of dietary fat, which can also be a significant effector of LDL particle size distribution and metabolism [ 66 ].
Most RCTs of KD were conducted in patients with a limited group of participants, such as those with overweight, obesity, metabolic syndrome, cancer, and refractory epilepsy. In addition, most outcomes measured were limited to only surrogate outcomes. Thus, more clinical trials with a broader scope in populations and outcomes associated with KD would expand the role of KD in a clinical setting. For example, participant selection could be expanded from previous trials to include elderly patients, nonalcoholic fatty live disease (NAFLD) patients, and polycystic ovarian syndrome patients. Outcomes of interest of could be expanded to include (1) clinical outcomes such as cardiovascular events and liver outcomes, (2) short- and long-term safety outcomes such as adverse events (e.g., gastrointestinal, neurological, hepatic, and renal), eating disorder syndrome, sleep parameters, lipid profiles, and thyroid function and (3) other outcomes such as adherence and quality of life. More importantly, long-term studies are needed to investigate the sustainability of the clinical benefits of KD.
Our findings are useful to support the generation of evidence-based recommendations for clinicians contemplating use of KD in their patients, as well as for the general population. We further emphasize the importance of consultation with healthcare professionals before utilizing KD and any other dietary interventions. We demonstrated the benefits of KD on various outcomes in the short term. However, these improvements may prove difficult to sustain in the long term because of challenges in adherence. As for any diet interventions to achieve sustainable weight loss, factors of success include adherence, negative energy balance, and high-quality foods. Thus, communication and education with KD practitioners are important to ensure their adherence to the diet. Some individuals might benefit from switching from KD to other dietary interventions to maintain long-term weight loss.
Limitations
This umbrella review has several limitations. Firstly, we focused on published meta-analyses which confined us from assessing the associations of KD on outcomes and populations that were not included in existing meta-analyses. Secondly, most of the included meta-analyses were rated with AMSTAR-2 as critically low confidence, mainly due to a lack of study exclusion reasons, unexplained study heterogeneity, and unassessed publication bias. However, these domains unlikely affected our findings. Thirdly, we could not perform a dose–response analysis to understand the effects of different levels of carbohydrate intake on health outcomes because of insufficient details of carbohydrate intake reported in the meta-analyses. Fourthly, most RCTs of KD were limited to a relatively small number of participants with a short-term follow-up period, which limited our assessment of sustained beneficial effects after stopping KD. Lastly, due to decreased adherence, carbohydrate intake most likely increased across the course of the trials. For example, subjects in the KD arm of the A TO Z Weight Loss Study [ 67 ], started with a carbohydrate intake < 10 g/day but ended at 12 months with a carbohydrate intake accounting for 34% of TEI. In the DIRECT trial, subjects in the KD group started with carbohydrate intake of 20 g/day and ended at 12 months with 40% of TEI from carbohydrate intake [ 68 ]. Thus, we cannot be certain how the precise degree of ketosis contributed to the beneficial effects noted.
Beneficial associations of practicing KD were supported by moderate- to high-quality evidence, including weight loss, lower triglyceride levels, decreased HbA 1c , RER, and decreased seizure frequency. However, KD was associated with a clinically meaningful increase in LDL-C. Clinical trials with long-term follow-up are warranted to investigate whether these short-term effects of KD will translate to beneficial effects on more long-term clinical outcomes such as cardiovascular events and mortality.
Availability of data and materials
All data generated or analysed during this study are included in this published article and its supplementary information files.
Abbreviations
Beta-hydroxybutyrate
Body mass index
Diastolic blood pressure
Gamma-aminobutyric acid
High-density lipoprotein cholesterol
Hemoglobin A 1c
Homeostatic model assessment of insulin resistance
Ketogenic low-carbohydrate high-fat diet
Ketogenic diets
Low-density lipoprotein cholesterol
Modified Atkins diet
Minimally clinically important difference
Nonalcoholic fatty liver disease
Randomized clinical trials
Respiratory exchange ratio
Systolic blood pressure
Type 2 diabetes mellitus
Total energy intake
Very low-calorie ketogenic diet
Maximal oxygen consumption
Bueno NB, de Melo IS, de Oliveira SL, da Rocha Ataide T. Very-low-carbohydrate ketogenic diet v. low-fat diet for long-term weight loss: a meta-analysis of randomised controlled trials. Br J Nutr. 2013;110(7):1178–87.
Article CAS PubMed Google Scholar
Choi YJ, Jeon SM, Shin S. Impact of a ketogenic diet on metabolic parameters in patients with obesity or overweight and with or without type 2 diabetes: a meta-analysis of randomized controlled trials. Nutrients. 2020;12(7):2005.
Article CAS PubMed PubMed Central Google Scholar
Kirkpatrick CF, Bolick JP, Kris-Etherton PM, Sikand G, Aspry KE, Soffer DE, et al. Review of current evidence and clinical recommendations on the effects of low-carbohydrate and very-low-carbohydrate (including ketogenic) diets for the management of body weight and other cardiometabolic risk factors: a scientific statement from the National Lipid Association Nutrition and Lifestyle Task Force. J Clin Lipidol. 2019;13(5):689-711.e1.
Article PubMed Google Scholar
Dahlin M, Singleton SS, David JA, Basuchoudhary A, Wickström R, Mazumder R, et al. Higher levels of Bifidobacteria and tumor necrosis factor in children with drug-resistant epilepsy are associated with anti-seizure response to the ketogenic diet. eBioMedicine. 2022;80:104061.
Crosby L, Davis B, Joshi S, Jardine M, Paul J, Neola M, et al. Ketogenic diets and chronic disease: weighing the benefits against the risks. Front Nutr. 2021;8:702802.
Article PubMed PubMed Central Google Scholar
Paoli A, Rubini A, Volek JS, Grimaldi KA. Beyond weight loss: a review of the therapeutic uses of very-low-carbohydrate (ketogenic) diets. Eur J Clin Nutr. 2013;67(8):789–96.
Gershuni VM, Yan SL, Medici V. Nutritional ketosis for weight management and reversal of metabolic syndrome. Curr Nutr Rep. 2018;7(3):97–106.
Zhu H, Bi D, Zhang Y, Kong C, Du J, Wu X, et al. Ketogenic diet for human diseases: the underlying mechanisms and potential for clinical implementations. Signal Transduct Target Ther. 2022;7(1):11.
Lefevre F, Aronson N. Ketogenic diet for the treatment of refractory epilepsy in children: a systematic review of efficacy. Pediatrics. 2000;105(4):E46.
Kossoff EH, Zupec-Kania BA, Auvin S, Ballaban-Gil KR, Christina Bergqvist AG, Blackford R, et al. Optimal clinical management of children receiving dietary therapies for epilepsy: Updated recommendations of the International Ketogenic Diet Study Group. Epilepsia Open. 2018;3(2):175–92.
Bough KJ, Rho JM. Anticonvulsant mechanisms of the ketogenic diet. Epilepsia. 2007;48(1):43–58.
Yudkoff M, Daikhin Y, Melø TM, Nissim I, Sonnewald U, Nissim I. The ketogenic diet and brain metabolism of amino acids: relationship to the anticonvulsant effect. Annu Rev Nutr. 2007;27:415–30.
Yuan X, Wang J, Yang S, Gao M, Cao L, Li X, et al. Effect of the ketogenic diet on glycemic control, insulin resistance, and lipid metabolism in patients with T2DM: a systematic review and meta-analysis. Nutr Diabetes. 2020;10(1):38.
Goldenberg JZ, Day A, Brinkworth GD, Sato J, Yamada S, Jönsson T, et al. Efficacy and safety of low and very low carbohydrate diets for type 2 diabetes remission: systematic review and meta-analysis of published and unpublished randomized trial data. BMJ. 2021;372:m4743.
Castellana M, Conte E, Cignarelli A, Perrini S, Giustina A, Giovanella L, et al. Efficacy and safety of very low calorie ketogenic diet (VLCKD) in patients with overweight and obesity: a systematic review and meta-analysis. Rev Endocr Metab Disord. 2020;21(1):5–16.
Sourbron J, Klinkenberg S, van Kuijk SMJ, Lagae L, Lambrechts D, Braakman HMH, et al. Ketogenic diet for the treatment of pediatric epilepsy: review and meta-analysis. Childs Nerv Syst. 2020;36(6):1099–109.
Lee HS, Lee J. Influences of ketogenic diet on body fat percentage, respiratory exchange rate, and total cholesterol in athletes: a systematic review and meta-analysis. Int J Environ Res Public Health. 2021;18(6):2912.
Page MJ, McKenzie JE, Bossuyt PM, Boutron I, Hoffmann TC, Mulrow CD, et al. The PRISMA 2020 statement: an updated guideline for reporting systematic reviews. BMJ. 2021;372:n71.
Dragioti E, Solmi M, Favaro A, Fusar-Poli P, Dazzan P, Thompson T, et al. Association of antidepressant use with adverse health outcomes: a systematic umbrella review. JAMA Psychiat. 2019;76(12):1241–55.
Article Google Scholar
Patikorn C, Roubal K, Veettil SK, Chandran V, Pham T, Lee YY, et al. Intermittent Fasting and Obesity-Related Health Outcomes: An Umbrella Review of Meta-analyses of Randomized Clinical Trials. JAMA Netw Open. 2021;4(12):e2139558-e.
Theodoratou E, Tzoulaki I, Zgaga L, Ioannidis JP. Vitamin D and multiple health outcomes: umbrella review of systematic reviews and meta-analyses of observational studies and randomised trials. BMJ. 2014;348: g2035.
Shea BJ, Reeves BC, Wells G, Thuku M, Hamel C, Moran J, et al. AMSTAR 2: a critical appraisal tool for systematic reviews that include randomised or non-randomised studies of healthcare interventions, or both. BMJ. 2017;358:j4008.
DerSimonian R, Laird N. Meta-analysis in clinical trials. Control Clin Trials. 1986;7(3):177–88.
Higgins JP, Thompson SG, Spiegelhalter DJ. A re-evaluation of random-effects meta-analysis. J R Stat Soc Ser A Stat Soc. 2009;172(1):137–59.
Egger M, Davey Smith G, Schneider M, Minder C. Bias in meta-analysis detected by a simple, graphical test. BMJ. 1997;315(7109):629–34.
Heymsfield SB, Gonzalez MC, Shen W, Redman L, Thomas D. Weight loss composition is one-fourth fat-free mass: a critical review and critique of this widely cited rule. Obes Rev. 2014;15(4):310–21.
Mulligan AA, Lentjes MA, Luben RN, Wareham NJ, Khaw K-T. Changes in waist circumference and risk of all-cause and CVD mortality: results from the European Prospective Investigation into Cancer in Norfolk (EPIC-Norfolk) cohort study. BMC Cardiovasc Disord. 2019;19(1):1–15.
Jayedi A, Khan TA, Aune D, Emadi A, Shab-Bidar S. Body fat and risk of all-cause mortality: a systematic review and dose-response meta-analysis of prospective cohort studies. Int J Obes. 2022;46(9):1573–81.
Article CAS Google Scholar
Keller HH, Østbye T. Body mass index (BMI), BMI change and mortality in community-dwelling seniors without dementia. J Nutr Health Aging. 2005;9(5):316–20.
CAS PubMed Google Scholar
Kelley GA, Kelley KS, Stauffer BL. Walking and resting blood pressure: an inter-individual response difference meta-analysis of randomized controlled trials. Sci Prog. 2022;105(2):00368504221101636.
Langendam MW, Akl EA, Dahm P, Glasziou P, Guyatt G, Schünemann HJ. Assessing and presenting summaries of evidence in cochrane reviews. Syst Rev. 2013;2(1):81.
Dechartres A, Altman DG, Trinquart L, Boutron I, Ravaud P. Association between analytic strategy and estimates of treatment outcomes in meta-analyses. JAMA. 2014;312(6):623–30.
Sterne JA, Savović J, Page MJ, Elbers RG, Blencowe NS, Boutron I, et al. RoB 2: a revised tool for assessing risk of bias in randomised trials. BMJ. 2019;366:l4898.
Sainsbury E, Kizirian NV, Partridge SR, Gill T, Colagiuri S, Gibson AA. Effect of dietary carbohydrate restriction on glycemic control in adults with diabetes: a systematic review and meta-analysis. Diabetes Res Clin Pract. 2018;139:239–52.
Rafiullah M, Musambil M, David SK. Effect of a very low-carbohydrate ketogenic diet vs recommended diets in patients with type 2 diabetes: a meta-analysis. Nutr Rev. 2022;80(3):488–502.
Alarim RA, Alasmre FA, Alotaibi HA, Alshehri MA, Hussain SA. Effects of the ketogenic diet on glycemic control in diabetic patients: meta-analysis of clinical trials. Cureus. 2020;12(10):e10796.
PubMed PubMed Central Google Scholar
Amini MR, Aminianfar A, Naghshi S, Larijani B, Esmaillzadeh A. The effect of ketogenic diet on body composition and anthropometric measures: A systematic review and meta-analysis of randomized controlled trials. Crit Rev Food Sci Nutr. 2022;62(13):3644–57.
Ashtary-Larky D, Bagheri R, Asbaghi O, Tinsley GM, Kooti W, Abbasnezhad A, et al. Effects of resistance training combined with a ketogenic diet on body composition: a systematic review and meta-analysis. Crit Rev Food Sci Nutr. 2022;62(21):5717–32.
Cao J, Lei S, Wang X, Cheng S. The effect of a ketogenic low-carbohydrate, high-fat diet on aerobic capacity and exercise performance in endurance athletes: a systematic review and meta-analysis. Nutrients. 2021;13(8):2896.
Lee HS, Lee J. Effects of combined exercise and low carbohydrate ketogenic diet interventions on waist circumference and triglycerides in overweight and obese individuals: a systematic review and meta-analysis. Int J Environ Res Public Health. 2021;18(2):828.
López-Espinoza M, Chacón-Moscoso S, Sanduvete-Chaves S, Ortega-Maureira MJ, Barrientos-Bravo T. Effect of a ketogenic diet on the nutritional parameters of obese patients: a systematic review and meta-analysis. Nutrients. 2021;13(9):2946.
Muscogiuri G, El Ghoch M, Colao A, Hassapidou M, Yumuk V, Busetto L. European guidelines for obesity management in adults with a very low-calorie ketogenic diet: a systematic review and meta-analysis. Obes Facts. 2021;14(2):222–45.
Smith ES, Smith HA, Betts JA, Gonzalez JT, Atkinson G. A Systematic review and meta-analysis comparing heterogeneity in body mass responses between low-carbohydrate and low-fat diets. Obesity. 2020;28(10):1833–42.
Yang YF, Mattamel PB, Joseph T, Huang J, Chen Q, Akinwunmi BO, et al. Efficacy of low-carbohydrate ketogenic diet as an adjuvant cancer therapy: a systematic review and meta-analysis of randomized controlled trials. Nutrients. 2021;13(5):1388.
Vargas-Molina S, Gómez-Urquiza JL, García-Romero J, Benítez-Porres J. Effects of the ketogenic diet on muscle hypertrophy in resistance-trained men and women: a systematic review and meta-analysis. Int J Environ Res Public Health. 2022;19(19):12629.
El-Rashidy OF, Nassar MF, Abdel-Hamid IA, Shatla RH, Abdel-Hamid MH, Gabr SS, et al. Modified Atkins diet vs classic ketogenic formula in intractable epilepsy. Acta Neurol Scand. 2013;128(6):402–8.
Kossoff EH, McGrogan JR, Bluml RM, Pillas DJ, Rubenstein JE, Vining EP. A modified Atkins diet is effective for the treatment of intractable pediatric epilepsy. Epilepsia. 2006;47(2):421–4.
Bough KJ, Wetherington J, Hassel B, Pare JF, Gawryluk JW, Greene JG, et al. Mitochondrial biogenesis in the anticonvulsant mechanism of the ketogenic diet. Ann Neurol. 2006;60(2):223–35.
Kim DY, Simeone KA, Simeone TA, Pandya JD, Wilke JC, Ahn Y, et al. Ketone bodies mediate antiseizure effects through mitochondrial permeability transition. Ann Neurol. 2015;78(1):77–87.
Garriga-Canut M, Schoenike B, Qazi R, Bergendahl K, Daley TJ, Pfender RM, et al. 2-Deoxy-D-glucose reduces epilepsy progression by NRSF-CtBP–dependent metabolic regulation of chromatin structure. Nat Neurosci. 2006;9(11):1382–7.
Wang ZJ, Bergqvist C, Hunter JV, Jin D, Wang DJ, Wehrli S, et al. In vivo measurement of brain metabolites using two-dimensional double-quantum MR spectroscopy—exploration of GABA levels in a ketogenic diet. Magn Reson Med. 2003;49(4):615–9.
Olson CA, Vuong HE, Yano JM, Liang QY, Nusbaum DJ, Hsiao EY. The gut microbiota mediates the anti-seizure effects of the ketogenic diet. Cell. 2018;173(7):1728-41.e13.
Dupuis N, Curatolo N, Benoist JF, Auvin S. Ketogenic diet exhibits anti-inflammatory properties. Epilepsia. 2015;56(7):e95–8.
Shimazu T, Hirschey MD, Newman J, He W, Shirakawa K, Le Moan N, et al. Suppression of oxidative stress by β-hydroxybutyrate, an endogenous histone deacetylase inhibitor. Science. 2013;339(6116):211–4.
O’Neill B, Raggi P. The ketogenic diet: Pros and cons. Atherosclerosis. 2020;292:119–26.
Freire R. Scientific evidence of diets for weight loss: different macronutrient composition, intermittent fasting, and popular diets. Nutrition. 2020;69: 110549.
Becker A, Gaballa D, Roslin M, Gianos E, Kane J. Novel nutritional and dietary approaches to weight loss for the prevention of cardiovascular disease: ketogenic diet, intermittent fasting, and bariatric surgery. Curr Cardiol Rep. 2021;23(7):1–9.
Ashtary-Larky D, Bagheri R, Bavi H, Baker JS, Moro T, Mancin L, et al. Ketogenic diets, physical activity and body composition: a review. Br J Nutr. 2022;127(12):1898–920.
Thomsen HH, Rittig N, Johannsen M, Møller AB, Jørgensen JO, Jessen N, et al. Effects of 3-hydroxybutyrate and free fatty acids on muscle protein kinetics and signaling during LPS-induced inflammation in humans: anticatabolic impact of ketone bodies. Am J Clin Nutr. 2018;108(4):857–67.
Koutnik AP, D’Agostino DP, Egan B. Anticatabolic effects of ketone bodies in skeletal muscle. Trends Endocrinol Metab. 2019;30(4):227–9.
Parker BA, Walton CM, Carr ST, Andrus JL, Cheung EC, Duplisea MJ, et al. β-hydroxybutyrate elicits favorable mitochondrial changes in skeletal muscle. Int J Mol Sci. 2018;19(8):2247.
Huang Z, Huang L, Waters MJ, Chen C. Insulin and growth hormone balance: implications for obesity. Trends Endocrinol Metab. 2020;31(9):642–54.
Møller N, Copeland KC, Nair KS. Growth hormone effects on protein metabolism. Endocrinol Metab Clin North Am. 2007;36(1):89–100.
Parks EJ. Effect of dietary carbohydrate on triglyceride metabolism in humans. J Nutr. 2001;131(10):2772S-S2774.
Lamarche B, Tchernof A, Moorjani S, Cantin B, Dagenais GR, Lupien PJ, et al. Small, dense low-density lipoprotein particles as a predictor of the risk of ischemic heart disease in men: prospective results from the Qué bec Cardiovascular Study. Circulation. 1997;95(1):69–75.
Dreon DM, Fernstrom HA, Campos H, Blanche P, Williams PT, Krauss RM. Change in dietary saturated fat intake is correlated with change in mass of large low-density-lipoprotein particles in men. Am J Clin Nutr. 1998;67(5):828–36.
Gardner CD, Kiazand A, Alhassan S, Kim S, Stafford RS, Balise RR, et al. Comparison of the Atkins, Zone, Ornish, and LEARN diets for change in weight and related risk factors among overweight premenopausal women: the A TO Z weight loss study: a randomized trial. JAMA. 2007;297(9):969–77.
Shai I, Schwarzfuchs D, Henkin Y, Shahar DR, Witkow S, Greenberg I, et al. Weight loss with a low-carbohydrate, Mediterranean, or low-fat diet. N Engl J Med. 2008;359(3):229–41.
Download references
Acknowledgements
The authors would like to acknowledge Thunchanok Ingkaprasert and Wachiravit Youngjanin for their editorial assistance.
No funding was obtained for the conduct of this study.
Author information
Authors and affiliations.
Department of Pharmacotherapy, College of Pharmacy, University of Utah, 30 2000 E, Salt Lake City, Utah, 84112, USA
Chanthawat Patikorn, Pantakarn Saidoung, Sajesh K. Veettil & Nathorn Chaiyakunapruk
Department of Social and Administrative Pharmacy, Faculty of Pharmaceutical Sciences, Chulalongkorn University, Bangkok, Thailand
Chanthawat Patikorn
Division of Gastroenterology, Hepatology & Nutrition, Department of Internal Medicine, University of Utah, Salt Lake City, Utah, USA
Division of Ambulatory Medicine, Department of Medicine, Faculty of Medicine Siriraj Hospital, Mahidol University, Bangkok, Thailand
Pochamana Phisalprapa
School of Medical Sciences, Universiti Sains Malaysia, Kota Bharu, Malaysia
Yeong Yeh Lee
Department of Kinesiology and Nutrition, University of Illinois at Chicago, Chicago, Illinois, USA
Krista A. Varady
IDEAS Center, Veterans Affairs Salt Lake City Healthcare System, Salt Lake City, Utah, USA
Nathorn Chaiyakunapruk
You can also search for this author in PubMed Google Scholar
Contributions
CP, PS, SKV, and NC conceived and designed the study protocol. CP, PS, and SKV performed a literature review and data analysis. CP, PS, TP, PP, YYL, KAV, SKV, and NC interpreted the study findings. CP and PS were major contributors to writing the manuscript. All authors read and approved the final manuscript.
Corresponding authors
Correspondence to Sajesh K. Veettil or Nathorn Chaiyakunapruk .
Ethics declarations
Ethics approval and consent to participate.
Not applicable.
Consent for publication
Competing interests.
The authors declare that they have no competing interests.
Additional information
Publisher’s note.
Springer Nature remains neutral with regard to jurisdictional claims in published maps and institutional affiliations.
Supplementary Information
Additional file 1..
PRISMA 2020 Main Checklist.
Additional file 2:
Method S1. Data extraction. Table S1. Difference from original review protocol. Table S2. Search strategy. Table S3. Excluded studies with reasons. Table S4. Quality assessment. Table S5. Summary of associations. Table S6. Sensitivity analyses.
Rights and permissions
Open Access This article is licensed under a Creative Commons Attribution 4.0 International License, which permits use, sharing, adaptation, distribution and reproduction in any medium or format, as long as you give appropriate credit to the original author(s) and the source, provide a link to the Creative Commons licence, and indicate if changes were made. The images or other third party material in this article are included in the article's Creative Commons licence, unless indicated otherwise in a credit line to the material. If material is not included in the article's Creative Commons licence and your intended use is not permitted by statutory regulation or exceeds the permitted use, you will need to obtain permission directly from the copyright holder. To view a copy of this licence, visit http://creativecommons.org/licenses/by/4.0/ . The Creative Commons Public Domain Dedication waiver ( http://creativecommons.org/publicdomain/zero/1.0/ ) applies to the data made available in this article, unless otherwise stated in a credit line to the data.
Reprints and permissions
About this article
Cite this article.
Patikorn, C., Saidoung, P., Pham, T. et al. Effects of ketogenic diet on health outcomes: an umbrella review of meta-analyses of randomized clinical trials. BMC Med 21 , 196 (2023). https://doi.org/10.1186/s12916-023-02874-y
Download citation
Received : 25 November 2022
Accepted : 19 April 2023
Published : 25 May 2023
DOI : https://doi.org/10.1186/s12916-023-02874-y
Share this article
Anyone you share the following link with will be able to read this content:
Sorry, a shareable link is not currently available for this article.
Provided by the Springer Nature SharedIt content-sharing initiative
- Ketegenic diet
- Umbrella review
- Systematic review
- Meta-analysis
- Weight loss
BMC Medicine
ISSN: 1741-7015
- Submission enquiries: [email protected]
- General enquiries: [email protected]
Thank you for visiting nature.com. You are using a browser version with limited support for CSS. To obtain the best experience, we recommend you use a more up to date browser (or turn off compatibility mode in Internet Explorer). In the meantime, to ensure continued support, we are displaying the site without styles and JavaScript.
- View all journals
- My Account Login
- Explore content
- About the journal
- Publish with us
- Sign up for alerts
- Review Article
- Open access
- Published: 17 January 2022
Ketogenic diet for human diseases: the underlying mechanisms and potential for clinical implementations
- Huiyuan Zhu 1 na1 ,
- Dexi Bi 1 na1 ,
- Youhua Zhang 1 na1 ,
- Cheng Kong 2 , 3 na1 ,
- Jiahao Du 2 ,
- Xiawei Wu 2 , 4 ,
- Qing Wei 1 &
- Huanlong Qin 2 , 3
Signal Transduction and Targeted Therapy volume 7 , Article number: 11 ( 2022 ) Cite this article
70k Accesses
101 Citations
572 Altmetric
Metrics details
- Health policy
- Metabolic disorders
The ketogenic diet (KD) is a high-fat, adequate-protein, and very-low-carbohydrate diet regimen that mimics the metabolism of the fasting state to induce the production of ketone bodies. The KD has long been established as a remarkably successful dietary approach for the treatment of intractable epilepsy and has increasingly garnered research attention rapidly in the past decade, subject to emerging evidence of the promising therapeutic potential of the KD for various diseases, besides epilepsy, from obesity to malignancies. In this review, we summarize the experimental and/or clinical evidence of the efficacy and safety of the KD in different diseases, and discuss the possible mechanisms of action based on recent advances in understanding the influence of the KD at the cellular and molecular levels. We emphasize that the KD may function through multiple mechanisms, which remain to be further elucidated. The challenges and future directions for the clinical implementation of the KD in the treatment of a spectrum of diseases have been discussed. We suggest that, with encouraging evidence of therapeutic effects and increasing insights into the mechanisms of action, randomized controlled trials should be conducted to elucidate a foundation for the clinical use of the KD.
Similar content being viewed by others
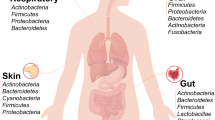
Microbiota in health and diseases
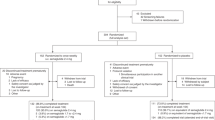
Two-year effects of semaglutide in adults with overweight or obesity: the STEP 5 trial
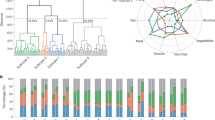
Associations of dietary patterns with brain health from behavioral, neuroimaging, biochemical and genetic analyses
Introduction.
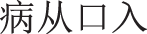
The KD comprises a high-fat component, very low carbohydrates, and adequate proteins (Fig. 1 ), 5 , 6 , 7 and has been clinically used since the early 1920s to control seizures in patients with epilepsy, especially those who do not respond adequately to antiepileptic medication. 7 , 8 , 9 The history of dietary interventions used as “cures” for epilepsy possibly dates back to 500 before christ, whereas fasting has been recognized as an effective therapy against epilepsy and has even been recorded in the Hippocratic collection. 8 Modern implementation of fasting as an antiepileptic treatment began in 1911, 8 when it was noted that a diet containing few carbohydrates but a high proportion of fat could produce acetone and beta-hydroxybutyric acid (β-HB), similar to what is seen with starvation, 10 and that alternative ketonemia-producing approaches might achieve effects similar to that of fasting. 5 In 1921, Russel Wilder first proposed that a ketone-producing diet could be as effective as fasting for the treatment of epilepsy, and coined the term “ketogenic diet”. 8 In particular, the KD can mimic the metabolic effects of fasting without significant calorie deprivation. The KD enjoyed wide popularity as a medical approach for treating epilepsy for nearly a decade before the introduction of antiepileptic agents, such as diphenylhydantoin. 8 The KD re-emerged in the 1990s and became well established as an option for drug-resistant epilepsy. 8 , 9 , 11 , 12 In the past few decades (Fig. 1 ), the KD has received extensive interest because of its beneficial effects in a number of diseases, such as neurological disorders, obesity, type 2 diabetes mellitus (T2DM), cancer, intestinal disorders, and respiratory compromise. 13 , 14 , 15 , 16 , 17 , 18 , 19 , 20 , 21 , 22 , 23 Here, we provide a comprehensive review of the KD, covering the therapeutic effects, relevant mechanisms, and clinical evidence underlying the implementation of the KD in various diseases.
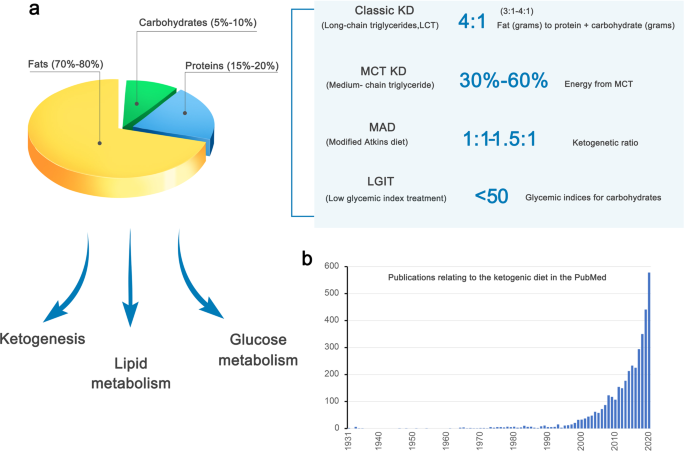
The composition and metabolic effects of the ketogenic diet, which have increasingly generated interest. a The compositional features of the classic KD and its variants are shown. b The number of publications obtained for the search term “ketogenic diet” in PubMed is shown by the year of publication. Articles published before 1931 were not included due to the unavailability of PubMed records predating this timepoint
Types of the ketogenic diet
The KD is characterized as a high-fat, very-low-carbohydrate diet. Several variant KD that show similar efficacy to that of the original form has been developed to date, and offer flexibility to increase compliance with the regimens. 24 , 25 There are four major types of the KD with proven efficacy: the classic long-chain triglyceride (LCT) KD, medium-chain triglyceride (MCT) KD, modified Atkins diet (MAD), and low glycemic index treatment (Fig. 1 ). 24
The classic LCT KD is the most traditional type of the KD, is widely used in the clinical setting, and incorporates a 4:1 ratio of fat (in grams) to protein plus carbohydrate (in grams). 26 , 27 Fat provides 90% calories, and its predominant source is food-derived LCT, and a 3:1 or lower ratio may be used. 24 Moreover, the low ratios are appropriate for the KD initiation in infants, whereas in older children, initiation with a 4:1 ratio, followed by a reduced ratio may be more effective. 24 , 28 Furthermore, there is evidence that calorie and fluid restriction is unnecessary as no beneficial effect was proved with these two factors. 24 , 29
Due to the severe carbohydrate restriction, the LCT KD is unpalatable, difficult to prepare, and, therefore, difficult to maintain. 30 In 1971, the MCT (C6-C12) KD was devised. 30 The dietary use of MCT oil is more acceptable and is more ketogenic than LCTs. 30 , 31 , 32 , 33 The MCT KD has better flexibility in diet ratios than the LCT KD, and the calorie intake is calculated based on the percentage of energy derived from MCT. 24 , 31 In addition, there is clinical evidence of the equivalent efficacy of the MCT and LCT KD. 12 , 32 However, the MCT KD is frequently associated with gastrointestinal side effects. 24 , 31
The MAD is based on the Atkins diet, which was popularly used in weight loss 34 , 35 , 36 and shares similar food choices with the classic KD, but without the need for precise weighing of ingredients. The MAD does not have a strict ketogenic ratio, which typically ranges from 1:1 to 1.5:1 and, sometimes, can reach 4:1. 35 Moreover, the MAD does not include protein, fluid, or calorie restrictions. Carbohydrate intake in the MAD is restricted to 10–15 g/day in the first month and can be subsequently increased to 20 g/day. 37 , 38 There is clinical evidence supporting the efficacy of the MAD in children with intractable epilepsy. 37 , 38 , 39 , 40 , 41 , 42 , 43 , 44
The low glycemic index treatment is based on the concept that the protective effect of the KD relies on stable glucose levels, 45 but has a liberalized regimen with low-carbohydrate composition to minimize glycemic increases (glycemic indices <50), 45 and is an effective antiepileptic intervention in children with intractable epilepsy. 44 , 45 , 46 , 47 , 48 , 49
Despite the abovementioned evidence that suggests the similar efficacy of the four types of KD, it is unclear whether the mechanisms of action of these diets differ.
The impact of the ketogenic diet on metabolism
Lipid metabolism.
The metabolism of blood lipids during KD is often a concern. In the presence of oxygen, most cellular energy originates, through glycolysis, from glucose-metabolized pyruvate, which then undergoes oxidative phosphorylation within mitochondria. In the absence of glucose, cellular energy is produced by the degradation of fatty acids. 50 A low-carbohydrate, high-protein, and high-fat diet can be unhealthy as it may lead to an increase in the circulating low-density lipoprotein (LDL), cholesterol, and triglyceride (TG) concentrations. As for liver fat metabolism, from the perspective of diet metabolism, a low total and saturated fat/high-carbohydrate diet can effectively manage liver fat storage by limiting exogenous fats. 51 However, the KD has potential health benefits with regard to these cardiovascular risk factors, and recent animal and clinical studies provided ample evidence that cutting carbs can actually lower total cholesterol, increase high-density lipoprotein (HDL), and reduce blood TG levels. 52 , 53 With the premise of ensuring constant total calorie intake, the KD reduces carbohydrate intake, lowers serum insulin levels, increases insulin sensitivity, and enhances fat catabolism, thus reducing blood lipids. 14 Due to increased de novo lipogenesis and decreased fatty acid oxidation and/or ketone production, higher carbohydrate intake may be detrimental to the net loss of liver fat. In contrast, low-carbohydrate/high-fat KD significantly increases the rate of whole-body fatty acid oxidation and liver ketogenesis. 54 , 55 Therefore, KD has been shown to reduce liver fat. 56 , 57 Moreover, the KD induces the expression of fibroblast growth factor-1 and promotes the hepatic clearance of TGs. 58 In addition, the KD can increase the size and volume of LDL-C particles, 59 which is believed to reduce the risk of cardiovascular disease, as smaller LDL particles have higher atherogenic activity. Furthermore, the KD affects endogenous cholesterol synthesis. β-Hydroxy β-methylglutaryl-CoA reductase, a key enzyme in cholesterol biosynthesis, is activated by insulin. Therefore, increased blood glucose concentrations and higher insulin levels lead to increased endogenous cholesterol synthesis. Thus, reducing dietary carbohydrates and proper cholesterol intake will lead to the inhibition of cholesterol biosynthesis.
Glucose metabolism
There are two sources of glucose in humans: glycogenic amino acids and glycerol that are released by TG lysis. 60 , 61 The importance of the latter source increases during ketosis. In the first few days of the KD, glycogenesis from amino acids is the main source of glucose. Subsequently, the contribution of amino acids is reduced, whereas the amount of glucose obtained from glycerol increases. In fact, TG-hydrolysis-induced glycerol can generate more than 16% glucose in the liver during the KD, compared to 60% glucose after several days of complete fasting. 62 The effect of the KD on blood sugar levels remains controversial. After fasting for several days or restricting carbohydrate intake, the glucose reserves in the body are insufficient to produce oxaloacetate in the Krebs cycle for normal fat oxidation and supply of glucose to the central nervous system. 63 Thus, most studies believe that the KD leads to decreased blood sugar concentration and a lower insulin-to-glucagon ratio, which is beneficial for glycemic control in individuals with diabetes. 20 , 64 Elevated glucagon levels are associated with hepatic glucose mobilization. A recent study analyzed the effects of KDs in exercising and sedentary rats. 65 After 6 weeks, KD decreased insulin levels by 80%, blood sugar by 50%, TGs by 55%, and cholesterol by 20%, compared to the standard feed, whereas exercising did not bring benefits. Furthermore, a 5-year prospective study that included a total of 27,799 men and 36,875 women in Japan showed that LCDs are significantly associated with a reduced risk of type 2 diabetes in women, whereas high-fat and high-protein diets are protective factors against diabetes in Japanese women. 66 However, Delahanty et al. arrived at the opposite conclusion. Independent of exercise and body mass index, patients with type 1 diabetes who consume high fat and LCDs have higher glycosylated hemoglobin and poorer blood sugar control. 67 Some animal experiments have shown that glucose tolerance decreases in mice that are fed KD for 22 weeks. 68 The KD did not prevent the decline in β-cell function, nor did it improve insulin secretion. Therefore, individual differences and treatment conditions should be considered in the clinical application of the KD.
Ketogenic process
In the liver, excessive production of acetyl coenzyme A (acetyl-CoA) and oxidation of fatty acids leads to the production of Ketone Bodies (KBs). 69 The acetyl-CoA molecule can be utilized in the Krebs cycle or to produce acetoacetate, which is then spontaneously converted to acetone or 3-β-hydroxybutyrate by 3-β-hydroxybutyrate dehydrogenase. 70 , 71 The KBs then enter the bloodstream and can be utilized by the brain, heart, and muscle, where they produce cellular energy in mitochondria. 7 , 72 , 73 Higher circulating KB levels lead to ketonemia and ketonuria. 74 Under physiological conditions, the blood concentration of KBs during prolonged fasting usually is 5–7 mM, while the glucose concentration could be lowered to below 1 mM without either convulsions or any impairment of cognitive function. 75 In diabetic ketoacidosis, the plasma KB levels can increase up to 25 mM due to insulin deficiency, with a consequent increase in the plasma glucose concentration and decreased blood pH. 74 The KBs constitute a more efficient energy source than glucose, metabolize faster than glucose, and can bypass the glycolytic pathway by directly entering the Krebs cycle, whereas glucose needs to undergo glycolysis. 76 Moreover, KBs cause fatty acid-mediated activation of peroxisome proliferator-activated receptor α as well as the inhibition of glycolysis and fatty acids. 77 Therefore, KBs reduce the production of glycolytic adenosine triphosphate (ATP) and increase mitochondrial oxidation-induced ATP generation, 71 thereby promoting mitochondrial oxidative metabolism, with resultant beneficial downstream metabolic changes.
Ketogenic diet and gut microbiota
The effects of the KD on the gut microbiome have been reported in many murine and human studies (Table 1 ). Mice that were fed a 4-day KD showed significant changes in gut bacterial composition, which was characterized by an increase in Akkermansia and Parabacteriodes populations that induced an anti-seizure effect in germ-free or antibiotic-treated mice. 78 The increased gut populations of these two bacterial genera decrease the γ-glutamyl transpeptidase level, which catalyzes the transfer of functional groups of γ-glutamyl from glutathione to an amino acid acceptor that may produce glutamate. 79 In addition, ketogenic γ-glutatamylated amino acids decreased in the gut and in the blood, which supports the key anti-seizure effects of KD-associated microbiota. 78 In the human gut, the post-KD production of KB by the host can partially drive gut microbial shifts, which reduces the number of intestinal Th17 cells. 19 Similarly, using a murine model, Kong et al. demonstrated that an increase in Akkermansia muciniphila, Lactobacillus , and Roseburia following a KD plays a potential anti-colitis effect. 20 The potential protective effects on intestinal barrier function may be related to the production of RORγt + CD3 - group 3 innate lymphoid cells and related inflammatory cytokines (IL-17α, IL-18, IL-22, CCL-4). 20 Another study of a 16-week KD revealed beneficial effects of the ketogenic-induced microbiota, including improved neurovascular functions in mice and reduced risk of Alzheimer’s disease. 80 These beneficial effects may be related to changes in the gut microbiota composition, including an increase in the beneficial bacteria Akkermansia muciniphila and Lactobacillus , which produce short-chain fatty acids. Interestingly, Ma et al. also found a decrease in the numbers of pro-inflammatory microbes, such as Desulfovibrio and Turicibacter . Furthermore, the KD improves the gut microbiome in a murine model of autism, 81 and Newell et al. observed an overall reduction in the microbial richness of the cecum and feces and an increased ratio of Firmicutes and Bacteroides after the administration of the KD. As carbohydrates are the basic blocks that the microbes break down to produce energy, the lower carbohydrate content in the KD results in a decline in overall microbial diversity. 82 Furthermore, in treatment-refractory epilepsy, the KD significantly reduced the abundance of pathogenic proteobacteria ( Escherichia , Salmonella , and Vibrio ), whereas Bacteroidetes populations increased. 83 Notably, Bacteroidetes are closely involved in the digestion and metabolism of high-fat nutrients, regulation of interleukin secretion in dendritic cells, and are associated with seizure effects in epileptic patients. 84 Another study found differences in the gut microbiota between responders (reduced seizure frequency or seizure cessation) and non-responders (no effect on seizure) among children who received a KD and noted that an increase in Bacteroides and a decrease in Firmicutes and Actinomycetes populations in the responders. 85 On the other hand, populations of Clostridia , Ruminococcus , and Lachnospiraceae ( Firmicutes phylum) increased in non-responders. These data suggest that the KD-induced gut microbiota changes should be considered as a potential biomarker for the efficacy of antiepileptic therapy. Moreover, an updated study showed that KD potentiates cognitive impairment induced by intermittent hypoxia in mice and increases the risk-associated Bilophila wadsworthia. 86 Inhibiting Th1 cell development abrogates the adverse effects of both B. wadsworthia and environmental risk factors on cognitive impairment. 86 Taken together, these findings identify the potential select gut bacteria that contribute to KD effects on target site in mice and humans.
In the potential physiological application of the ketogenic diet, some studies have found that KD could extend longevity and reduce midlife mortality in the mouse model. 87 , 88 In fact, the mechanism of how KD works in our body from the intestine to the target site is still controversial. Based on the gut microbiota, the ketone body itself can selectively inhibit the growth of bifidobacteria , thereby reducing the level of intestinal pro-inflammatory Th17 cells. 19 The ketone bodies are also involved in multiple metabolic pathways, and protective effects of ketone bodies may lead to improvement in health status and delay both aging and the development of related diseases through improving mitochondrial function, antioxidant and anti-inflammatory effects, histone and non-histone acetylation, β-hydroxybutyrylation of histones, modulation of neurotransmitter systems and RNA functions. 89 Thus, the accumulation of ketone bodies can at least partly explain the influence of the gut microbiota by KD, 20 which thereby inhibiting colitis, improving several diseases such as epilepsy. The summary of changes in metabolism and gut microbiota induced by the ketogenic diet is shown in Fig. 2 .
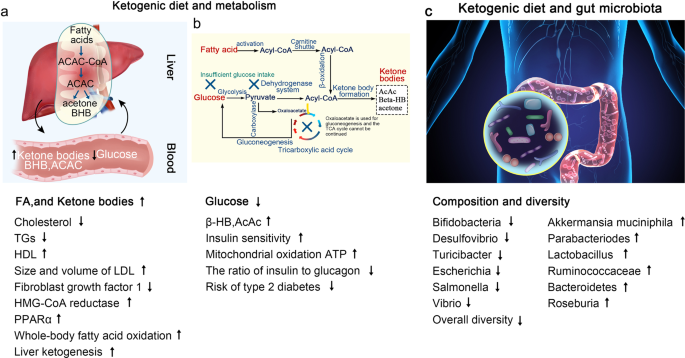
Summary of KD-induced changes in metabolism and gut microbiota. a , b The KD increases the levels of FA and KBs and decreases plasma glucose concentrations through different pathways. c The KD alters the composition and diversity of microbiota as follows: the increased abundance of Akkermansia muciniphila , Parabacteriodes , Lactobacillus , Ruminococcaceae , Bacteroidetes , and Roseburia , and reduced populations of Bifidobacteria , Desulfovibrio , Turicibacter , Escherichia , Salmonella , and Vibrio
Function in endocrine and metabolic disorders
Type 2 diabetes mellitus.
T2DM is characterized by chronic hyperglycemia with fasting plasma glucose concentrations ≥126 mg/dL and glycated hemoglobin (HbA1c) ≥6.5%. 90 As dietary carbohydrates are the major macronutrients that increase glycemic levels, 91 it is logical to reduce the dietary carbohydrate intake to treat T2DM. Researchers have found that carbohydrate restriction has the greatest effect on reducing postprandial and overall glucose concentrations and the HbA1c. 92 , 93 , 94 , 95 , 96 For example, in a study comparing the effect of a very-low-carbohydrate ketogenic diet (VLCKD) and a low-calorie diet on blood glucose levels in diabetic patients, the decrease in blood glucose concentrations was greater in the VLCKD group than in the low-calorie diet group for 24 weeks. More importantly, the blood glucose level of the VLCKD group was ~1 mM lower than that of the low-calorie diet group and reverted to a normal level after 24 weeks. However, the blood glucose level of the low-calorie group leveled out at 16 weeks and remained elevated thereafter. At 24 weeks, the HbA1c level in the VLCKD group decreased to 6.2%, compared to >7.5% in the low-calorie diet group. 96 Similarly, a meta-analysis revealed that VLCKD resulted in a significant decrease in HbA1c and weight loss after 3 months and after 6 months, however, it was not better after 12 months compared to a control diet. 97 VLCKD showed more beneficial effects on serum triglycerides and high-density lipoprotein cholesterol levels and reducing antidiabetic medications for up to 12 months. 97
Hyperglycemia is the most frequent characteristic of T2DM; however, the pathophysiology of T2DM involves insulin resistance and hyperinsulinemia. Therefore, reducing insulin levels should be a therapeutic target in the treatment of T2DM. The homeostatic model assessment of insulin resistance is an indicator for evaluating insulin resistance. The consumption of the KD decreased the homeostatic model assessment of insulin resistance in patients with T2DM from −0.4 to −3.4. 98 , 99 , 100 , 101 A systematic meta-analysis that included 13 studies showed that the KD not only guarantees the basic supply of nutrients but also maintains a negative balance of energy, thereby decreasing the fluctuation and reduction of insulin secretion caused by reduced carbohydrate intake as well, which eventually leads to increased insulin sensitivity. 102 Thus, KD improves glycemic control in T2DM patients by reducing glucose uptake and improving systemic insulin sensitivity (Fig. 3 ).
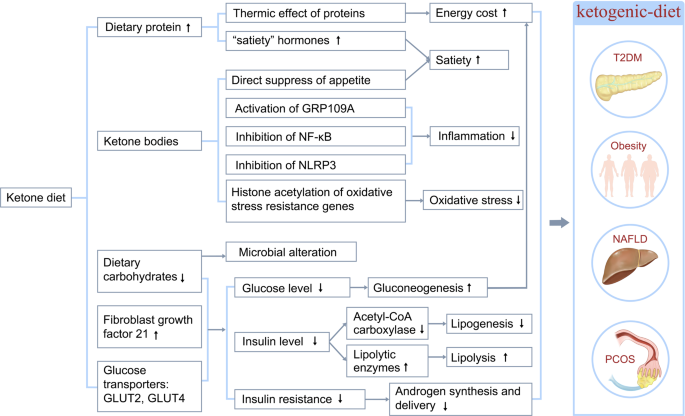
Possible mechanisms whereby the ketogenic diet ameliorates metabolic disorders. The mechanisms, through which the ketogenic diet ameliorates endocrine and metabolic disorders, including T2DM, obesity, NAFLD, and PCOS, are shown. Ketogenic diets exert therapeutic effects on metabolic disorders through various mechanisms, including reduction of plasma glucose, glycated hemoglobin levels, and serum insulin levels; improvement of insulin sensitivity; increased satiety; and decreased inflammation
The molecular mechanism underlying the KD-induced improvement of T2DM clinical outcomes has been investigated in both the system biology approach and mouse model studies. Using a cell network-analysis approach, researchers identified a strong correlation between insulin resistance and the main pathways of ketosis. Glucose transporter type 4, an effector protein of the insulin-resistance pathway, directly correlates with proteins, such as Hydroxyacy1-CoA dehydrogenase 1 and Acyl-coenzyme A oxidase 1, that are involved in the KD-induced pathways. 103 In ob/ob mice studies, several molecules are involved in the improvement of hyperglycemia and hyperinsulinemia during the KD. The expression of certain O-GlcNAc-modified proteins is altered when the KD improves hyperglycemia. 104 Fatty acid synthase and acetyl-CoA carboxylase 1, which are two key enzymes that are involved in hepatic lipogenesis, are present in regular diet fed-ob/ob mice but absent in the KD-fed mice. 105 KD administration decreased liver mRNA expression of Glucose transporter type 2 while increased that of Fibroblast growth factor 21 in diabetic mice. 106 Glucose transporter type 2 plays an important role in glucose induced-insulin secretion in pancreatic β cells 107 thus decreased Glucose transporter type 2 expression suggests a decreased insulin level and improved insulin resistance in T2DM. Fibroblast growth factor 21 is an important target gene of peroxisome proliferator-activated receptor α, which promotes lipid catabolism and improves insulin resistance. 108 Moreover, the β-HB could inhibit nuclear factor κB (NF-κB) signaling, 109 an upregulated inflammatory pathway associated with the pathogenesis of T2DM. 110
Caution should be exercised when prescribing the KD to T2DM patients on other drug treatments, such as sodium glucose cotransporter 2 (SGLT2) inhibitors and insulin. SGLT2 inhibitors, which confer cardiovascular benefits in T2DM patients, can also exert pro-ketogenic effects by mediating a metabolic switch from glucose to lipid utilization. Thus, T2DM patients who are already receiving SGLT2 inhibitors will have a significantly higher risk of developing euglycemic diabetic ketoacidosis if placed on the KD; therefore, the KD should not be prescribed to T2DM patients receiving SGLT2 inhibitors. 111 Carbohydrate restriction may increase the risk of hypoglycemia in patients receiving insulin and insulin secretagogues; thus, it is recommended that the drug dosage should be modified based on the goal of glycemic control and the type of antidiabetes therapy when prescribing the KD to T2DM patients. 112
With the increasing prevalence of obesity, the 21st century has witnessed the emergence of various diet programs, with the KD at the forefront, for promoting weight loss and enhancing physical performance. Many studies have demonstrated that the KD is a potentially promising diet for reducing obesity while maintaining the capacity for physical activity. A study conducted in 2016 showed that short-term KD followed by an almost carbohydrate-free diet effectively reduced body weight, waist circumference, blood pressure, and insulin resistance in clinically healthy morbidly obese adults with body mass index (BMI) ≥ 45 kg/m. 2 , 113 In a long-term study, the KD significantly decreased BMI, blood cholesterol, and plasma glucose, and increased weight loss, thereby reducing the risk factors for various obesity-associated chronic diseases, in obese hypercholesterolemic patients with BMI > 35 kg/m 2 without any side effects. 114 In a controlled study enrolling 20 participants who received the VLCKD, a significant improvement in biochemical parameters was observed after 8 weeks of KD adherence, which included a reduction in BMI, LDL-C, TGs, insulinemia, and liver transaminases.
In addition, KD has a more beneficial effect on obesity than other diets. In a meta-analysis of 11 studies, significant weight reductions were reported in the LCD group when compared to the low-fat diet group. Interestingly, the authors attributed this effect to a lower energy intake rather than the macronutrient composition. 115 In individuals assigned to a VLCKD, the body weight (weighted mean difference [WMD]−0.91 kg, 1,415 patients), TG (WMD 0.18 mmol/l, 1,258 patients), and diastolic blood pressure (WMD-1.43 mmHg, 1,298 patients) decreased, whereas HDL-C (WMD 0.09 mmol/l, 1,257 patients) and LDL-C (WMD 0.12 mmol/l, 1,255 patients) increased, and resulted in a greater weight loss than in those assigned to a low-fat diet in the long term; thus, a VLCKD may be an alternative option in obesity. 116 Similarly, a meta-analysis of randomized controlled trials (RCTs) demonstrated that, compared to low-fat diets, KD more effectively improved the metabolic parameters associated with glycemic, weight, and lipid control in obese participants, especially those with preexisting diabetes. 117
Plasma lipids constitute the main exigent concern with the KD in the treatment of obesity. The general opinion is that a low-carbohydrate, high-protein, and high-fat diet is potentially unhealthy because it may increase LDL-C and TGs, which is an especially important issue in obese individuals. However, several lines of evidence support the positive effects of the KD on these cardiovascular risk factors. The majority of studies amply demonstrate that reduced carbohydrate uptake can decrease total cholesterol and TG levels and increase the HDL-C level. 116 , 118 Furthermore, KD increase the size and volume of LDL-C particles 59 which could alleviate the risk of cardiovascular disease that is attributable to the higher atherogenicity of smaller LDL particles. In addition, KD influence endogenous cholesterol synthesis, whereby an increase in blood glucose and insulin levels increases endogenous cholesterol synthesis. In turn, reduced carbohydrate uptake accompanied by proper cholesterol intake inhibits cholesterol biosynthesis.
The KD is obviously effective in the weight control of obese individuals; however, the underlying mechanism is incompletely understood. Researchers have proposed several mechanisms for the KD effect on weight loss including: (1) reduced appetite due to the higher satiety effect of proteins (by increasing the concentrations of “satiety” hormones, such as glucagon-like peptide-1, cholecystokinin, and ghrelin), 119 , 120 effect on appetite-control hormones, 121 and a possible direct suppression of appetite by KBs, such as β-HB, which act both in energy/satiety signaling and in mediating the central satiety signal; 122 , 123 (2) reduced lipogenesis due to improved insulin resistance 124 and increased lipolysis due to increased expression of lipolytic enzymes, such as adipose triglyceride lipase, hormone-sensitive lipase, and lipoprotein lipase; 125 (3) higher metabolic efficiency in consuming fats that is indicated by the reduction in the resting respiratory quotient; 126 , 127 and (4) increased energy consumption due to increased gluconeogenesis, which is an energy-intensive process that costs ~400–600 Kcal/day and the thermic effect of protein, which has the highest energy cost among all the three macronutrients (Fig. 3 ). 128 , 129
Besides fat and weight loss, the KD can exert a series of other beneficial effects on obesity. Insulin resistance is common in obese patients. Very-low-carbohydrate diets could improve glycemic control, HbA1c levels, and lipid markers in obese individuals before obvious weight loss occur, which indicates that the KD could improve metabolic markers independent of weight loss. Moreover, in isocaloric experiments, the KD showed a dramatic improvement of metabolic markers than a low-fat diet in obese participants with insulin resistance. 130 The KD may protect against obesity-induced cognitive damage 131 and confer positive effects on mood in obese participants. 132 , 133 Another postulated beneficial effect of KD is related to longevity. Although restricted to animal studies, the KD is related to several pathways in metabolic syndrome and cancer, including increased 5′ adenosine monophosphate-activated protein kinase (AMPK) activity, inhibition of the mTOR/AKT pathway, 134 lowering the serum ratio of insulin-like growth factor/IGF-binding protein 3 135 and increasing peroxisome proliferator-activated receptor-γ coactivator-1α expression (a master mitochondrial metabolic regulator that can increase mitochondrial biogenesis). 136
Nonalcoholic fatty liver disease
Nonalcoholic fatty liver disease (NAFLD) is a highly prevalent disease that is characterized by hepatic adiposity, which comprises fatty liver, fibrosis, and inflammation. The earliest stage of NAFLD is hepatic steatosis, wherein TG accumulate in >5% of hepatocytes or the intrahepatic TG concentrations exceed 55 mg/g liver (5.5%). 137 , 138 Weight loss is recommended for the general clinical management of NAFLD.
The LCDs, especially those with high-fat content, are reported to have a worsening effect on hepatic steatosis due to the influence on cholesterol levels and hepatic function. However, the beneficial effects of KD on NAFLD have been widely reported. A meta-analysis of ten studies examining the effects of LCDs on NAFLD revealed that participants with NAFLD who followed LCDs exhibited a significant reduction in intrahepatic lipid content; however, there was no significant alteration in the concentration of liver enzymes. 139 Moreover, the KD exerts a more beneficial effect on NAFLD parameters than interventions such as calorie-restricted and low-fat diets. A small clinical trial revealed a greater reduction in intrahepatic TGs in patients with NAFLD after a 2-week carbohydrate-restricted diet without calorie restriction than in patients who received a calorie-restricted diet, without any significant weight-loss differences in the two treatment groups. 140 Consistently, a 2-year multicenter trial that included more than 300 patients who enrolled in a comprehensive lifestyle modification regimen demonstrated similar weight-loss patterns with low-fat diets and LCDs, although the LCD group displayed superior HDL-C profiles. 141
The KD might protect against NAFLD through several mechanisms 142 (Fig. 3 ). On one hand, due to its low-carbohydrate content, the KD could decrease insulin levels, with a consequent increase in fat oxidation and reduced lipogenesis, 143 and induce a microbiome shift, with increased folate production and limited inflammatory and oxidative stress. 54 On the other hand, the KD-induced KBs may result in (1) satiety, which limits food intake and facilitates weight loss; 144 and (2) epigenetic modifications, which play an important role in NAFLD pathogenesis. For example, β-HB increases the histone acetylation of genes that encode resistance factors against oxidative stress; 145 (3) activates GPR109A, which is widely expressed in various types of immune cells and exerts anti-inflammatory effects in many diseases, including obesity, inflammatory bowel disease, and cancer; 109 , 146 (4) inhibits NLRP3, 147 a key inflammasome that activates pro-inflammatory cytokines, such as IL-1β and IL-18, which closely correlate with obesity and T2DM pathogenesis. 148
Polycystic ovarian syndrome
The polycystic ovarian syndrome (PCOS) is closely related to other metabolic and endocrinological abnormalities, including insulin resistance, hyperinsulinemia, T2DM, dyslipidemia, and hyperandrogenism, and is characterized by insulin resistance, androgen excess, and abnormal gonadotropin dynamics. Therefore, the treatment goal is to improve insulin resistance and weight loss and decrease luteinizing hormone (LH)/follicle-stimulating hormone (FSH) ratios and excess androgen levels (Fig. 3 ).
The KD has been postulated to have a positive impact on women with PCOS. A study implemented a 6-month period of the KD for women diagnosed with PCOS, with BMI greater than 27 kg/m 2 , and no other serious medical conditions. After 24 weeks, women with PCOS showed a significant decrease in fasting serum insulin (pre, post-design: 23.5 to post-design: 8.2), in the LH/FSH ratio (2.23 to 1.21), and the free testosterone level (2.19 to 1.70). Furthermore, participants had an overall mean weight loss of 12.1% and a mean 4.0 kg/m 2 decrease in BMI. 149 A crossover study compared the effects of a standard diet and an LCD on PCOS and showed that the LCD decreased glycemia, fasting serum insulin, and testosterone, and increased insulin sensitivity. 150 Paoli et al. reported similar results, with significant reductions in BMI, glycemia, insulin, LDL-C, HDL-C, TGs, LH, testosterone, and dehydroepiandrosterone sulfate. The initial reversal of the LH/FSH ratio did not persist after 12 weeks. 151 Although these studies demonstrated a beneficial effect of the KD on PCOS, they have limitations such as small sample size, broad age range, single-arm design, and a short intervention time interval.
The exact mechanism by which the KD achieves therapeutic benefits in PCOS remains unclear. The exact mechanism by which KD achieves therapeutic benefit on PCOS remains unclear. A variety of studies identify a central role of insulin resistance in the pathogenesis of PCOS. Fasting insulin shows a positive correlation with androgen levels in women with PCOS. 152 Insulin stimulates increased production of androgens in theca cells isolated from women with PCOS, which effect is mediated by the insulin receptor. 153 Furthermore, excess insulin inhibits liver sex hormone-binding globulin synthesis, which increased the delivery of free androgens to target tissue. It has also been reported that AMPK, a regulator of cellular metabolism and energy balance, plays an essential role in the improvements of KD toward PCOS. 154
Function in neurodegenerative diseases
Alzheimer’s disease.
Alzheimer’s disease (AD) affects ~50 million people worldwide and is characterized by cognitive impairment that is associated with a progressive decline in memory, impaired self-care, disorientation, and personality changes. 14 AD induces changes in amyloid precursor protein cleavage and production of the amyloid precursor protein fragment beta-amyloid (Aβ) along with hyperphosphorylated tau protein aggregation. 155 Patients with AD have mitochondrial dysfunction and metabolic changes, such as impaired glucose utilization in the brain. 156 During the past decades, changes in dietary patterns and lifestyle modifications have had potential application in the treatment of AD and have received extensive attention in AD research, including calorie restriction, dietary approaches to stop hypertension, Mediterranean diet, and the KD. 157
The KD is a biochemical model of fasting or starvation, which promotes the utilization of KBs as the dominant fuel source to replace glucose in the central nervous system. 158 This may modulate the neuropathological and biochemical changes observed in AD, and the KD can directly reduce the accumulation of amyloid plaques while reversing Aβ toxicity; furthermore, KBs may protect against Aβ neurotoxicity. 159 Kashiwaya et al. treated cultured rat hippocampal cells with Aβ, β-HB, or both Aβ plus β-HB. Treatment with Aβ alone resulted in reduced neurite numbers and length compared to controls, and the additional treatment with β-HB reversed Aβ toxicity, suggesting that β-HB could potentially play a neuroprotective role against Aβ toxicity. 160
In addition, the development of AD is associated with hypometabolism, mitochondrial dysfunction, oxidative stress, and inflammation (Fig. 4 ). 161 , 162 , 163 , 164 Impaired glucose uptake and utilization in regional brain energy-substrate hypometabolism may be one of the earliest hallmarks of AD and suggests a potential avenue for compensating the brain energy deficit in AD dementia with ketones. 165 Both β-HB and acetoacetate bypass glycolysis to reduce acetyl-CoA, which can then be channeled into the Krebs cycle, and can thus increase energy availability in the brain. 166 In AD, brain ketone uptake is unimpaired, which makes KBs a viable alternative energy source.
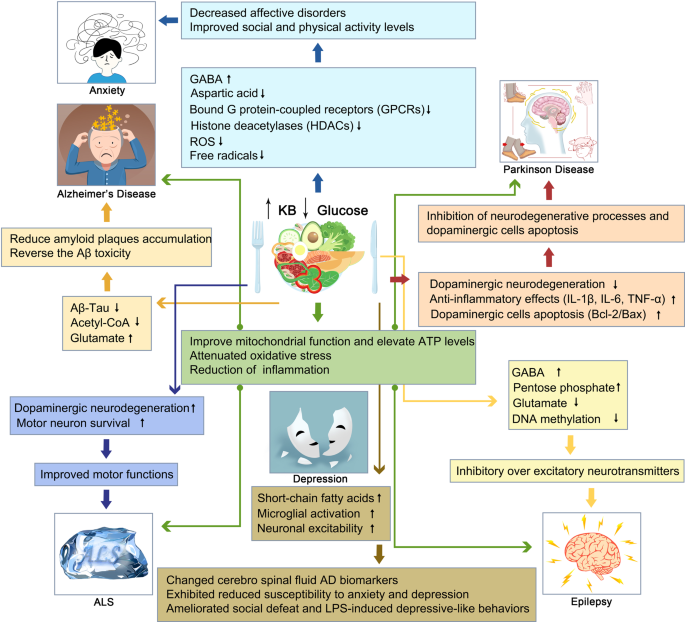
Mechanisms of the KD on neuromuscular and neurodegenerative diseases, including AD, PD, ALS, and epilepsy. Ketogenic diets altered the neuropathological and biochemical behavior through a variety of mechanisms including increasing mitochondrial function and ATP producing, decreasing oxidation stress and inflammation in the brain, and improving motor function and motor neuron survival
Mitochondrial dysfunction and oxidative stress play significant roles in neurodegenerative diseases, and both generate high levels of reactive oxygen species (ROS), which are harmful to all cellular macromolecules. 167 Importantly, the KD could improve mitochondrial numbers and function by inhibiting glycolysis and increasing KB formation to provide neuroprotective benefits in the neuronal cell line (SH-SY5Y). 168 In addition, KBs may regulate the homeostatic status of mitochondria by modulating calcium-induced membrane permeability transition. 169 Moreover, the KD elevates brain ATP and induces a higher phosphocreatine/creatine ratio and glutamate levels, but decreases glycogen levels. 170 Lu et al. reported that the KD increased superoxide dismutase activity and attenuated oxidative stress by activating Nrf2. 171
Furthermore, the KD reduces inflammation through established effects. The β-HB receptor HCA2 could activate a neuroprotective phenotype of macrophages depending on PGD2 production by COX1 and hematopoietic PGD2 synthase. 172 Pretreatment with the KD is associated with a reduction of pro-inflammatory cytokines, such as IL-1β and tumor necrosis factor alpha (TNF-α), which are induced by lipopolysaccharide injection, thereby suggesting that KD has anti-inflammatory properties. 173 Another mechanism of KD is the inhibition of histone deacetylases (HDACs) by the β-HB. 163 HDACs are a kind of protease that alters chromatin structure, and accessibility. HDACs are inhibited by β-HB, which improves memory function and synaptic plasticity. 170 , 174 Moreover, the KD inhibits the activation of NF-κB in activated B cells and downregulates COX2. 77 Thus, the KD could regulate dysregulated brain metabolism, mitochondrial homeostasis, and inflammation in AD.
In a transgenic mouse model of AD, the KD reduced the level of soluble Aβ deposits in the brain by 25% after only 40 days. 175 In addition, treatment with the KD, exogenous β-HB, and MCT reduced brain Aβ levels and improved cognitive ability. 176 When a mouse model of AD was fed a KD for 43 days, serum levels of the KB β-HB increased, total Aβ levels decreased, mitochondrial function improved, and oxidative stress decreased compared to controls. 177 Aged dogs receiving MCT showed dramatically improved mitochondrial function and decreased oxidative damage compared to age-matched controls. 178 In aged rats, the KD improved cognitive performance due to increased angiogenesis and capillary density, supporting the theory that diet-induced ketosis is beneficial in the treatment of neurodegenerative conditions. 179
Clinical data show that significant increases in β-HB levels and improved memory were observed in 20 participants with AD or mild cognitive impairment when they were administered MCT. 180 A randomized, double-blind, placebo-controlled, multicenter trial compared the effects of MCT on memory and cognition and demonstrated that elevated serum β-HB levels improved cognitive function and memory. Phillips et al. conducted a randomized crossover trial of a modified ketogenic diet in the treatment of Alzheimer’s disease. The results showed that patients achieved sustained physiological ketosis (12-week mean beta-hydroxybutyrate level: 0.95 ± 0.34 mmol/L). Compared with the usual diet, patients on the ketogenic diet increased their mean within-individual ADCS-ADL ( P = 0.0067) and QOL-AD ( P = 0.023) scores. 181 Another study compared an LCD with a high-carbohydrate diet in 23 adult patients treated with MCTs for 6 weeks. The LCD showed better memory performance and was positively correlated with levels of KBs. 182 Krikorian et al. reported maximum cognitive benefit of KD treatment in ApoE4(−) patients after 24-week treatment with MCT or a ketogenic product compared to placebo. 183 This observational study was limited to ApoE4(−) patients with mild AD. In a recent study, MCT was administered to 20 Japanese patients with mild to moderate AD over 12 weeks, and 120 min after MCT intake, the level of KBs increased; after 8 weeks, the patients demonstrated significant improvement in their immediate and delayed memory tests compared to their baseline scores. Additional research is warranted to determine the therapeutic benefits of MCT in patients with AD and to ascertain how APOE-4 status may mediate β-HB efficacy.
Parkinson’s disease
Parkinson’s disease (PD) is the commonest serious movement disorder worldwide and affects ~1% of adults who are older than 60 years. 184 PD is characterized by the loss of nigrostriatal dopaminergic neurons and a deficit in mitochondrial respiration. α-Synuclein is an overexpression protein in PD while its relationship with PD is unknown. The knockout of α-Synuclein only increased the number of dopamine neurons which suggested that the loss of synucleins does not produce parkinsonism. 185 In recent years, animal and in vitro studies have demonstrated the beneficial effect of KBs on the course of PD due to their function in mitochondrial homeostasis.
Joniec-Maciejak et al. observed that the administration of octanoic acid induced the inhibition of the neurodegenerative processes seen after 1-methyl-4-phenyl-1,2,3,6-tetrahydropyridine (MPTP) administration and was related to increased metabolic activity in striatal mitochondria. 186 One of the important factors that cause PD is DNA oxidative damage mediated by reactive oxygen species. It has been discussed that the injury of the respiratory chain and the mutations of mitochondrial DNA found in patients with PD suggest the importance of oxidative stress in PD. 187 The accumulation of hydroxyl radicals in the brain may lead to increased dopamine metabolism and, at the same time, to the accumulation of iron in the redox form of neurons. 188 KBs produced by liver metabolism not only promote mitochondrial respiration by increasing ATP production, but also reduce free radical production by increasing the efficiency of the mitochondrial respiratory chain complex (increasing NADH oxidation and inhibiting mitochondrial permeability conversion). An increase in anti-peroxidase in the hippocampus protects the central nervous system from degenerative changes. 13 , 189 Other studies showed that the KD exerted anti-inflammatory effects by decreasing the levels of pro-inflammatory cytokines, including IL-1β, IL-6, and TNF-α, in the substantial nigra. 190 Cheng et al. found that β-HB could inhibit the apoptosis of dopaminergic cells that were exposed to MPTP in relation to the upregulation of Bcl-2/Bax mRNA. 191 A summary of the KD-induced changes in PD is shown in Fig. 4 .
In a rat model of PD, the KD, via glutathione activity, protected dopaminergic neurons of the substantia nigra against 6-hydroxydopamine neurotoxicity. 192 In mice, β-HB infusion confers partial protection against MPTP-induced dopaminergic neurodegeneration and motor deficits. 193 These effects appear to be mediated by a Complex II-dependent mechanism that improves mitochondrial respiration and ATP production. Shaafi et al. observed a beneficial influence of the KD on motor function in a rat model of PD and confirmed that, when coadministered, the KD potentiated the efficacy of pramipexole. 194
In a clinical study, five patients with PD who consented to adhere to KD rules in their home environments were observed for 28 days and exhibited some improvements in the Unified Parkinson’s Disease Rating Scale. 195 In another pilot RCT, the effect of a low-fat diet versus the KD in 47 PD patients was assessed; 44 participants commenced the diets, and 38 completed the study (86% completion rate). The KD group maintained physiological ketosis. Both groups showed significantly decreased MDS-Unified Parkinson’s Disease Rating Scale scores, but the KD group showed a greater decrease in Part 1 (−4.58 ± 2.17 points; 41% improvement in baseline Part 1 scores) compared to the low-fat group (−0.99 ± 3.63 points; 11% improvement) ( P < 0.001); the largest intergroup differences in decreases were observed for urinary problems, pain and other sensations, fatigue, daytime sleepiness, and cognitive impairment. The results of the study indicated that it is plausible and safe for PD patients to maintain a low-fat diet or KD for 8 weeks. Both diet groups showed significant improvements in motor and nonmotor symptoms; however, the ketogenic group showed greater improvements in nonmotor symptoms. 196
Amyotrophic lateral sclerosis
Amyotrophic lateral sclerosis (ALS) is a neurodegenerative disease caused by the death of motor neurons in the spinal cord and brainstem, resulting in death within 2–5 years of symptom onset and respiratory paralysis. The accepted hypothesis of ALS pathogenesis includes loss of oxidative control with an excessive generation of oxidative free radicals, accumulation of neurofilaments, and excitotoxicity linked to an increase in the neurotransmitter glutamate, with resultant mitochondrial membrane dysfunction. 197 Furthermore, at least 20 genes are associated with ALS. Most familial and sporadic cases of ALS are caused by variants of the superoxide dismutase 1 (SOD1), C9orf72, FUS, and TARDBP genes. 198 To date, there are no effective treatments for ALS. The only US FDA-approved pharmacological therapy is riluzole, which could prolong survival by ~2 months in ALS patients. 199
Mitochondrial dysfunction may play an important role in the pathogenesis of ALS, which makes it a potential novel therapeutic target of intervention for ALS. Kong showed that KD can increase antioxidant power and attenuate oxidative stress in spinal cord injury by suppressing class I histone deacetylases. 200 Mutations in the gene encoding Cu/Zn SOD1 lead to abnormal mitochondrial activity, which is associated with a portion of ALS. 201 In energy alterations secondary to the mitochondrial dysfunction in ALS, the KD increased energy production and regulated mitochondrial activity by restoring the activity of complex I of the electron chain whose function is reduced in ALS (Fig. 4 ). 202
Moreover, the KD improved motor functions, which were associated with increased motor neurons in a mouse model of ALS. In the SOD1-G93A transgenic ALS mouse model, the KD led to higher motor neuron survival and an improvement in motor function compared to KO mice without the KD; furthermore, weight and synthesis of ATP at the mitochondrial level increased. 202 Mice that were fed the KD exhibited better motor performance on all motor function tests at 15 and 16 weeks of age compared to controls and, after the implementation of the Deanna protocol based on the KD, significantly extended the survival time of SOD1-G93A mice; 63% of mice in this group lived beyond 125 days, whereas only 9% of the control animals survived beyond this timepoint. 203 In another study, SOD1-G93A mice were treated with caprylic triglyceride, a medium-chain TG that is metabolized into KBs and can serve as an alternative energy substrate for neuronal metabolism. Treatment with caprylic triglyceride attenuated the progression of weakness, protected spinal cord motor neuron loss, and promoted the mitochondrial oxygen consumption rate in vivo. 204 These studies indicate that KD treatment may have a high impact on the quality of life of ALS patients.
Epilepsy is a chronic brain disorder characterized by recurrent seizures, which are short episodes of involuntary movement that can affect a part or all of the body, and are sometimes accompanied by loss of consciousness or loss of control of bladder or bowel function. The causes of epilepsy include brain tumors, stroke, brain infection, severe head injury, congenital abnormalities associated with brain defects, brain damage due to prenatal or perinatal injuries, and certain genetic syndromes. 205 Although 50–70 million people worldwide suffer from epilepsy, the available pharmacological treatment for epilepsy has limited effectiveness. 206
The KD was first recognized as an effective treatment for epilepsy. Studies have re-emerged that demonstrating the efficacy of KD in patients with drug-resistant epilepsy and some pediatric epilepsy syndromes. 207 The anticonvulsant mechanisms of the KD are incompletely understood, and the potential mechanisms of the KD are based essentially on the role of neurotransmitters, brain energy metabolism, ion channels, and oxidative stress (Fig. 4 ). 7 One study confirmed that norepinephrine and the orexigenic neuropeptide galanin are the two classes of molecules that contribute to the anticonvulsant effect of the KD. 208 However, in children with refractory epilepsy, the KD does not alter the level of norepinephrine but significantly alters the levels of dopamine and serotonin metabolites in the cerebrospinal fluid. 209 And a significant increase in γ-aminobutyric acid (GABA) and agmatine levels, without changes in glutamate levels, was observed in the hippocampus of rats subjected to a KD for 15 days compared to rats on a normal rat chow diet; this supports the notion that the KD modifies different transmitters while favoring inhibitory over excitatory neurotransmitters. 210 Recently, Zarnowski et al. find that the KD plays an important role in the neuroprotection and anti-convulsion via the kynurenine pathway. Kynurenic acid, the metabolite from the kynurenine pathway, participates in epilepsy. KBs, rich in KD, can inhibit glutamate which reduces the production of kynurenic acid. 211 While ATP is produced by mitochondrial respiration, the damaged substance ROS is produced at the same time. The uncoupling proteins, upregulated by mitochondrial respiration, can reduce the production of ROS and increase the resistance towards seizure. Moreover, the KD can also protect the mitochondrial DNA from ROS by improving the glutathione levels. In final, the KD can generate beneficial substances to reduce the happening of epilepsy. 212 , 213 Under KD conditions, the reduction of brain glucose utilization and glycolytic ATP production may induce potassium channels that are sensitive to ATP opening, which leads to the hyperpolarization of the neuronal membrane, 214 consequently reducing the electrical excitability of the brain and increasing the seizure threshold. Moreover, KD treatment increases polyunsaturated fatty acids and induces the expression of neuronal uncoupling proteins, regulates numerous energy metabolism genes, and induces mitochondrial biogenesis, which further limits ROS generation and increases energy production. 215
A meta-analysis reviewed 12 studies using classic KD, MAD, and classic KD in combination with MCT in adults with antiepileptic drug-resistant epilepsy. They found a combined efficacy rate of 52% for classic KD and 34% for MAD. The results indicate that a classical ketogenic diet may be more effective, and adult patients are likely to be less compliant with the KD than with an MAD. 216 In an observational study, at 3 months, 36% of 101 participants responded (≥50% seizure reduction) to diet therapy, and 16% were seizure-free. At 1 year, 30% responded, and 13% were seizure-free. At 4 years, 21% responded, and 7% were seizure-free. This study provided evidence that ketogenic diets may be feasible, effective, and safe in the long-term in adults, although long-term adherence was limited. Thus, adequately controlled studies are necessary to determine the efficacy of ketogenic diets in the treatment of adults with epilepsy. 217 An RCT in Iran compared the proportion of patients with focal or generalized epilepsy achieving ≥50% seizure reduction between 34 patients randomized to 2 months of MAD (22 completed the study), compared to 32 patients randomized to standard medical management, and found 35.5% (12/34) efficacy in the MAD group (intention-to-treat (ITT) analysis) at 2 months compared to 0% in the control group, a difference that was statistically significant. 218 In contrast, an RCT in Norway compared the change in seizure frequency in patients with drug-resistant focal or multifocal epilepsy between 37 patients randomized to 12 weeks of MAD (of whom 28 received the intervention and 24 completed the study) and 38 adults randomized to their habitual diet (of whom 34 received the intervention and 32 completed the study). 219 Overall, ITT data from adult observational studies demonstrate responder rates of 22–70% for classic KD and 12–67% for MAD, with some suggestion of increased efficacy in adults with generalized rather than focal epilepsy. 220 , 221 Additional RCTs with larger sample sizes are warranted to investigate the efficacy of the MAD in different subpopulations of adult patients with epilepsy. Thus, the KD offers a necessary adjunctive strategy for management with the advantages of potentially synergizing concurrent treatments and reducing the need for prolonged use of anesthetic drugs.
Depression is a common kind of mood disorder that involves reduced inhibitory GABA neurotransmission. KD may do a favor to depression via its nutrition and microbiome that attracts more attention from doctors and researchers. The main source of GABA is the glutamate–glutamine cycle which happens to be adequate in KD. Moreover, KD is full of other nutrition such as ω-3s, tryptophan, vitamin B, which may regulate the disorders in both physiology and psychology. 222 The gut–brain axis suggests that the microbiome plays an important role in mental health and an existing study proves that the KD may affect mental health through the gut–brain axis by the gut microbiome. Nagpal et al. find that the KD alters the gut microbiome signature and short-chain fatty acid in association with cerebrospinal fluid AD biomarkers. 223
KD has been reported to exhibit preventative effects on depressive-like behaviors in rodents while its exact mechanism is still unknown. As we noticed before, the adult offspring of pregnant mice fed by KD exhibited less prone to depression behaviors. 224 Recently, Sun et al. find that a ketogenic diet can ameliorate social defeat and lipopolysaccharide-induced depressive-like behaviors by the restoration of microglial inflammatory activation and neuronal excitability. 225 Also, Campbell’s report revealed that a ketogenic diet may lead to mood stabilization. 226 According to the randomized controlled trial, DM. et al. find that the KD group showed lower levels of anxious and mood-disturbed behaviors. 227
Anxiety disorders
Anxiety disorder is an increasingly common mental disorder worldwide, resulting in considerable mental stress for patients, and social medicine and economic burden. According to epidemiological statistical analysis, about one-third of the global population is affected by anxiety disorders during their lifetime. 228 Current treatments including drugs, psychological interventions, and so on still fail to meet the need for a cure. Recently, nutritional therapies such as ketogenic diets are considered promising due to their effectiveness in preventing relapse.
Disorders in the metabolism of neurotransmitters are considered to be a major factor in anxiety disorders. A deficiency of the central inhibitory neurotransmitter GABA has been linked to anxiety, depression and other affective disorders. Due to the decrease of blood glucose level during the implementation of the ketogenic diet, the glycolysis pathway in the brain is significantly inhibited, and the energy supplier of the central nervous system changes from glucose to ketone bodies. Excitation of the steroid pathway enhances the synthesis and transmission of GABA at the synapse, while decreasing the content of aspartic acid and the excitability of neurons. 229 , 230 It is suggested in several studies that the progeny of mice exposed to KD during pregnancy show lower susceptibility to anxiety and depression and significantly improve social and physical activity levels compared to the standard diet group.
On the other hand, bi-directional interactions between the central nervous system and the intestinal microbiome are associated with the development of psychiatric disorders. With the disorder of intestinal microflora, the barrier function of the intestinal epithelium is gradually reduced or even lost with the increased permeability, which then mediates the enhancement of immune response and causes chronic neuritis. Neurotransmitter metabolism, particularly serotonin metabolism, which is closely associated with the onset of anxiety and depression, would be disrupted. 231 Mechanistically, ketones produced by liver metabolism act as both energy supply molecules and signal molecules involved in binding G protein-coupled receptors, inhibiting HDACs, regulating the abundance of intestinal microbiota, improving intestinal barrier function, and reducing the production of ROS and free radicals. 232 , 233 Experiments on mice have demonstrated the positive effects of KD on the intestinal microbiome. 234 Overall, KD has an ideal potential to be further used to the mood disorder and the mechanism behind remains to be explored.
Function in cancer
Cancer is one of the greatest global public health challenges and is a leading cause of global mortality. Complementary approaches to significantly enhance the efficacy of standard anticancer therapies are scarce. The KD appears to sensitize most cancers to standard treatment by exploiting the reprogramming metabolism of cancer cells, making it a promising candidate in adjuvant cancer therapy. 17
Tumor cells use glucose as the primary energy source. To meet the requirements of rapid proliferation, tumor cells utilize glycolysis, even in the presence of oxygen: a phenomenon known as the “Warburg effect”. 235 Thus, any pharmacologic intervention that reduces intratumoral glucose levels may be effective for slowing tumor growth. During KD implementation, tumor cells have limited access to glucose and cannot use KBs as an energy source owing to aberrant mitochondrial function and reduced enzymatic activity for ketone consumption, which makes the KD a promising approach for cancer prevention. Due to a reduction in blood glucose levels, the KD could concurrently affect glucose metabolism and glucose-dependent signaling in tumor cells. 236 Furthermore, glucose starvation leads to a suppressed lactate/pyruvate cycle, which inhibits neovascularization, hypoxia-induced vascular epidermal growth factor activation, and angiogenesis, and causes ultimate necrosis in tumor cells, especially for colon adenocarcinoma xenografts. 237 KBs can inhibit inflammation, which is closely correlated with tumor pathogenesis. By inhibiting the NLRP3 inflammasome, β-HB diminishes the inflammatory microenvironment, which provides ancillary therapeutic benefits for therapeutic interventions in glioblastoma. 238 Interestingly, GPR109A, a receptor for β-HB, is downregulated in cancer. GPR109A, a tumor suppressor, was downregulated when β-HB synthesis was suppressed. Therefore, low levels of β-HB attenuate the tumor-suppressive function of GPR109A in colon cancer cells. 239 Recent studies have extended the tumor-suppressive function of the receptor beyond the colon, as GPR109A suppressed mammary tumorigenesis in a mouse model of breast cancer. 240
Furthermore, the KD could enhance the efficacy of phosphatidylinositol 3 kinase (PI3K) inhibitors and overcome drug resistance, which was confirmed in different mouse cancer models, including pancreatic, bladder, endometrial, and breast cancer, as well as in acute myeloid leukemia. The KD improves the efficacy of anti-PI3K treatment and drug resistance by decreasing hyperglycemia and reducing the insulin-secretory response, and these effects correlated with reduced intratumoral mTORC1 signaling. 241 In a mouse model of melanoma, acceleration of proliferation in BRAF V600E-mutated melanoma cells after the KD treatment was observed because of the selectively increased activation of BRAF V600E-mutant-dependent MEK1 signaling by the KB acetoacetate. In contrast, NRAS Q61K- and Q61R-mutated and BRAF wild-type melanoma cells were unaffected by the KD. 242
Besides slowing tumor growth, KD sensitizes tumor cells to classic chemotherapy or radiotherapy in neuroblastoma, glioma, and lung cancer. 243 , 244 , 245 , 246 For example, the KD supplemented with medium-chain TG enhanced the antitumor and anti-angiogenic efficacy of chemotherapy on neuroblastoma xenografts in a CD1-nu mouse model. 247 In addition, KD showed promising benefits in boosting the effect of anti-PD-1/PD-L1 immunotherapy. Ferrere et al. found that anti-PD-1, alone or in combination with anti-CTLA-4, failed to inhibit tumor growth in mice receiving a standard diet, whereas KD implementation, or oral administration of 3-hydroxybutyrate (3-HB), a principal KB generated in the KD, reestablished therapeutic responses. 248 3-HB prevented the upregulation of PD-L1 on myeloid cells whereas promoting the expansion of CXCR3 + T cells and instituting consequent T cell-mediated tumor immunosurveillance. Similarly, Dai et al. reported that KD treatment decreased PD-L1 protein levels and increased the expression of type-I interferon (IFN) and antigen-presentation genes, leading to the enhanced efficacy of anti-CTLA-4 immunotherapy. 249 The key molecular event of KD treatment in promoting immunotherapy is the activation of AMPK, which phosphorylates PD-L1, resulting in its disrupted interaction with CMTM4 and subsequent PD-L1 degradation, which phosphorylates EZH2, resulting in improper PRC2 function and subsequently enhanced expression of IFN and antigen-presentation gene. 249
Many studies investigating the effect of the KD on tumor metastasis indicate a metastasis-inhibiting effect of the KD. 250 , 251 , 252 An early study reported that KBs could inhibit the growth rate of malignant lymphoblasts (Raji cells) and diet-induced ketosis could reduce the number of B16 melanoma deposits in mouse lung. 251 Combined treatment with the KD and hyperbaric oxygen significantly reduced the tumor-growth rate and diminished metastatic spread, while increasing survival, in the VM-M3 mouse model of metastatic cancer, possibly through induction of ROS production in tumor cells. 250
Apart from preclinical studies, clinical trials have demonstrated the beneficial effects of the KD on antitumor therapy. A clinical trial showed that positron emission tomography revealed an average decrease of 21.8% in glucose uptake at the tumor site in children after 8 weeks of KD implementation. One child displayed significant mood improvement, promotion of skill-learning ability, and remained free of disease progression after continuing the KD for 12 months. All participants remained in remission for 4–5 years after diagnosis, with good quality of life. A prospective feasibility trial applying the MAD to patients with glioblastoma who were not receiving chemotherapy reported that four patients were stable or improved after 16 weeks of dietary intervention. 253 A randomized controlled clinical trial showed that TNF-α decreased significantly after 12 weeks of treatment ( P < 0.001), while IL-10 increased ( P < 0.001) in the intervention compared to the control group. Patients in the KD group had lower adjusted serum insulin compared to the control group ( P < 0.002). KD lead to a reduction in tumor size in the KD compared to the control (27 vs 6 mm, P = 0.01). Stage decreased significantly in patients with locally advanced disease in the KD group after 12 weeks ( P < 0.01). 254 A study that investigated the effect of KD in combination with intranasal perillyl alcohol in patients with recurrent glioblastoma found that after 3 months of combined therapy, the KD increased the partial response rate to 77.8%, compared with 25% in the control group. The percentage of tumor progression was 11.1% in the KD group, compared to 50% in the control group. 255 A study among ovarian and endometrial cancer patients reported that the KD improved overall physical health and increased energy in patients without chemotherapy. 256 Therefore, the administration of KD may be a potential approach to enhance the therapeutic efficacy of chemotherapy. In addition, the boundary between a high-fat diet and KD still needs to be further clarified. Recently, Jun Yu’s team found that high-fat diet can drive colorectal tumors by inducing gut microbiota dysbiosis, metabolic changes, and intestinal epithelial barrier dysfunction in mice, and revealed potentially key bacteria and metabolites. Moreover, compared with a high-oleic acid diet, a high-fat diet rich in linoleic acid can specifically promote breast tumor growth. 257
Taken together, the KD has shown benefits in tumor-growth inhibition and enhanced efficacy of multiple antitumor therapies in various types of cancer, including glioblastoma, prostate, colon, pancreatic, and lung cancer (Fig. 5 ). These mechanisms can be attributed to a limited glucose source and reduced inflammation. However, the efficacy of KD could be influenced by cancer type, subtype, genetic features, or tumor-associated syndrome. Future studies should focus on more molecular studies as well as RCTs with heterogeneous study designs and large samples in order to elucidate the mechanisms of the KD in tumor therapy and to evaluate the application of the KD in the clinical setting.
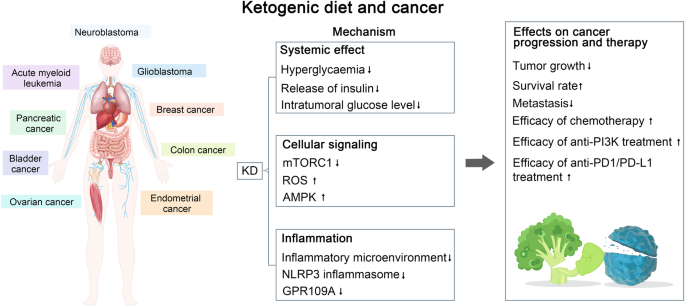
Summary of the potential interplay in the molecular mechanisms of the ketogenic diet (KD) and cancer. The KD exerts a therapeutic effect on tumors such as neuroblastoma, acute myeloid leukemia, glioblastoma, etc., through decreased GPR109A expression, mTORC1 activation, and glucose uptake at the tumor site, which leads to decreased tumor growth, increased survival, and increased chemotherapeutic efficacy
Function in cardiac diseases
Heart failure (HF) is characterized by metabolic abnormalities. Therefore, augmenting cardiac ATP production in a bioenergetically efficient manner is of significant interest to the HF field.
Growing evidence has demonstrated increased ketone body utilization in the failing heart. For example, expression of β-hydroxybutyrate dehydrogenase 1 (a key enzyme in the ketone oxidation pathway) and ketogenic β-hydroxybutyryl-CoA, in association with increased myocardial utilization of β-hydroxybutyrate, is increased in the heart failure samples supported the notion that the hypertrophied and failing heart shifts to ketone bodies as an alternate fuel and myocardial ketone oxidation as a key metabolic adaptation in the failing human heart. 258 , 259 In mice and canine pacing model of progressive heart failure, increased delivery of 3-HB ameliorates pathologic cardiac remodeling and dysfunction. Mice rendered incapable of oxidizing the ketone body 3-HB in the heart exhibits worsened heart failure compared with WT controls. These results indicate that the heart utilizes 3-HB as a metabolic stress defense and suggest that strategies aimed at increasing ketone delivery to the heart could prove useful in the treatment of heart failure. 260 Preclinical and clinical studies also suggest that exogenous delivery of ketones may improve cardiovascular function as well as prevent the development of pathological remodeling en-route to HF. 261 The potential mechanism of ketosis is associated with SGLT2 inhibitors and reductions in HF morbidity and cardiovascular mortality were observed in patients with HF (irrespective of diabetes status) further confirmed the potential benefits of ketones in patients with HF. 262
Zhang et al. have constructed mice with cardiomyocyte-restricted deletion of subunit 1 of MPC (cMPC1 −/− ). The mice develop age-dependent pathologic cardiac hypertrophy, transitioning to a dilated cardiomyopathy and premature death. The KD could increase the availability of non-glucose substrates in vivo and reverse the structural, metabolic, and functional remodeling of non-stressed cMPC1 −/− hearts. Although concurrent short-term KD did not rescue cMPC1 −/− hearts from rapid decompensation and early mortality after pressure overload, 3 weeks of the KD before transverse aortic constriction is sufficient to rescue this phenotype. 263
Although the beneficial effects of β-HB on HF have been acknowledged by numerous reports, its safety on other cardiovascular problems has been challenged by certain lines of evidence. In rat models, KD decreased mitochondrial biogenesis, reduced cell respiration, and increased cardiomyocyte apoptosis and cardiac fibrosis. Mechanistically, the KD increases levels of β-HB, promotes histone acetylation of the Sirt7 promoter, and activates Sirt7 transcription. This in turn inhibits the transcription of mitochondrial ribosome-encoding genes and mitochondrial biogenesis, leading to cardiomyocyte apoptosis and cardiac fibrosis. 264 This study highlighted the unknown detrimental effects of the KD.
In atrial fibrillation which is the most common arrhythmia encountered in clinical practice, the concentration of β-HB in heart tissues is significantly higher. 265 The potential detrimental effects of β-HB have also been confirmed in clinical studies. A retrospective study assessed the relationship between serum β-HB and prognosis in 405 stable hemodialysis patients. Increased serum β-HB levels are independently associated with cardiovascular events and all-cause death. In addition, increased circulating β-HB is independently associated with major adverse cardiovascular events. 266 Twenty patients on the ketogenic diet at one institution were investigated in another research. Prolonged QT interval was found in three patients (15%). There was a significant correlation between prolonged QT interval and both low serum bicarbonate and high beta-hydroxybutyrate. In addition, three patients had evidence of cardiac chamber enlargement. One patient with severe dilated cardiomyopathy and prolonged QT interval normalized when the diet was discontinued. 267 Taken together, these findings suggest that KD consumption or β-HB accumulation may increase the risks of cardiovascular disease, suggesting that long-term consumption of a KD should be carefully considered in cardiovascular disease.
By digesting animal protein and other components of animal products—red meat—symbiotic bacteria in the gut produce metabolites that have been linked to insulin resistance and cancer formation. One such molecule, trimethylamine N-oxide (TMAO), has recently gained a lot of attention as a possible and a closely linked risk factor for gut microbiota and cardiovascular and kidney disease. Trimethylamine is produced by gastrointestinal bacteria after they metabolize dietary choline and carnitine. Trimethylamine is then absorbed and oxidized to TMAO under the action of flavin-monooxygenases (FMOs), mainly FMO3. 268 KD changes the pattern of energy metabolism and makes more use of fat and ketone bodies through a very-low-carbohydrate and high-fat diet. The researchers compared the ketogenic diet in mice with the obesity-inducing high-fat diet and found that the ketogenic diet, while apparently healthier, significantly reduced glucose tolerance. The liver of the mice became less responsive to insulin and became insulin resistant. 269 In people with hepatic insulin resistance, low hepatic insulin activity increases FMO3 expression, further enhancing TMAO levels. Hepatic insulin resistance, often associated with hepatic steatosis, may lead to increased cardiovascular risk and an increased risk of type 2 diabete, and these increased risks are also associated with elevated TMAO (Fig. 6 ).
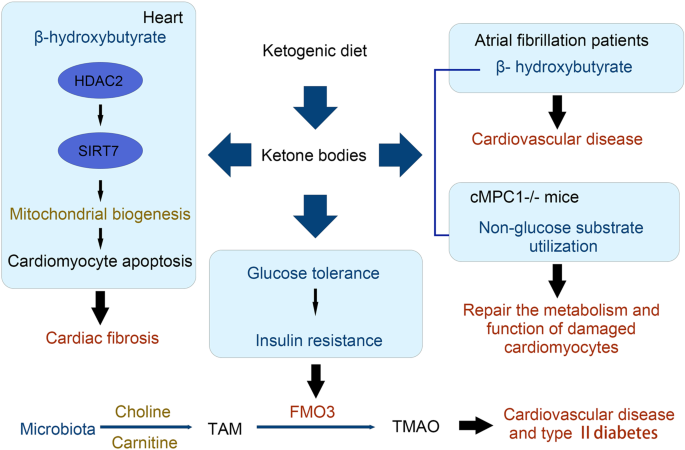
Improvements and mechanisms of the functions of the KD exert on cardiac diseases. The KD increases levels of β-HB, promotes histone acetylation of the Sirt7 promoter and activates Sirt7 transcription in cardiac fibrosis and increases the availability of non-glucose substrates in cMPC1 −/− hearts
Function in other inflammation diseases
Inflammatory bowel disease.
A recent study found that the KD can alleviate colitis in a dextran sulfate sodium mouse model compared with a low-carbohydrate diet and normal diet. 20 A 16-week KD intervention before colitis induction in mice was found to increase the abundance of Akkermansia and Roseburia and simultaneously alter the gut metabolites. Upon DSS treatment, the KD-fed mice had decreased weight loss and disease activity index scores with reduced inflammatory cell infiltration in the intestinal epithelium. The KD was found to exert an anti-inflammation effect via reducing the production of RORγt + CD3 − group 3 innate lymphoid cells as well as the related inflammatory cytokines including IL-17α, IL-18, IL-22, and CCL-4. The KD-fed mice remained to have overabundant Akkermansia in feces. Importantly, transplantation of the feces of KD-fed mice to germ-free mice retained the above effects, highlighting the KD-modulated gut microbiota have a profound role in alleviating colitis.
Irritable bowel syndrome
A study also showed that KD contributes to beneficial effects in a rat model of irritable bowel syndrome via reducing the stress on gut mitochondrial biogenesis. 270 Feeding with KD was found to be able to reduce intestinal inflammation, improve cellular redox status and restore mitochondrial function in the model. Such effects might be exerted due to the upregulation of the PPAR-γ/PGC-1α axis (Table 2 ).
The covid-19 pandemic is now a global threat. Especially, severe covid-19 with cytokine storm syndrome is desperately lethal and a main cause of mortality. The KD has been proposed as an adjunct therapy for covid-19 patients due to its contribution to the reduction of critical risk factors such as obesity, type 2 diabetes and hypertension, anti-inflammation, and metabolism modulation. 21 , 271 It is proposed that switching lipid metabolism, which can be achieved by a ketogenic diet rich in MCTs or by intermittent fasting, may disfavor viral replication and infection and inhibit cytokine storm. 272 Importantly, it is also believed that the induction of ketosis may help to prevent the cytokine storm. 273 , 274 A recent retrospective analysis of 34 covid-19 patients receiving an eucaloric KD in comparison to 68 who received a eucaloric standard diet showed that the former might have a lower risk in motility and ICU admission. 275 Thus, the KD could be a theoretical preventive and supportive care option for patients with covid-19. But clinical evidence are needed.
Adverse effects and challenges
Short-term adverse effects, such as fatigue, irritability, headache, nausea, dehydration, hypoglycemia, diarrhea, metabolic acidosis, and refusal to eat, are commonly seen during the first few weeks of the KD as responses to diet-induced metabolic shift and are usually predictable and preventable. 276 , 277 Long-term adverse effects of KD include elevated cardiovascular risks with poor cholesterol profiles and nephrolithiasis, likely due to the metabolic effects of the KD, such as uric acidemia, hypocitraturia, hypercalciuria, aciduria, growth retardation, decreased bone mineral density, anemia, and neuropathy, 276 , 277 and are usually monitored during follow-up. Furthermore, there are adverse effects that can span the duration of KD therapy, which frequently include gastrointestinal disturbances, including constipation, abdominal pain, emesis, and gastroesophageal reflux disease. 277 Due to the lack of sufficient clinical evidence of the long-term safety of the KD, the use of KD in chronic diseases remains debatable. 276
Moreover, due to the lack of sufficient clinical evidence demonstrating the efficacy and safety of KD, there still are debates on using KD in several diseases, 276 including diabetes, cardiovascular disease, and Alzheimer’s disease. As type 1 diabetes is often associated with metabolic irregularities, hyperketonemia and ketosis may increase the risk of complications. 278 Risks of KD in certain diseases are also demonstrated in animal models. In spontaneously hypertensive rats, the KD increases hypertension, 279 , 280 impairs endothelium-dependent relaxation in mesenteric arteries, 280 causes renal damage 279 and aggravates interstitial fibrosis and inflammatory responses in the heart. 281 In the rat, The KD exacerbated disorders of glucose and lipid metabolisms and activated the renin-angiotensin-aldosterone system, 279 and the ketone body might reduce eNOS expression via NF-κB singling pathway in vein endothelial cells, 280 which could collectively contribute to hypertension and endothelial dysfunction. Meanwhile, KD reduced renal autophagy to cause damage and the β-hydroxybutyrate was found to promote TGF-β-induced fibrosis in cardiac fibroblasts. 281 The KD can be detrimental to cognitive behavior. In an Alzheimer’s disease rat model, KD worsened cognitive performance likely because it exacerbated gut dysbiosis. 282 Consistently, a very recent study showed that the KD and hypoxia-altered gut microbiota increased intestinal IFN-γ-producing Th1 cells and consequently impaired cognitive behavior in mice. 86 Also, in an animal model of early Parkinson’s disease, long-term KD seemed to be insufficient for the neuroprotective effect. 283
Furthermore, the KD is a multidisciplinary therapy that requires the involvement of experienced caregivers, such as doctors, nurses, and dietitians, in addition to the patients. 284 Thus, successful implementation of the KD requires well-organized and consistently functioning cooperation between caregivers and patients, and clinical settings that support this cooperation. Moreover, as a patient-centered therapy, treatment adherence remains a major challenge of the KD. 277 , 284 Ineffectiveness, adverse effects, diet restrictiveness, and unpalatable taste may decrease the patient’s motivation and lead to the discontinuation and consequent failure of the treatment.
Future directions
Dietary interventions for the treatment and prevention of diseases are now widely acknowledged and have increasingly gained importance. Diet planning may not only serve as a medical approach for the treatment of diseases but can also provide a way to maintain health in the general population. The KD, as a potential therapeutic approach for various diseases, still faces challenges with regard to broad clinical application. Future studies are needed to provide high-quality clinical evidence on the efficacy and safety of the KD in diseases other than epilepsy; to further modify the diet to decrease the adverse effects and increase tolerability; and to comprehensively understand the mechanisms that may, in turn, guide disease- or patient-customized application and even a KD-derived drug design.
The KD exhibits broad therapeutic potential in many diseases other than epilepsy; however, the clinical implementation of the KD is not well established yet. High-quality RCTs must be conducted to confirm the safety and efficacy of the KD. In addition, based on clinical research, it is important to identify the syndromes and conditions that can benefit from the KD-based therapies, contraindications for use, KD variants with good effectiveness and tolerability, and factors and biomarkers that can predict KD-related outcomes, which may collectively guide the clinical utilization of the KD in different diseases.
Despite the adverse effects described, the KD usually does not have long-term tolerability, which not just introduces barriers to compliance by caregivers and patients but also compromises the clinical efficacy of the therapy. Although several KD variants are available to increase adherence, additional modifications are still needed and should be specifically designed for distinct diseases to increase both efficacy and tolerability, while facilitating dynamic adjustment to minimize the short- and long-term adverse effects.
The mechanisms of action of the KD, especially at the cellular and molecular levels, in different types of diseases, are poorly understood. As a metabolic therapy, the KD may function via a combination of multiple and complex mechanisms involving metabolic, cellular, and molecular responses. Recent studies have shown that the gut microbiota plays an important role in these processes. Given that the composition of the gut microbiota varies in specific diseases, the possibility of disease-specific mechanisms of KD that are mediated through a functional axis that originates with gut microbes is worth exploring regarding the beneficial effects and side effects of the KD. Therefore, further in-depth investigations into the intrinsic therapeutic mechanisms of the KD in different diseases are needed, as it will not only provide insights into disease pathogenesis from new perspectives, but also lead to the identification of key intermediate biochemical pathways, molecules, and/or other factors, such as gut microbes, that govern the KD treatment-related effects, and these can be utilized as promising targets for drug design or the development of novel customized interventional strategies to mirror the effects of KD.
Bowen, D. J. & Beresford, S. A. A. Dietary interventions to prevent disease. Annu. Rev. Public Health 23 , 255–286 (2002).
Article PubMed Google Scholar
Shreiner, A. B., Kao, J. Y. & Young, V. B. The gut microbiome in health and in disease. Curr. Opin. Gastroenterol. 31 , 69–75 (2015).
Article CAS PubMed PubMed Central Google Scholar
Chen, R. Y. et al. A microbiota-directed food intervention for undernourished children. N. Engl. J. Med. 384 , 1517–1528 (2021).
Article PubMed PubMed Central Google Scholar
Gehrig, J. L. et al. Effects of microbiota-directed foods in gnotobiotic animals and undernourished children. Science 365 , eaau4732 (2019).
Wilder, R. M. The effect on ketonemia on the course of epilepsy. Mayo Clin. Bull. 2 , 307–308 (1921).
Google Scholar
Peterman, M. G. The ketogenic diet. J. Am. Med. Assoc. 90 , 1427–1429 (1928).
Article CAS Google Scholar
Ulamek-Koziol, M., Czuczwar, S. J., Januszewski, S. & Pluta, R. Ketogenic diet and epilepsy. Nutrients 11 , 2510 (2019).
Article CAS PubMed Central Google Scholar
Wheless, J. W. History of the ketogenic diet. Epilepsia 49 (Suppl 8), 3–5 (2008).
Yuen, A. W. & Sander, J. W. Rationale for using intermittent calorie restriction as a dietary treatment for drug resistant epilepsy. Epilepsy Behav. 33 , 110–114 (2014).
Woodyatt, R. T. Objects and method of diet adjustment in diabetes. Arch. Intern. Med. 28 , 125–141 (1921).
Neal, E. G. et al. The ketogenic diet for the treatment of childhood epilepsy: a randomised controlled trial. Lancet Neurol. 7 , 500–506 (2008).
Neal, E. G. et al. A randomized trial of classical and medium-chain triglyceride ketogenic diets in the treatment of childhood epilepsy. Epilepsia 50 , 1109–1117 (2009).
Stafstrom, C. E. & Rho, J. M. The ketogenic diet as a treatment paradigm for diverse neurological disorders. Front. Pharmacol. 3 , 59 (2012).
Rusek, M., Pluta, R., Ulamek-Koziol, M. & Czuczwar, S. J. Ketogenic diet in Alzheimer’s disease. Int. J. Mol. Sci 20 , 3892 (2019).
Westman, E. C., Tondt, J., Maguire, E. & Yancy, W. S. Jr. Implementing a low-carbohydrate, ketogenic diet to manage type 2 diabetes mellitus. Expert Rev. Endocrinol. Metab. 13 , 263–272 (2018).
Article CAS PubMed Google Scholar
Muscogiuri, G. et al. The management of very low-calorie ketogenic diet in obesity outpatient clinic: a practical guide. J. Transl. Med. 17 , 356 (2019).
Weber, D. D. et al. Ketogenic diet in the treatment of cancer—where do we stand? Mol. Metab. 33 , 102–121 (2020).
Paoli, A. et al. Ketogenic diet and microbiota: friends or enemies? Genes 10 , 534 (2019).
Ang, Q. Y. et al. Ketogenic diets alter the gut microbiome resulting in decreased intestinal Th17 cells. Cell 181 , 1263–1275 e1216 (2020).
Kong, C. et al. Ketogenic diet alleviates colitis by reduction of colonic group 3 innate lymphoid cells through altering gut microbiome. Signal Transduct. Target. Ther. 6 , 154 (2021).
Gangitano, E. et al. Ketogenic diet as a preventive and supportive care for COVID-19 patients. Nutrients 13 , 1004 (2021).
Li, R. J., Liu, Y., Liu, H. Q. & Li, J. Ketogenic diets and protective mechanisms in epilepsy, metabolic disorders, cancer, neuronal loss, and muscle and nerve degeneration. J. Food Biochem. 44 , e13140 (2020).
PubMed Google Scholar
Abbasi, J. Interest in the ketogenic diet grows for weight loss and type 2 diabetes. J. Am. Med. Assoc. 319 , 215–217 (2018).
Article Google Scholar
Kossoff, E. H. et al. Optimal clinical management of children receiving dietary therapies for epilepsy: updated recommendations of the International Ketogenic Diet Study Group. Epilepsia Open. 3 , 175–192 (2018).
Blackford, R. Not your parents’ ketogenic diet—flexibility in 2020. Epilepsy Res. 162 , 106307 (2020).
Coppola, G. et al. The ketogenic diet in children, adolescents and young adults with refractory epilepsy: an Italian multicentric experience. Epilepsy Res. 48 , 221–227 (2002).
Hassan, A. M. et al. Ketogenic diet in the treatment of refractory epilepsy in childhood. Pediatr. Neurol. 21 , 548–552 (1999).
Seo, J. H. et al. Efficacy and tolerability of the ketogenic diet according to lipid:nonlipid ratios-comparison of 3:1 with 4:1 diet. Epilepsia 48 , 801–805 (2007).
Vaisleib, I. I., Buchhalter, J. R. & Zupanc, M. L. Ketogenic diet: outpatient initiation, without fluid, or caloric restrictions. Pediatr. Neurol. 31 , 198–202 (2004).
Huttenlocher, P. R., Wilbourn, A. J. & Signore, J. M. Medium-chain triglycerides as a therapy for intractable childhood epilepsy. Neurology 21 , 1097–1103 (1971).
Liu, Y. M. & Wang, H. S. Medium-chain triglyceride ketogenic diet, an effective treatment for drug-resistant epilepsy and a comparison with other ketogenic diets. Biomed. J. 36 , 9–15 (2013).
Schwartz, R. H., Eaton, J., Bower, B. D. & Aynsley-Green, A. Ketogenic diets in the treatment of epilepsy: short-term clinical effects. Dev. Med. Child Neurol. 31 , 145–151 (1989).
Vining, E. P. Clinical efficacy of the ketogenic diet. Epilepsy Res. 37 , 181–190 (1999).
Kossoff, E. H., Krauss, G. L., McGrogan, J. R. & Freeman, J. M. Efficacy of the Atkins diet as therapy for intractable epilepsy. Neurology 61 , 1789–1791 (2003).
Kossoff, E. H. et al. A modified Atkins diet is effective for the treatment of intractable pediatric epilepsy. Epilepsia 47 , 421–424 (2006).
Foster, G. D. et al. A randomized trial of a low-carbohydrate diet for obesity. N. Engl. J. Med. 348 , 2082–2090 (2003).
Kossoff, E. H. et al. A randomized, crossover comparison of daily carbohydrate limits using the modified Atkins diet. Epilepsy Behav. 10 , 432–436 (2007).
Kang, H. C. et al. Use of a modified Atkins diet in intractable childhood epilepsy. Epilepsia 48 , 182–186 (2007).
Sharma, S., Sankhyan, N., Gulati, S. & Agarwala, A. Use of the modified Atkins diet for treatment of refractory childhood epilepsy: a randomized controlled trial. Epilepsia 54 , 481–486 (2013).
Kim, J. A. et al. Efficacy of the classic ketogenic and the modified Atkins diets in refractory childhood epilepsy. Epilepsia 57 , 51–58 (2016).
Sharma, S. et al. Evaluation of a simplified modified Atkins diet for use by parents with low levels of literacy in children with refractory epilepsy: A randomized controlled trial. Epilepsy Res. 127 , 152–159 (2016).
Sharma, S., Sankhyan, N., Gulati, S. & Agarwala, A. Use of the modified Atkins diet in infantile spasms refractory to first-line treatment. Seizure 21 , 45–48 (2012).
Mehta, R. et al. Efficacy and tolerability of the modified Atkins diet in young children with refractory epilepsy: Indian experience. Ann. Indian Acad. Neurol. 19 , 523–527 (2016).
Sondhi, V. et al. Efficacy of ketogenic diet, modified Atkins diet, and low glycemic index therapy diet among children with drug-resistant epilepsy: a randomized clinical trial. JAMA Pediatr. 174 , 944–951 (2020).
Pfeifer, H. H. & Thiele, E. A. Low-glycemic-index treatment: a liberalized ketogenic diet for treatment of intractable epilepsy. Neurology 65 , 1810–1812 (2005).
Muzykewicz, D. A. et al. Efficacy, safety, and tolerability of the low glycemic index treatment in pediatric epilepsy. Epilepsia 50 , 1118–1126 (2009).
Coppola, G. et al. Low glycemic index diet in children and young adults with refractory epilepsy: first Italian experience. Seizure 20 , 526–528 (2011).
Karimzadeh, P. et al. Low glycemic index treatment in pediatric refractory epilepsy: the first Middle East report. Seizure 23 , 570–572 (2014).
Kim, S. H. et al. Low glycemic index treatment in patients with drug-resistant epilepsy. Brain Dev. 39 , 687–692 (2017).
Paoli, A. Ketogenic diet for obesity: friend or foe? Int. J. Environ. Res. Public Health 11 , 2092–2107 (2014).
Crabtree, C. D. et al. Comparison of ketogenic diets with and without ketone salts versus a low-fat diet: liver fat responses in overweight adults. Nutrients 13 , 966 (2021).
Tragni, E. et al. Reduction of cardio-metabolic risk and body weight through a multiphasic very-low calorie ketogenic diet program in women with overweight/obesity: a study in a real-world setting. Nutrients 13 , 1804 (2021).
Alarim, R. A. et al. Effects of the ketogenic diet on glycemic control in diabetic patients: meta-analysis of clinical trials. Cureus 12 , e10796 (2020).
PubMed PubMed Central Google Scholar
Mardinoglu, A. et al. An integrated understanding of the rapid metabolic benefits of a carbohydrate-restricted diet on hepatic steatosis in humans. Cell Metab. 27 , 559–571 e555 (2018).
Luukkonen, P. K. et al. Effect of a ketogenic diet on hepatic steatosis and hepatic mitochondrial metabolism in nonalcoholic fatty liver disease. Proc. Natl Acad. Sci. USA 117 , 7347–7354 (2020).
Volek, J. S., LaFountain, R. A. & Dituro, P. Extended ketogenic diet and physical training intervention in military personnel. Mil. Med. 184 , 199–200 (2019).
Hyde, P. N. et al. Dietary carbohydrate restriction improves metabolic syndrome independent of weight loss. JCI Insight 4 , e128308 (2019).
Article PubMed Central Google Scholar
Domouzoglou, E. M. & Maratos-Flier, E. Fibroblast growth factor 21 is a metabolic regulator that plays a role in the adaptation to ketosis. Am. J. Clin. Nutr. 93 , 901s–905s (2011).
Volek, J. S., Sharman, M. J. & Forsythe, C. E. Modification of lipoproteins by very low-carbohydrate diets. J. Nutr. 135 , 1339–1342 (2005).
Veldhorst, M. A., Westerterp-Plantenga, M. S. & Westerterp, K. R. Gluconeogenesis and energy expenditure after a high-protein, carbohydrate-free diet. Am. J. Clin. Nutr. 90 , 519–526 (2009).
Schutz, Y. Protein turnover, ureagenesis and gluconeogenesis. Int. J. Vitam. Nutr. Res. 81 , 101–107 (2011).
Dienel, G. A. Brain glucose metabolism: integration of energetics with function. Physiol. Rev. 99 , 949–1045 (2019).
Owen, O. E. Ketone bodies as a fuel for the brain during starvation. Biochem. Mol. Biol. Educ. 33 , 246–251 (2005).
Bough, K. J. & Rho, J. M. Anticonvulsant mechanisms of the ketogenic diet. Epilepsia 48 , 43–58 (2007).
Holland, A. M. et al. Effects of a ketogenic diet on adipose tissue, liver, and serum biomarkers in sedentary rats and rats that exercised via resisted voluntary wheel running. Am. J. Physiol. Regul. Integr. Comp. Physiol. 311 , R337–351 (2016).
Nanri, A. et al. Low-carbohydrate diet and type 2 diabetes risk in Japanese men and women: the Japan Public Health Center-Based Prospective Study. PLoS ONE 10 , e0118377 (2015).
Delahanty, L. M. et al. Association of diet with glycated hemoglobin during intensive treatment of type 1 diabetes in the diabetes control and complications trial. Am. J. Clin. Nutr. 89 , 518–524 (2009).
Ellenbroek, J. H. et al. Long-term ketogenic diet causes glucose intolerance and reduced β- and α-cell mass but no weight loss in mice. Am. J. Physiol. Endocrinol. Metab. 306 , E552–558 (2014).
McDonald, T. J. W. & Cervenka, M. C. The expanding role of ketogenic diets in adult neurological disorders. Brain Sci 8 , 148 (2018).
Newman, J. C. & Verdin, E. Ketone bodies as signaling metabolites. Trends Endocrinol. Metab. 25 , 42–52 (2014).
Veyrat-Durebex, C. et al. How can a ketogenic diet improve motor function? Front. Mol. Neurosci. 11 , 15 (2018).
Achanta, L. B. & Rae, C. D. β-hydroxybutyrate in the brain: one molecule, multiple mechanisms. Neurochem Res. 42 , 35–49 (2017).
McCue, M. D. Starvation physiology: reviewing the different strategies animals use to survive a common challenge. Comp. Biochem. Physiol. A Mol. Integr. Physiol. 156 , 1–18 (2010).
Paoli, A., Bianco, A., Damiani, E. & Bosco, G. Ketogenic diet in neuromuscular and neurodegenerative diseases. Biomed. Res. Int. 2014 , 474296 (2014).
Veech, R. L. et al. Ketone bodies, potential therapeutic uses. IUBMB Life 51 , 241–247 (2001).
Elamin, M., Ruskin, D. N., Masino, S. A. & Sacchetti, P. Ketone-based metabolic therapy: is increased NAD(+) a primary mechanism? Front. Mol. Neurosci. 10 , 377 (2017).
Cullingford, T. E. The ketogenic diet; fatty acids, fatty acid-activated receptors and neurological disorders. Prostaglandins Leukot. Ess. Fat. Acids 70 , 253–264 (2004).
Olson, C. A. et al. The gut microbiota mediates the anti-seizure effects of the ketogenic diet. Cell 173 , 1728–1741.e1713 (2018).
Whitfield, J. B. Gamma glutamyl transferase. Crit. Rev. Clin. Lab Sci. 38 , 263–355 (2001).
Ma, D. et al. Ketogenic diet enhances neurovascular function with altered gut microbiome in young healthy mice. Sci. Rep. 8 , 6670 (2018).
Newell, C. et al. Ketogenic diet modifies the gut microbiota in a murine model of autism spectrum disorder. Mol. Autism 7 , 37 (2016).
Swidsinski, A. et al. Reduced mass and diversity of the colonic microbiome in patients with multiple sclerosis and their improvement with ketogenic diet. Front Microbiol. 8 , 1141 (2017).
Xie, G. et al. Ketogenic diet poses a significant effect on imbalanced gut microbiota in infants with refractory epilepsy. World J. Gastroenterol. 23 , 6164–6171 (2017).
Arumugam, M. et al. Enterotypes of the human gut microbiome. Nature 473 , 174–180 (2011).
Zhang, Y. et al. Altered gut microbiome composition in children with refractory epilepsy after ketogenic diet. Epilepsy Res. 145 , 163–168 (2018).
Olson, C. A. et al. Alterations in the gut microbiota contribute to cognitive impairment induced by the ketogenic diet and hypoxia. Cell Host Microbe 29 , 1378–1392 (2021).
Newman, J. C. et al. Ketogenic diet reduces midlife mortality and improves memory in aging mice. Cell Metab. 26 , 547–557.e548 (2017).
Roberts, M. N. et al. A ketogenic diet extends longevity and healthspan in adult mice. Cell Metab. 26 , 539–546.e535 (2017).
Kov cs, Z., Brunner, B. & Ari, C. Beneficial effects of exogenous ketogenic supplements on aging processes and age-related neurodegenerative diseases. Nutrients 13 , 2197 (2021).
American Diabetes, A. 2. Classification and diagnosis of diabetes. Diabetes Care 40 , S11–S24 (2017).
American Diabetes, A. et al. Nutrition recommendations and interventions for diabetes: a position statement of the American Diabetes Association. Diabetes Care. 31 (Suppl 1), S61–78 (2008).
Rizza, R. A. Pathogenesis of fasting and postprandial hyperglycemia in type 2 diabetes: implications for therapy. Diabetes 59 , 2697–2707 (2010).
Gannon, M. C., Hoover, H. & Nuttall, F. Q. Further decrease in glycated hemoglobin following ingestion of a LoBAG30 diet for 10 weeks compared to 5 weeks in people with untreated type 2 diabetes. Nutr. Metab. 7 , 64 (2010).
Nuttall, F. Q., Schweim, K., Hoover, H. & Gannon, M. C. Effect of the LoBAG30 diet on blood glucose control in people with type 2 diabetes. Br. J. Nutr. 99 , 511–519 (2008).
Dashti, H. M. et al. Beneficial effects of ketogenic diet in obese diabetic subjects. Mol. Cell Biochem. 302 , 249–256 (2007).
Hussain, T. A. et al. Effect of low-calorie versus low-carbohydrate ketogenic diet in type 2 diabetes. Nutrition 28 , 1016–1021 (2012).
Rafiullah, M., Musambil, M. & David, S. K. Effect of a very low-carbohydrate ketogenic diet vs recommended diets in patients with type 2 diabetes: a meta-analysis. Nutr. Rev. https://doi.org/10.1093/nutrit/nuab040 (2021).
Tay, J. et al. Comparison of low- and high-carbohydrate diets for type 2 diabetes management: a randomized trial. Am. J. Clin. Nutr. 102 , 780–790 (2015).
Kinzig, K. P., Honors, M. A. & Hargrave, S. L. Insulin sensitivity and glucose tolerance are altered by maintenance on a ketogenic diet. Endocrinology 151 , 3105–3114 (2010).
Cox, N. et al. Ketogenic diets potentially reverse type II diabetes and ameliorate clinical depression: a case study. Diabetes Metab. Syndr. 13 , 1475–1479 (2019).
Cohen, C. W. et al. A ketogenic diet reduces central obesity and serum insulin in women with ovarian or endometrial cancer. J. Nutr. 148 , 1253–1260 (2018).
Yuan, X. et al. Effect of the ketogenic diet on glycemic control, insulin resistance, and lipid metabolism in patients with T2DM: a systematic review and meta-analysis. Nutr. Diabetes 10 , 38–38 (2020).
Farrés, J. et al. Revealing the molecular relationship between type 2 diabetes and the metabolic changes induced by a very-low-carbohydrate low-fat ketogenic diet. Nutr. Metab. 7 , 88–88 (2010).
Okuda, T., Fukui, A. & Morita, N. Altered expression of O-GlcNAc-modified proteins in a mouse model whose glycemic status is controlled by a low carbohydrate ketogenic diet. Glycoconj. J. 30 , 781–789 (2013).
Okuda, T. & Morita, N. A very low carbohydrate ketogenic diet prevents the progression of hepatic steatosis caused by hyperglycemia in a juvenile obese mouse model. Nutr. Diabetes 2 , e50 (2012).
Zhang, Q. et al. Treatment of diabetic mice with a combination of ketogenic diet and aerobic exercise via modulations of PPARs gene programs. PPAR Res. 2018 , 4827643 (2018).
Olson, A. L. & Pessin, J. E. Structure, function, and regulation of the mammalian facilitative glucose transporter gene family. Annu Rev. Nutr. 16 , 235–256 (1996).
Xu, J. et al. Fibroblast growth factor 21 reverses hepatic steatosis, increases energy expenditure, and improves insulin sensitivity in diet-induced obese mice. Diabetes 58 , 250–259 (2009).
Taggart, A. K. et al. D)-beta-Hydroxybutyrate inhibits adipocyte lipolysis via the nicotinic acid receptor PUMA-G. J. Biol. Chem 280 , 26649–26652 (2005).
Jin, W. & Patti, M. E. Genetic determinants and molecular pathways in the pathogenesis of Type 2 diabetes. Clin. Sci. (Lond.). 116 , 99–111 (2009).
Kalra, S., Jain, A., Ved, J. & Unnikrishnan, A. G. Sodium-glucose cotransporter 2 inhibition and health benefits: The Robin Hood effect. Indian J. Endocrinol. Metab. 20 , 725–729 (2016).
Feinman, R. D. et al. Dietary carbohydrate restriction as the first approach in diabetes management: critical review and evidence base. Nutrition 31 , 1–13 (2015).
Castaldo, G. et al. Aggressive nutritional strategy in morbid obesity in clinical practice: safety, feasibility, and effects on metabolic and haemodynamic risk factors. Obes. Res. Clin. Pract. 10 , 169–177 (2016).
Dashti, H. M. et al. Long-term effects of a ketogenic diet in obese patients. Exp. Clin. Cardiol. 9 , 200–205 (2004).
CAS PubMed PubMed Central Google Scholar
Mansoor, N., Vinknes, K. J., Veierod, M. B. & Retterstol, K. Effects of low-carbohydrate diets v. low-fat diets on body weight and cardiovascular risk factors: a meta-analysis of randomised controlled trials. Br. J. Nutr. 115 , 466–479 (2016).
Bueno, N. B., de Melo, I. S., de Oliveira, S. L. & da Rocha Ataide, T. Very-low-carbohydrate ketogenic diet v. low-fat diet for long-term weight loss: a meta-analysis of randomised controlled trials. Br. J. Nutr. 110 , 1178–1187 (2013).
Choi, Y. J., Jeon, S.-M. & Shin, S. Impact of a ketogenic diet on metabolic parameters in patients with obesity or overweight and with or without type 2 diabetes: a meta-analysis of randomized controlled trials. Nutrients 12 , 2005 (2020).
Paoli, A., Rubini, A., Volek, J. S. & Grimaldi, K. A. Beyond weight loss: a review of the therapeutic uses of very-low-carbohydrate (ketogenic) diets. Eur. J. Clin. Nutr. 67 , 789–796 (2013).
Westerterp-Plantenga, M. S. et al. Dietary protein, weight loss, and weight maintenance. Annu. Rev. Nutr. 29 , 21–41 (2009).
Veldhorst, M. et al. Protein-induced satiety: effects and mechanisms of different proteins. Physiol. Behav. 94 , 300–307 (2008).
Sumithran, P. et al. Ketosis and appetite-mediating nutrients and hormones after weight loss. Eur. J. Clin. Nutr. 67 , 759–764 (2013).
Johnstone, A. M. et al. Effects of a high-protein ketogenic diet on hunger, appetite, and weight loss in obese men feeding ad libitum. Am. J. Clin. Nutr. 87 , 44–55 (2008).
Laeger, T., Metges, C. C. & Kuhla, B. Role of beta-hydroxybutyric acid in the central regulation of energy balance. Appetite 54 , 450–455 (2010).
Yang, Z. et al. Effects of low-carbohydrate diet and ketogenic diet on glucose and lipid metabolism in type 2 diabetic mice. Nutrition 89 , 111230 (2021).
Ma, S. et al. An 8-week ketogenic diet alternated interleukin-6, ketolytic and lipolytic gene expression, and enhanced exercise capacity in mice. Nutrients 10 , 1696 (2018).
Tagliabue, A. et al. Effects of the ketogenic diet on nutritional status, resting energy expenditure, and substrate oxidation in patients with medically refractory epilepsy: a 6-month prospective observational study. Clin. Nutr. 31 , 246–249 (2012).
Paoli, A. et al. Medium term effects of a ketogenic diet and a Mediterranean diet on resting energy expenditure and respiratory ratio. BMC Proc. 6 , P37 (2012).
Fine, E. J. & Feinman, R. D. Thermodynamics of weight loss diets. Nutr. Metab. 1 , 15 (2004).
Feinman, R. D. & Fine, E. J. Nonequilibrium thermodynamics and energy efficiency in weight loss diets. Theor. Biol. Med. Model. 4 , 27 (2007).
Volek, J. S. et al. Carbohydrate restriction has a more favorable impact on the metabolic syndrome than a low fat diet. Lipids 44 , 297–309 (2009).
Davidson, T. L. et al. Inter-relationships among diet, obesity and hippocampal-dependent cognitive function. Neuroscience 253 , 110–122 (2013).
Brinkworth, G. D., Noakes, M., Clifton, P. M. & Buckley, J. D. Effects of a low carbohydrate weight loss diet on exercise capacity and tolerance in obese subjects. Obesity 17 , 1916–1923 (2009).
Yancy, W. S. Jr. et al. Effects of two weight-loss diets on health-related quality of life. Qual. Life Res. 18 , 281–289 (2009).
McDaniel, S. S. et al. The ketogenic diet inhibits the mammalian target of rapamycin (mTOR) pathway. Epilepsia 52 , e7–11 (2011).
Freedland, S. J. et al. Carbohydrate restriction, prostate cancer growth, and the insulin-like growth factor axis. Prostate 68 , 11–19 (2008).
Srivastava, S. et al. Mitochondrial biogenesis and increased uncoupling protein 1 in brown adipose tissue of mice fed a ketone ester diet. FASEB J. 26 , 2351–2362 (2012).
Fabbrini, E., Sullivan, S. & Klein, S. Obesity and nonalcoholic fatty liver disease: biochemical, metabolic, and clinical implications. Hepatology 51 , 679–689 (2010).
Tiniakos, D. G., Vos, M. B. & Brunt, E. M. Nonalcoholic fatty liver disease: pathology and pathogenesis. Annu. Rev. Pathol. 5 , 145–171 (2010).
Haghighatdoost, F., Salehi-Abargouei, A., Surkan, P. J. & Azadbakht, L. The effects of low carbohydrate diets on liver function tests in nonalcoholic fatty liver disease: a systematic review and meta-analysis of clinical trials. J. Res. Med. Sci. 21 , 53 (2016).
Browning, J. D. et al. Short-term weight loss and hepatic triglyceride reduction: evidence of a metabolic advantage with dietary carbohydrate restriction. Am. J. Clin. Nutr. 93 , 1048–1052 (2011).
Foster, G. D. et al. Weight and metabolic outcomes after 2 years on a low-carbohydrate versus low-fat diet: a randomized trial. Ann. Intern. Med. 153 , 147–157 (2010).
Watanabe, M. et al. Beneficial effects of the ketogenic diet on nonalcoholic fatty liver disease: a comprehensive review of the literature. Obes. Rev. 21 , e13024 (2020).
Paoli, A. et al. Long term successful weight loss with a combination biphasic ketogenic Mediterranean diet and Mediterranean diet maintenance protocol. Nutrients 5 , 5205–5217 (2013).
Gibson, A. A. et al. Do ketogenic diets really suppress appetite? A systematic review and meta-analysis. Obes. Rev. 16 , 64–76 (2015).
Shimazu, T. et al. Suppression of oxidative stress by beta-hydroxybutyrate, an endogenous histone deacetylase inhibitor. Science 339 , 211–214 (2013).
Graff, E. C., Fang, H., Wanders, D. & Judd, R. L. Anti-inflammatory effects of the hydroxycarboxylic acid receptor 2. Metabolism 65 , 102–113 (2016).
Youm, Y.-H. et al. The ketone metabolite β-hydroxybutyrate blocks NLRP3 inflammasome–mediated inflammatory disease. Nat. Med. 21 , 263–269 (2015).
Vandanmagsar, B. et al. The NLRP3 inflammasome instigates obesity-induced inflammation and insulin resistance. Nat. Med. 17 , 179–188 (2011).
Mavropoulos, J. C., Yancy, W. S., Hepburn, J. & Westman, E. C. The effects of a low-carbohydrate, ketogenic diet on the polycystic ovary syndrome: a pilot study. Nutr. Metab. 2 , 35 (2005).
Gower, B. A. et al. Favourable metabolic effects of a eucaloric lower-carbohydrate diet in women with PCOS. Clin. Endocrinol. 79 , 550–557 (2013).
Paoli, A. et al. Effects of a ketogenic diet in overweight women with polycystic ovary syndrome. J. Transl. Med. 18 , 104 (2020).
Burghen, G. A., Givens, J. R. & Kitabchi, A. E. Correlation of hyperandrogenism with hyperinsulinism in polycystic ovarian disease. J. Clin. Endocrinol. Metab. 50 , 113–116 (1980).
Nestler, J. E. et al. Insulin stimulates testosterone biosynthesis by human thecal cells from women with polycystic ovary syndrome by activating its own receptor and using inositolglycan mediators as the signal transduction system. J. Clin. Endocrinol. Metab. 83 , 2001–2005 (1998).
CAS PubMed Google Scholar
Cabrera-Cruz, H. et al. The insulin-sensitizing mechanism of myo-inositol is associated with AMPK activation and GLUT-4 expression in human endometrial cells exposed to a PCOS environment. Am. J. Physiol. Endocrinol. Metab. 318 , E237–e248 (2020).
Soria Lopez, J. A., Gonzalez, H. M. & Leger, G. C. Alzheimer’s disease. Handb. Clin. Neurol. 167 , 231–255 (2019).
Macdonald, R., Barnes, K., Hastings, C. & Mortiboys, H. Mitochondrial abnormalities in Parkinson’s disease and Alzheimer’s disease: can mitochondria be targeted therapeutically? Biochem. Soc. Trans. 46 , 891–909 (2018).
Omar, S. H. Mediterranean and MIND diets containing olive biophenols reduces the prevalence of Alzheimer’s disease. Int. J. Mol. Sci . 20 , 2797 (2019).
McDonald, T. J. W. & Cervenka, M. C. Ketogenic diets for adult neurological disorders. Neurotherapeutics 15 , 1018–1031 (2018).
Broom, G. M., Shaw, I. C. & Rucklidge, J. J. The ketogenic diet as a potential treatment and prevention strategy for Alzheimer’s disease. Nutrition 60 , 118–121 (2019).
Kashiwaya, Y. et al. D-beta-hydroxybutyrate protects neurons in models of Alzheimer’s and Parkinson’s disease. Proc. Natl Acad. Sci. USA 97 , 5440–5444 (2000).
Costantini, L. C., Barr, L. J., Vogel, J. L. & Henderson, S. T. Hypometabolism as a therapeutic target in Alzheimer’s disease. BMC Neurosci. 9 (Suppl 2), S16 (2008).
Johri, A. & Beal, M. F. Mitochondrial dysfunction in neurodegenerative diseases. J. Pharm. Exp. Ther. 342 , 619–630 (2012).
Pinto, A. et al. Anti-oxidant and anti-inflammatory activity of ketogenic diet: new perspectives for neuroprotection in Alzheimer’s disease. Antioxidants 7 , 63 (2018).
Holmes, C. Review: systemic inflammation and Alzheimer’s disease. Neuropathol. Appl. Neurobiol. 39 , 51–68 (2013).
Castellano, C. A. et al. Lower brain 18F-fluorodeoxyglucose uptake but normal 11C-acetoacetate metabolism in mild Alzheimer’s disease dementia. J. Alzheimers Dis. 43 , 1343–1353 (2015).
Cunnane, S. C. et al. Can ketones compensate for deteriorating brain glucose uptake during aging? Implications for the risk and treatment of Alzheimer’s disease. Ann. N. Y Acad. Sci. 1367 , 12–20 (2016).
Rodell, A. et al. Natural selection of mitochondria during somatic lifetime promotes healthy aging. Front. Neuroenergetics. 5 , 7 (2013).
Hughes, S. D. et al. The ketogenic diet component decanoic acid increases mitochondrial citrate synthase and complex I activity in neuronal cells. J. Neurochem. 129 , 426–433 (2014).
Kim, D. Y. et al. Ketone bodies mediate antiseizure effects through mitochondrial permeability transition. Ann. Neurol. 78 , 77–87 (2015).
Bough, K. J. et al. Mitochondrial biogenesis in the anticonvulsant mechanism of the ketogenic diet. Ann. Neurol. 60 , 223–235 (2006).
Lu, Y. et al. Ketogenic diet attenuates oxidative stress and inflammation after spinal cord injury by activating Nrf2 and suppressing the NF-kappaB signaling pathways. Neurosci. Lett. 683 , 13–18 (2018).
Rahman, M. et al. The beta-hydroxybutyrate receptor HCA2 activates a neuroprotective subset of macrophages. Nat. Commun. 5 , 3944 (2014).
Dupuis, N., Curatolo, N., Benoist, J. F. & Auvin, S. Ketogenic diet exhibits anti-inflammatory properties. Epilepsia 56 , e95–98 (2015).
Peixoto, L. & Abel, T. The role of histone acetylation in memory formation and cognitive impairments. Neuropsychopharmacology 38 , 62–76 (2013).
Yudkoff, M. et al. Response of brain amino acid metabolism to ketosis. Neurochem. Int. 47 , 119–128 (2005).
Kashiwaya, Y. et al. A ketone ester diet exhibits anxiolytic and cognition-sparing properties, and lessens amyloid and tau pathologies in a mouse model of Alzheimer’s disease. Neurobiol. Aging 34 , 1530–1539 (2013).
Van der Auwera, I., Wera, S., Van Leuven, F. & Henderson, S. T. A ketogenic diet reduces amyloid beta 40 and 42 in a mouse model of Alzheimer’s disease. Nutr. Metab. 2 , 28 (2005).
Studzinski, C. M. et al. Induction of ketosis may improve mitochondrial function and decrease steady-state amyloid-beta precursor protein (APP) levels in the aged dog. Brain Res. 1226 , 209–217 (2008).
Xu, K. et al. Diet-induced ketosis improves cognitive performance in aged rats. Adv. Exp. Med. Biol. 662 , 71–75 (2010).
Reger, M. A. et al. Effects of beta-hydroxybutyrate on cognition in memory-impaired adults. Neurobiol. Aging 25 , 311–314 (2004).
Phillips, M. C. L. et al. Randomized crossover trial of a modified ketogenic diet in Alzheimer’s disease. Alzheimers Res. Ther. 13 , 51 (2021).
Krikorian, R. et al. Dietary ketosis enhances memory in mild cognitive impairment. Neurobiol. Aging 33 , 425 e419–427 (2012).
Rebello, C. J. et al. Pilot feasibility and safety study examining the effect of medium chain triglyceride supplementation in subjects with mild cognitive impairment: a randomized controlled trial. BBA Clin. 3 , 123–125 (2015).
Samii, A., Nutt, J. G. & Ransom, B. R. Parkinson’s disease. Lancet 363 , 1783–1793 (2004).
Anwar, S. et al. Functional alterations to the nigrostriatal system in mice lacking all three members of the synuclein family. J. Neurosci. 31 , 7264–7274 (2011).
Joniec-Maciejak, I. et al. Octanoic acid prevents reduction of striatal dopamine in the MPTP mouse model of Parkinson’s disease. Pharm. Rep. 70 , 988–992 (2018).
Zecca, L. et al. Interaction of neuromelanin and iron in substantia nigra and other areas of human brain. Neuroscience 73 , 407–415 (1996).
Gorell, J. et al. Increased iron-related MRI contrast in the substantia nigra in Parkinson’s disease. Neurology 45 , 1138–1143 (1995).
Martuscello, R. et al. A supplemented high-fat low-carbohydrate diet for the treatment of glioblastoma. Clin. Cancer Res. 22 , 2482–2495 (2016).
Yang, X. & Cheng, B. Neuroprotective and anti-inflammatory activities of ketogenic diet on MPTP-induced neurotoxicity. J. Mol. Neurosci. 42 , 145–153 (2010).
Cheng, B. et al. D-beta-hydroxybutyrate inhibits the apoptosis of PC12 cells induced by 6-OHDA in relation to up-regulating the ratio of Bcl-2/Bax mRNA. Auton. Neurosci. 134 , 38–44 (2007).
Cheng, B. et al. Ketogenic diet protects dopaminergic neurons against 6-OHDA neurotoxicity via up-regulating glutathione in a rat model of Parkinson’s disease. Brain Res. 1286 , 25–31 (2009).
Tieu, K. et al. D-beta-hydroxybutyrate rescues mitochondrial respiration and mitigates features of Parkinson disease. J. Clin. Investig. 112 , 892–901 (2003).
Shaafi, S. et al. The efficacy of the ketogenic diet on motor functions in Parkinson’s disease: a rat model. Iran. J. Neurol. 15 , 63–69 (2016).
Vanitallie, T. B. et al. Treatment of Parkinson disease with diet-induced hyperketonemia: a feasibility study. Neurology 64 , 728–730 (2005).
Phillips, M. C. L. et al. Low-fat versus ketogenic diet in Parkinson’s disease: a pilot randomized controlled trial. Mov. Disord. 33 , 1306–1314 (2018).
Caplliure-Llopis, J. et al. Therapeutic alternative of the ketogenic Mediterranean diet to improve mitochondrial activity in amyotrophic lateral sclerosis (ALS): a comprehensive review. Food Sci. Nutr. 8 , 23–35 (2020).
Yun, Y. & Ha, Y. CRISPR/Cas9-mediated gene correction to understand ALS. Int. J. Mol. Sci 21 , 3801 (2020).
Miller, R. G., Mitchell, J. D., Lyon, M. & Moore, D. H. Riluzole for amyotrophic lateral sclerosis (ALS)/motor neuron disease (MND). Cochrane Database Syst. Rev. CD001447 (2007).
Kong, G. et al. The ketone metabolite beta-hydroxybutyrate attenuates oxidative stress in spinal cord injury by suppression of class i histone deacetylases. J. Neurotrauma 34 , 2645–2655 (2017).
Rosen, D. Mutations in Cu/Zn superoxide dismutase gene are associated with familial amyotrophic lateral sclerosis. Nature 364 , 362 (1993).
Zhao, Z. et al. A ketogenic diet as a potential novel therapeutic intervention in amyotrophic lateral sclerosis. BMC Neurosci. 7 , 29 (2006).
Ari, C. et al. Metabolic therapy with Deanna Protocol supplementation delays disease progression and extends survival in amyotrophic lateral sclerosis (ALS) mouse model. PLoS ONE 9 , e103526 (2014).
Zhao, W. et al. Caprylic triglyceride as a novel therapeutic approach to effectively improve the performance and attenuate the symptoms due to the motor neuron loss in ALS disease. PLoS ONE 7 , e49191 (2012).
Singh, A. & Trevick, S. The epidemiology of global epilepsy. Neurol. Clin. 34 , 837–847 (2016).
Thurman, D. J. et al. The primary prevention of epilepsy: a report of the Prevention Task Force of the International League Against Epilepsy. Epilepsia 59 , 905–914 (2018).
Martin, K., Jackson, C. F., Levy, R. G. & Cooper, P. N. Ketogenic diet and other dietary treatments for epilepsy. Cochrane Database Syst. Rev. 2 , CD001903 (2016).
Weinshenker, D. The contribution of norepinephrine and orexigenic neuropeptides to the anticonvulsant effect of the ketogenic diet. Epilepsia 49 (Suppl 8), 104–107 (2008).
Dahlin, M., Mansson, J. E. & Amark, P. CSF levels of dopamine and serotonin, but not norepinephrine, metabolites are influenced by the ketogenic diet in children with epilepsy. Epilepsy Res. 99 , 132–138 (2012).
Calderon, N., Betancourt, L., Hernandez, L. & Rada, P. A ketogenic diet modifies glutamate, gamma-aminobutyric acid and agmatine levels in the hippocampus of rats: a microdialysis study. Neurosci. Lett. 642 , 158–162 (2017).
Zarnowski, T. et al. Kynurenic acid and neuroprotective activity of the ketogenic diet in the eye. Curr. Med. Chem. 24 , 3547–3558 (2017).
Kim, D. et al. Ketone bodies are protective against oxidative stress in neocortical neurons. J. Neurochem. 101 , 1316–1326 (2007).
Maalouf, M. et al. Ketones inhibit mitochondrial production of reactive oxygen species production following glutamate excitotoxicity by increasing NADH oxidation. Neuroscience 145 , 256–264 (2007).
Yellen, G. Ketone bodies, glycolysis, and KATP channels in the mechanism of the ketogenic diet. Epilepsia 49 (Suppl 8), 80–82 (2008).
Andrews, Z. B., Diano, S. & Horvath, T. L. Mitochondrial uncoupling proteins in the CNS: in support of function and survival. Nat. Rev. Neurosci. 6 , 829–840 (2005).
Ye, F. et al. Efficacy of and patient compliance with a ketogenic diet in adults with intractable epilepsy: a meta-analysis. J. Clin. Neurol. 11 , 26–31 (2015).
Cervenka, M. C. et al. Establishing an adult epilepsy diet center: experience, efficacy and challenges. Epilepsy Behav. 58 , 61–68 (2016).
Zare, M. et al. Modified Atkins diet in adult with refractory epilepsy: a controlled randomized clinical trial. Iran. J. Neurol. 16 , 72–77 (2017).
Kverneland, M. et al. Effect of modified Atkins diet in adults with drug-resistant focal epilepsy: a randomized clinical trial. Epilepsia 59 , 1567–1576 (2018).
Williams, T. J. & Cervenka, M. C. The role for ketogenic diets in epilepsy and status epilepticus in adults. Clin. Neurophysiol. Pract. 2 , 154–160 (2017).
Liu, H. et al. Ketogenic diet for treatment of intractable epilepsy in adults: a meta-analysis of observational studies. Epilepsia Open. 3 , 9–17 (2018).
Pehrson, A. L. & Sanchez, C. Altered gamma-aminobutyric acid neurotransmission in major depressive disorder: a critical review of the supporting evidence and the influence of serotonergic antidepressants. Drug Des. Devel Ther. 9 , 603–624 (2015).
Nagpal, R. et al. Modified Mediterranean-ketogenic diet modulates gut microbiome and short-chain fatty acids in association with Alzheimer’s disease markers in subjects with mild cognitive impairment. EBioMedicine 47 , 529–542 (2019).
Sussman, D., Germann, J. & Henkelman, M. Gestational ketogenic diet programs brain structure and susceptibility to depression & anxiety in the adult mouse offspring. Brain Behav. 5 , e00300 (2015).
Guan, Y. F. et al. Anti-depression effects of ketogenic diet are mediated via the restoration of microglial activation and neuronal excitability in the lateral habenula. Brain Behav. Immun. 88 , 748–762 (2020).
Campbell, I. H. & Campbell, H. Ketosis and bipolar disorder: controlled analytic study of online reports. BJPsych Open 5 , e58 (2019).
DM, I. J. et al. Cognitive and behavioral impact of the ketogenic diet in children and adolescents with refractory epilepsy: a randomized controlled trial. Epilepsy Behav. 60 , 153–157 (2016).
Bandelow, B. & Michaelis, S. Epidemiology of anxiety disorders in the 21st century. Dialogues Clin. Neurosci. 17 , 327–335 (2015).
Forte, N. et al. 2-Deoxy-d-glucose enhances tonic inhibition through the neurosteroid-mediated activation of extrasynaptic GABA receptors. Epilepsia 57 , 1987–2000 (2016).
Erecińska, M., Nelson, D., Daikhin, Y. & Yudkoff, M. Regulation of GABA level in rat brain synaptosomes: fluxes through enzymes of the GABA shunt and effects of glutamate, calcium, and ketone bodies. J. Neurochem. 67 , 2325–2334 (1996).
Rawat, K., Singh, N., Kumari, P. & Saha, L. A review on preventive role of ketogenic diet (KD) in CNS disorders from the gut microbiota perspective. Rev. Neurosci. 32 , 143–157 (2021).
Cheng, C. et al. Ketone body signaling mediates intestinal stem cell homeostasis and adaptation to diet. Cell 178 , 1115–1131.e1115 (2019).
Youm, Y. et al. The ketone metabolite β-hydroxybutyrate blocks NLRP3 inflammasome-mediated inflammatory disease. Nat. Med. 21 , 263–269 (2015).
Olson, C. et al. The gut microbiota mediates the anti-seizure effects of the ketogenic diet. Cell 174 , 497 (2018).
Pavlova, N. N. & Thompson, C. B. The emerging hallmarks of cancer metabolism. Cell Metab. 23 , 27–47 (2016).
Seyfried, T. N. et al. Role of glucose and ketone bodies in the metabolic control of experimental brain cancer. Br. J. Cancer 89 , 1375–1382 (2003).
Hao, G. W. et al. Growth of human colon cancer cells in nude mice is delayed by ketogenic diet with or without omega-3 fatty acids and medium-chain triglycerides. Asian Pac. J. Cancer Prev. 16 , 2061–2068 (2015).
Shang, S. et al. The Beta-Hydroxybutyrate Suppresses the Migration of Glioma Cells by Inhibition of NLRP3 Inflammasome. Cell Mol. Neurobiol. 38 , 1479–1489 (2018).
Ristic, B., Bhutia, Y. D. & Ganapathy, V. Cell-surface G-protein-coupled receptors for tumor-associated metabolites: A direct link to mitochondrial dysfunction in cancer. Biochim Biophys. Acta Rev. Cancer 1868 , 246–257 (2017).
Elangovan, S. et al. The niacin/butyrate receptor GPR109A suppresses mammary tumorigenesis by inhibiting cell survival. Cancer Res. 74 , 1166–1178 (2014).
Hopkins, B. D. et al. Suppression of insulin feedback enhances the efficacy of PI3K inhibitors. Nature 560 , 499–503 (2018).
Xia, S. et al. Prevention of dietary-fat-fueled ketogenesis attenuates BRAF V600E tumor growth. Cell Metab. 25 , 358–373 (2017).
Morscher, R. J. et al. Combination of metronomic cyclophosphamide and dietary intervention inhibits neuroblastoma growth in a CD1-nu mouse model. Oncotarget 7 , 17060–17073 (2016).
Allen, B. G. et al. Ketogenic diets enhance oxidative stress and radio-chemo-therapy responses in lung cancer xenografts. Clin. Cancer Res. 19 , 3905–3913 (2013).
Abdelwahab, M. G. et al. The ketogenic diet is an effective adjuvant to radiation therapy for the treatment of malignant glioma. PLoS ONE 7 , e36197 (2012).
Zahra, A. et al. Consuming a ketogenic diet while receiving radiation and chemotherapy for locally advanced lung cancer and pancreatic cancer: the University of Iowa experience of two phase 1 clinical trials. Radiat. Res. 187 , 743–754 (2017).
Aminzadeh-Gohari, S. et al. A ketogenic diet supplemented with medium-chain triglycerides enhances the anti-tumor and anti-angiogenic efficacy of chemotherapy on neuroblastoma xenografts in a CD1-nu mouse model. Oncotarget 8 , 64728–64744 (2017).
Ferrere, G. et al. Ketogenic diet and ketone bodies enhance the anticancer effects of PD-1 blockade. JCI Insight 6 , e145207 (2021).
Dai, X. et al. Energy status dictates PD-L1 protein abundance and anti-tumor immunity to enable checkpoint blockade. Mol. Cell. 81 , 2317–2331 e2316 (2021).
Poff, A. M. et al. Non-toxic metabolic management of metastatic cancer in VM mice: novel combination of ketogenic diet, ketone supplementation, and hyperbaric oxygen therapy. PLoS ONE 10 , e0127407 (2015).
Magee, B. A., Potezny, N., Rofe, A. M. & Conyers, R. A. The inhibition of malignant cell growth by ketone bodies. Aust. J. Exp. Biol. Med. Sci. 57 , 529–539 (1979).
Poff, A. M., Ari, C., Seyfried, T. N. & D’Agostino, D. P. The ketogenic diet and hyperbaric oxygen therapy prolong survival in mice with systemic metastatic cancer. PLoS ONE 8 , e65522 (2013).
Tan-Shalaby, J. L. et al. Modified Atkins diet in advanced malignancies—final results of a safety and feasibility trial within the Veterans Affairs Pittsburgh Healthcare System. Nutr. Metab. 13 , 52 (2016).
Khodabakhshi, A. et al. Effects of Ketogenic metabolic therapy on patients with breast cancer: a randomized controlled clinical trial. Clin. Nutr. 40 , 751–758 (2021).
Santos, J. G. et al. Efficacy of a ketogenic diet with concomitant intranasal perillyl alcohol as a novel strategy for the therapy of recurrent glioblastoma. Oncol. Lett. 15 , 1263–1270 (2018).
Cohen, C. W. et al. Favorable effects of a ketogenic diet on physical function, perceived energy, and food cravings in women with ovarian or endometrial cancer: a randomized, controlled trial. Nutrients 10 , 1187 (2018).
Jin, R. et al. Dietary fats high in linoleic acids impair anti-tumor T cell responses by inducing E-FABP-mediated mitochondrial dysfunction. Cancer Res. 81 , 5296–5310 (2021).
Aubert, G. et al. The failing heart relies on ketone bodies as a fuel. Circulation 133 , 698–705 (2016).
Bedi, K. C. Jr. et al. Evidence for intramyocardial disruption of lipid metabolism and increased myocardial ketone utilization in advanced human heart failure. Circulation 133 , 706–716 (2016).
Horton, J. L. et al. The failing heart utilizes 3-hydroxybutyrate as a metabolic stress defense. JCI Insight 4 , e124079 (2019).
Nielsen, R. et al. Cardiovascular effects of treatment with the ketone body 3-hydroxybutyrate in chronic heart failure patients. Circulation 139 , 2129–2141 (2019).
McMurray, J. J. V. et al. Dapagliflozin in patients with heart failure and reduced ejection fraction. N. Engl. J. Med. 381 , 1995–2008 (2019).
Zhang, Y. et al. Mitochondrial pyruvate carriers are required for myocardial stress adaptation. Nat. Metab. 2 , 1248–1264 (2020).
Xu, S. et al. Ketogenic diets inhibit mitochondrial biogenesis and induce cardiac fibrosis. Signal Transduct. Target Ther. 6 , 54 (2021).
Mayr, M. et al. Combined metabolomic and proteomic analysis of human atrial fibrillation. J. Am. Coll. Cardiol. 51 , 585–594 (2008).
Obokata, M. et al. Association between circulating ketone bodies and worse outcomes in hemodialysis patients. J. Am. Heart Assoc 6 , e006885 (2017).
Best, T. H. et al. Cardiac complications in pediatric patients on the ketogenic diet. Neurology 54 , 2328–2330 (2000).
Bislev, L. et al. Circulating levels of muscle-related metabolites increase in response to a daily moderately high dose of a vitamin D3 supplement in women with vitamin D insufficiency-secondary analysis of a randomized placebo-controlled trial. Nutrients 12 , 1310 (2020).
Grandl, G. et al. Short-term feeding of a ketogenic diet induces more severe hepatic insulin resistance than an obesogenic high-fat diet. J. Physiol. 596 , 4597–4609 (2018).
Chimienti, G. et al. The ketogenic diet reduces the harmful effects of stress on gut mitochondrial biogenesis in a rat model of irritable bowel syndrome. Int. J. Mol. Sci 22 , 3498 (2021).
Paoli, A., Gorini, S. & Caprio, M. The dark side of the spoon - glucose, ketones and COVID-19: a possible role for ketogenic diet? J. Transl. Med. 18 , 441 (2020).
Soliman, S., Faris, M. E., Ratemi, Z. & Halwani, R. Switching host metabolism as an approach to dampen SARS-CoV-2 infection. Ann. Nutr. Metab. 76 , 297–303 (2020).
Bradshaw, P. C. et al. COVID-19: proposing a ketone-based metabolic therapy as a treatment to blunt the cytokine storm. Oxid. Med. Cell Longev. 2020 , 6401341 (2020).
Sukkar, S. G. & Bassetti, M. Induction of ketosis as a potential therapeutic option to limit hyperglycemia and prevent cytokine storm in COVID-19. Nutrition 79-80 , 110967 (2020).
Sukkar, S. G. et al. Clinical efficacy of eucaloric ketogenic nutrition in the COVID-19 cytokine storm: a retrospective analysis of mortality and intensive care unit admission. Nutrition 89 , 111236 (2021).
Crosby, L. et al. Ketogenic diets and chronic disease: weighing the benefits against the risks. Front. Nutr. 8 , 702802 (2021).
Zarnowska, I. M. Therapeutic use of the ketogenic diet in refractory epilepsy: what we know and what still needs to be learned. Nutrients 12 , 2616 (2020).
Kanikarla-Marie, P. & Jain, S. K. Hyperketonemia and ketosis increase the risk of complications in type 1 diabetes. Free Radic. Biol. Med. 95 , 268–277 (2016).
Jia, P. et al. Ketogenic diet aggravates kidney dysfunction by exacerbating metabolic disorders and inhibiting autophagy in spontaneously hypertensive rats. Biochem Biophys. Res. Commun. 573 , 13–18 (2021).
Guo, Y. et al. Ketogenic diet aggravates hypertension via NF-kappaB-mediated endothelial dysfunction in spontaneously hypertensive rats. Life Sci. 258 , 118124 (2020).
You, Y. et al. Ketogenic diet aggravates cardiac remodeling in adult spontaneously hypertensive rats. Nutr. Metab. 17 , 91 (2020).
Park, S., Zhang, T., Wu, X. & Yi Qiu, J. Ketone production by ketogenic diet and by intermittent fasting has different effects on the gut microbiota and disease progression in an Alzheimer’s disease rat model. J. Clin. Biochem. Nutr. 67 , 188–198 (2020).
Kuter, K. Z., Olech, L., Glowacka, U. & Paleczna, M. Increased beta-hydroxybutyrate level is not sufficient for the neuroprotective effect of long-term ketogenic diet in an animal model of early parkinson’s disease. exploration of brain and liver energy metabolism markers. Int. J. Mol. Sci 22 , 7556 (2021).
Howrie, D. L. et al. The ketogenic diet: the need for a multidisciplinary approach. Ann. Pharmacother. 32 , 384–385 (1998).
Vallejo, et al. The contribution of ketone bodies to glycolytic inhibition for the treatment of adult and pediatric glioblastoma. J Neurooncol . 147 , 317–326 (2020).
Download references
Author information
These authors contributed equally: Huiyuan Zhu, Dexi Bi, Youhua Zhang, Cheng Kong
Authors and Affiliations
Department of Pathology, Shanghai Tenth People’s Hospital, Tongji University School of Medicine, Shanghai, China
Huiyuan Zhu, Dexi Bi, Youhua Zhang & Qing Wei
Research Institute of Intestinal Diseases, Tongji University School of Medicine, Shanghai, China
Cheng Kong, Jiahao Du, Xiawei Wu & Huanlong Qin
Department of Gastrointestinal Surgery, Shanghai Tenth People’s Hospital, Tongji University School of Medicine, Shanghai, China
Cheng Kong & Huanlong Qin
Shanghai Clinical College, Anhui Medical University, Hefei, China
You can also search for this author in PubMed Google Scholar
Corresponding authors
Correspondence to Qing Wei or Huanlong Qin .
Ethics declarations
Competing interests.
The authors declare no competing interests.
Rights and permissions
Open Access This article is licensed under a Creative Commons Attribution 4.0 International License, which permits use, sharing, adaptation, distribution and reproduction in any medium or format, as long as you give appropriate credit to the original author(s) and the source, provide a link to the Creative Commons license, and indicate if changes were made. The images or other third party material in this article are included in the article’s Creative Commons license, unless indicated otherwise in a credit line to the material. If material is not included in the article’s Creative Commons license and your intended use is not permitted by statutory regulation or exceeds the permitted use, you will need to obtain permission directly from the copyright holder. To view a copy of this license, visit http://creativecommons.org/licenses/by/4.0/ .
Reprints and permissions
About this article
Cite this article.
Zhu, H., Bi, D., Zhang, Y. et al. Ketogenic diet for human diseases: the underlying mechanisms and potential for clinical implementations. Sig Transduct Target Ther 7 , 11 (2022). https://doi.org/10.1038/s41392-021-00831-w
Download citation
Received : 06 July 2021
Revised : 21 October 2021
Accepted : 09 November 2021
Published : 17 January 2022
DOI : https://doi.org/10.1038/s41392-021-00831-w
Share this article
Anyone you share the following link with will be able to read this content:
Sorry, a shareable link is not currently available for this article.
Provided by the Springer Nature SharedIt content-sharing initiative
This article is cited by
Effects of dietary intervention on human diseases: molecular mechanisms and therapeutic potential.
- Yu-Ling Xiao
- Yi-Zhou Jiang
Signal Transduction and Targeted Therapy (2024)
Autism spectrum disorders and the gastrointestinal tract: insights into mechanisms and clinical relevance
- Lin Y. Hung
- Kara Gross Margolis
Nature Reviews Gastroenterology & Hepatology (2024)
The growing popularity of low-carb cereal-based products: the lay of the land
- Nicola Gasparre
- Cristina M. Rosell
- Fatma Boukid
European Food Research and Technology (2024)
Does a Ketogenic Diet Have a Place Within Diabetes Clinical Practice? Review of Current Evidence and Controversies
- Chloe H. Firman
- Duane D. Mellor
- Adrian Brown
Diabetes Therapy (2024)
Glucose metabolic reprogramming and its therapeutic potential in obesity-associated endometrial cancer
- Pengzhu Huang
- Xiangqin Fan
- Fengxia Xue
Journal of Translational Medicine (2023)
Quick links
- Explore articles by subject
- Guide to authors
- Editorial policies

Search form
Keto diet works best in small doses, yale researchers find.

(© stock.adobe.com)
A ketogenic diet — which provides 99% of calories from fat and protein and only 1% from carbohydrates — produces health benefits in the short term, but negative effects after about a week, Yale researchers found in a study of mice.
The results offer early indications that the keto diet could, over limited time periods, improve human health by lowering diabetes risk and inflammation. They also represent an important first step toward possible clinical trials in humans.
The keto diet has become increasingly popular as celebrities, including Gwyneth Paltrow, Lebron James, and Kim Kardashian, have touted it as a weight-loss regimen.
In the Yale study, published in the Jan. 20 issue of Nature Metabolism , researchers found that the positive and negative effects of the diet both relate to immune cells called gamma delta T-cells, tissue-protective cells that lower diabetes risk and inflammation.
A keto diet tricks the body into burning fat, said lead author Vishwa Deep Dixit of the Yale School of Medicine. When the body’s glucose level is reduced due to the diet’s low carbohydrate content, the body acts as if it is in a starvation state — although it is not — and begins burning fats instead of carbohydrates. This process in turn yields chemicals called ketone bodies as an alternative source of fuel. When the body burns ketone bodies, tissue-protective gamma delta T-cells expand throughout the body.
This reduces diabetes risk and inflammation, and improves the body’s metabolism, said Dixit, the Waldemar Von Zedtwitz Professor of Comparative Medicine and of Immunobiology. After a week on the keto diet, he said, mice show a reduction in blood sugar levels and inflammation.
But when the body is in this “starving-not-starving” mode, fat storage is also happening simultaneously with fat breakdown, the researchers found. When mice continue to eat the high-fat, low-carb diet beyond one week, Dixit said, they consume more fat than they can burn, and develop diabetes and obesity.
“ They lose the protective gamma delta T-cells in the fat,” he said.
Long-term clinical studies in humans are still necessary to validate the anecdotal claims of keto’s health benefits.
“ Before such a diet can be prescribed, a large clinical trial in controlled conditions is necessary to understand the mechanism behind metabolic and immunological benefits or any potential harm to individuals who are overweight and pre-diabetic,” Dixit said.
There are good reasons to pursue further study: According to the Centers for Disease Control, approximately 84 million American adults — or more than one out of three — have prediabetes (increased blood sugar levels), putting them at higher risk of developing type 2 diabetes, heart disease, and stroke. More than 90% of people with this condition don’t know they have it.
“ Obesity and type 2 diabetes are lifestyle diseases,” Dixit said. “Diet allows people a way to be in control.”
With the latest findings, researchers now better understand the mechanisms at work in bodies sustained on the keto diet, and why the diet may bring health benefits over limited time periods.
“ Our findings highlight the interplay between metabolism and the immune system, and how it coordinates maintenance of healthy tissue function,” said Emily Goldberg, the postdoctoral fellow in comparative medicine who discovered that the keto diet expands gamma-delta T cells in mice.
If the ideal length of the diet for health benefits in humans is a subject for later studies, Dixit said, discovering that keto is better in small doses is good news, he said: “Who wants to be on a diet forever?”
The research was funded in part by grants from the National Institutes of Health.
- Ketogenic diet helps tame flu virus
- Listening to the body: Study examines the effects of fasting on infections
- Study: New fat cells are created quickly, but dieting can’t eliminate them
Health & Medicine
Media Contact
Fred Mamoun: [email protected] , 203-436-2643
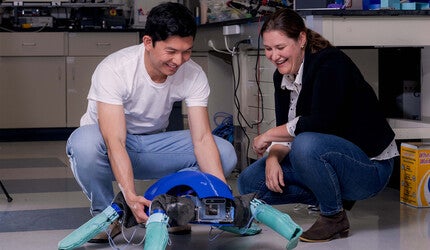
Rebecca Kramer-Bottiglio wins NSF Alan T. Waterman Award

Yale institute observes 50 years studying, celebrating the sacred arts


Sharing more than a border

Ethiopian church forests, a beacon of hope for environmental conservation
- Show More Articles
- See us on facebook
- See us on twitter
- See us on youtube
- See us on linkedin
- See us on instagram
Pilot study shows ketogenic diet improves severe mental illness
A small clinical trial led by Stanford Medicine found that the metabolic effects of a ketogenic diet may help stabilize the brain.
April 1, 2024 - By Nina Bai

A study led by researchers at Stanford Medicine showed that diet can help those with serious mental illness. nishihata
For people living with serious mental illness like schizophrenia or bipolar disorder, standard treatment with antipsychotic medications can be a double-edged sword. While these drugs help regulate brain chemistry, they often cause metabolic side effects such as insulin resistance and obesity, which are distressing enough that many patients stop taking the medications.
Now, a pilot study led by Stanford Medicine researchers has found that a ketogenic diet not only restores metabolic health in these patients as they continue their medications, but it further improves their psychiatric conditions. The results, published March 27 in Psychiatry Research , suggest that a dietary intervention can be a powerful aid in treating mental illness.
“It’s very promising and very encouraging that you can take back control of your illness in some way, aside from the usual standard of care,” said Shebani Sethi , MD, associate professor of psychiatry and behavioral sciences and the first author of the new paper.
Making the connection
Sethi, who is board certified in obesity and psychiatry, remembers when she first noticed the connection. As a medical student working in an obesity clinic, she saw a patient with treatment-resistant schizophrenia whose auditory hallucinations quieted on a ketogenic diet.
That prompted her to dig into the medical literature. There were only a few, decades-old case reports on using the ketogenic diet to treat schizophrenia, but there was a long track record of success in using ketogenic diets to treat epileptic seizures.
“The ketogenic diet has been proven to be effective for treatment-resistant epileptic seizures by reducing the excitability of neurons in the brain,” Sethi said. “We thought it would be worth exploring this treatment in psychiatric conditions.”
A few years later, Sethi coined the term metabolic psychiatry, a new field that approaches mental health from an energy conversion perspective.
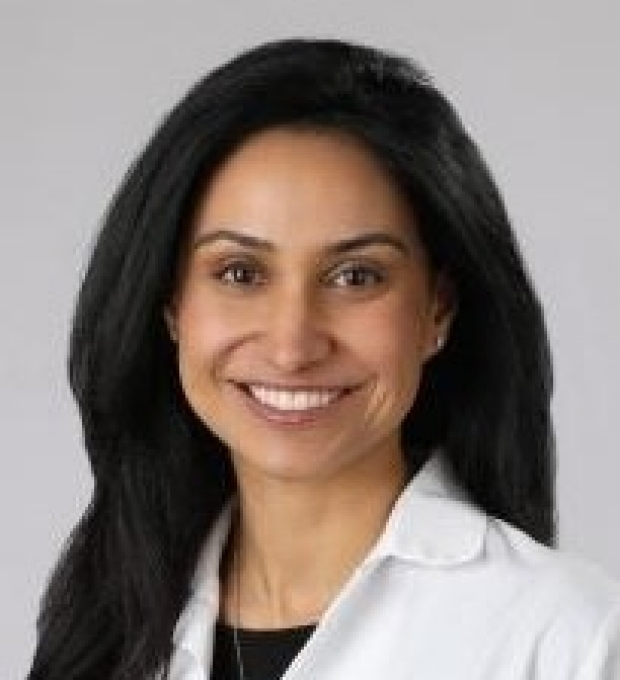
Shebani Sethi
In the four-month pilot trial, Sethi’s team followed 21 adult participants who were diagnosed with schizophrenia or bipolar disorder, taking antipsychotic medications, and had a metabolic abnormality — such as weight gain, insulin resistance, hypertriglyceridemia, dyslipidemia or impaired glucose tolerance. The participants were instructed to follow a ketogenic diet, with approximately 10% of the calories from carbohydrates, 30% from protein and 60% from fat. They were not told to count calories.
“The focus of eating is on whole non-processed foods including protein and non-starchy vegetables, and not restricting fats,” said Sethi, who shared keto-friendly meal ideas with the participants. They were also given keto cookbooks and access to a health coach.
The research team tracked how well the participants followed the diet through weekly measures of blood ketone levels. (Ketones are acids produced when the body breaks down fat — instead of glucose — for energy.) By the end of the trial, 14 patients had been fully adherent, six were semi-adherent and only one was non-adherent.
The participants underwent a variety of psychiatric and metabolic assessments throughout the trial.
Before the trial, 29% of the participants met the criteria for metabolic syndrome, defined as having at least three of five conditions: abdominal obesity, elevated triglycerides, low HDL cholesterol, elevated blood pressure and elevated fasting glucose levels. After four months on a ketogenic diet, none of the participants had metabolic syndrome.
On average, the participants lost 10% of their body weight; reduced their waist circumference by 11% percent; and had lower blood pressure, body mass index, triglycerides, blood sugar levels and insulin resistance.
“We’re seeing huge changes,” Sethi said. “Even if you’re on antipsychotic drugs, we can still reverse the obesity, the metabolic syndrome, the insulin resistance. I think that’s very encouraging for patients.”
The participants reported improvements in their energy, sleep, mood and quality of life.
The psychiatric benefits were also striking. On average, the participants improved 31% on a psychiatrist rating of mental illness known as the clinical global impressions scale, with three-quarters of the group showing clinically meaningful improvement. Overall, the participants also reported better sleep and greater life satisfaction.
“The participants reported improvements in their energy, sleep, mood and quality of life,” Sethi said. “They feel healthier and more hopeful.”
The researchers were impressed that most of the participants stuck with the diet. “We saw more benefit with the adherent group compared with the semi-adherent group, indicating a potential dose-response relationship,” Sethi said.
Alternative fuel for the brain
There is increasing evidence that psychiatric diseases such as schizophrenia and bipolar disorder stem from metabolic deficits in the brain, which affect the excitability of neurons, Sethi said.
The researchers hypothesize that just as a ketogenic diet improves the rest of the body’s metabolism, it also improves the brain’s metabolism.
“Anything that improves metabolic health in general is probably going to improve brain health anyway,” Sethi said. “But the ketogenic diet can provide ketones as an alternative fuel to glucose for a brain with energy dysfunction.”
Likely there are multiple mechanisms at work, she added, and the main purpose of the small pilot trial is to help researchers detect signals that will guide the design of larger, more robust studies.
As a physician, Sethi cares for many patients with both serious mental illness and obesity or metabolic syndrome, but few studies have focused on this undertreated population.
She is the founder and director of the metabolic psychiatry clinic at Stanford Medicine.
“Many of my patients suffer from both illnesses, so my desire was to see if metabolic interventions could help them,” she said. “They are seeking more help. They are looking to just feel better.”
Researchers from the University of Michigan; the University of California, San Francisco; and Duke University contributed to the study.
The study was supported by Baszucki Group Research Fund, the Kuen Lau Fund and the Obesity Treatment Foundation.
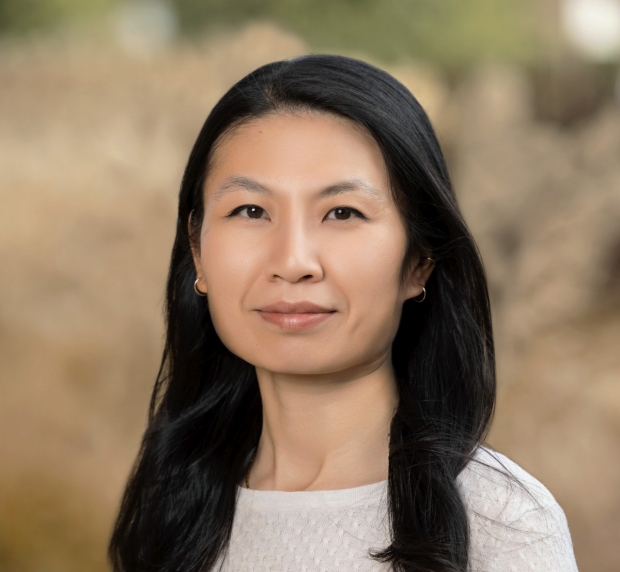
About Stanford Medicine
Stanford Medicine is an integrated academic health system comprising the Stanford School of Medicine and adult and pediatric health care delivery systems. Together, they harness the full potential of biomedicine through collaborative research, education and clinical care for patients. For more information, please visit med.stanford.edu .
Artificial intelligence
Exploring ways AI is applied to health care

CASE REPORT article
Time-restricted ketogenic diet in amyotrophic lateral sclerosis: a case study.
- 1 Department of Neurology, Waikato Hospital, Hamilton, New Zealand
- 2 Older Persons and Rehabilitation Service, Waikato Hospital, Hamilton, New Zealand
- 3 Department of Respiratory Medicine, Waikato Hospital, Hamilton, New Zealand
- 4 Department of Speech Language Therapy, Waikato Hospital, Hamilton, New Zealand
Amyotrophic lateral sclerosis (ALS) is an incurable neurodegenerative disorder. The most devastating variant is bulbar-onset ALS, which portends a median survival of 24 months from the onset of symptoms. Abundant evidence indicates that neuron metabolism and mitochondrial function are impaired in ALS. Metabolic strategies, particularly fasting and ketogenic diet protocols, alter neuron metabolism and mitochondria function in a manner that may mitigate the symptoms of this disorder. We report the case of a 64-year-old man with a 21-month history of progressive, deteriorating bulbar-onset ALS, with an associated pseudobulbar affect, who implemented a time-restricted ketogenic diet (TRKD) for 18 months. During this time, he improved in ALS-related function (7% improvement from baseline), forced expiratory volume (17% improvement), forced vital capacity (13% improvement), depression (normalized), stress levels (normalized), and quality of life (19% improvement), particularly fatigue (23% improvement). His swallowing impairment and neurocognitive status remained stable. Declines were measured in physical function, maximal inspiratory pressure, and maximal expiratory pressure. Weight loss was attenuated and no significant adverse effects occurred. This case study represents the first documented occurrence of a patient with ALS managed with either a fasting or ketogenic diet protocol, co-administered as a TRKD. We measured improved or stabilized ALS-related function, forced expiratory volume, forced vital capacity, swallowing, neurocognitive status, mood, and quality of life. Measurable declines were restricted to physical function, maximal inspiratory pressure, and maximal expiratory pressure. Now over 45 months since symptom onset, our patient remains functionally independent and dedicated to his TRKD.
Introduction
Amyotrophic lateral sclerosis (ALS) is an incurable neurodegenerative disorder that afflicts 4.1–8.4 out of every 100,000 people in the world ( 1 ), with the number of new cases anticipated to rise over the next 20–25 years ( 2 ). Although most patients develop limb-onset ALS, 25–30% of patients develop the more devastating bulbar-onset variant, which is associated with a median survival of 24 months from the onset of symptoms ( 3 ). Bulbar-onset ALS typically presents with dysarthria, dysphagia, or dysphonia. Many patients also develop changes in behavior, cognition, and mood. The pathogenesis of ALS involves the degeneration and death of upper motor neurons in the brain motor cortices, lower motor neurons in the brainstem and spinal cord, and neurons in the frontotemporal regions of the brain ( 4 ). Approximately 90% of patients lack a family history and are classified as sporadic ALS, whereas the remaining 10% show a pattern of inheritance associated with a gene mutation and are classified as familial. Treatment for sporadic and familial ALS is limited and new therapies are needed.
Abundant evidence indicates that neuron metabolism and mitochondrial function are impaired in ALS ( 5 – 10 ). On a morphological level, mitochondria are abnormally shaped, swollen, and vacuolated ( 6 , 11 ). Metabolically, human-derived motor neurons in both sporadic and familial ALS exhibit defective oxidative phosphorylation, ATP loss, and elevated levels of reactive oxygen species ( 9 , 12 ). Mitochondria in ALS motor neurons also display impaired glucose metabolism, tricarboxylic acid (TCA) cycle activity, calcium buffering, axonal transport, and population dynamics ( 5 , 10 ). Moreover, degradations in cell metabolism and mitochondria function have been documented in astrocytes, microglia, Schwann cells, hepatocytes, lymphocytes, and skeletal muscle cells ( 6 ). Collectively, these impairments in cell metabolism and mitochondria function culminate in a chronic bioenergetic challenge that disproportionately impacts metabolically active cells such as neurons, glia, and myocytes.
Metabolic strategies, particularly fasting and ketogenic diet protocols, alter cell metabolism and mitochondria function ( 13 ). Both strategies enhance production of the dominant blood ketone, beta-hydroxybutyrate (BHB), such that its concentration is sustained at 0.5–0.6 mmol/L or higher ( 14 ). BHB metabolism leads to an enhanced free energy of ATP hydrolysis and a greater supply of TCA cycle intermediates ( 15 ). Ketone metabolism also produces fewer reactive oxygen species and increases the production of oxidative stress resistance factors ( 16 , 17 ). Importantly, fasting and ketogenic diet regimens renew the mitochondria pool by upregulating mitogenesis and mitophagy ( 18 ). Despite these beneficial effects, current clinical evidence for metabolic strategies in ALS is limited to a handful of studies involving transgenic mouse models—for example, compared with mice maintained on a normal diet, mice sustained on a ketogenic diet show preserved motor performance ( 19 ), and mice fed caprylic triglyceride (a medium-chain fatty acid that is readily metabolized into ketones) show a delayed progression of weakness, improved performance, and protection from motor neuron loss ( 20 ).
Given the collective evidence, we hypothesized that a metabolic strategy might lead to clinical benefits and improved quality of life in a patient with ALS.
We report the case of a 64-year-old male dairy farm systems stock controller of European background who presented to his general practitioner with 9 months of slurring and slowing of speech, difficulty swallowing solids, coughing when consuming liquids, intermittent sialorrhea, and constant fatigue. His family had also noted intermittent episodes of laughing and sobbing. He was referred to our motor neuron disease clinic, which occurred 9 months later due to resource constraints, by which time his bulbar symptoms had worsened leading to 10 kg of weight loss since symptom onset. Medical history included colorectal cancer (T1N0M0) 12 years previously, which was treated with a hemicolectomy, as well as a myocardial infarction 11 years previously, treated with coronary angioplasty. He had a 2-year history of chronic musculoskeletal pain afflicting both shoulders, hips, and ankles. Regular medications included aspirin and metoprolol. He was a life-long smoker (10 cigarettes a day for 50 years—he had quit 4 years previously, but recently resumed smoking due to frustration over his symptoms). There was no family history of neuromuscular disease. Socially, he lived with his wife, who was also his caregiver, and had recently retired from work due to his symptoms. On examination, our patient had a muscular build, with little body fat, and he exhibited inappropriate laughter and crying throughout the consultation. He was 160 cm in height and 72.2 kg in weight, with a calculated body-mass index of 28.2 kg/m 2 . Neurological examination of the bulbar region revealed definite tongue wasting and fasciculations, a brisk jaw jerk, and spastic dysarthria. Examination of the cervical region revealed mild wasting of the bilateral supraspinatus and infraspinatus muscles and mild weakness in left shoulder abduction, elbow flexion and extension, and finger grip and abduction (all 5-/5). All limb reflexes were normal (2+) and plantar responses were normal. Electromyography of the bulbar, cervical, thoracic, and lumbosacral regions revealed moderate to severe chronic denervation (reduced recruitment and large-amplitude, long-duration motor unit action potentials) in multiple muscles in all four regions. MRI brain showed mild diffuse leukoariosis and MRI spine showed multilevel bilateral foraminal stenoses in the cervical spine, both considered normal for age by a neuroradiologist. An ALS panel analysis for mutations in 35 different genes was negative (ALS Panel, Blueprint Genetics, Espoo, Finland). Blood investigations were normal. At the 3-month follow-up, 21 months after symptom onset, our patient was diagnosed with bulbar-onset ALS with an associated pseudobulbar affect by two independent neurologists (both with neurophysiology fellowships) based on the 2020 Gold Coast criteria ( 21 ).
Given the deteriorating symptoms, riluzole was offered but our patient declined, after which an 18-month time-restricted ketogenic diet (TRKD) was presented as an option ( Figure 1 ). The TRKD involved reducing feeding times to two meals a day. Our patient chose the timing of the two meals every day and up to 1 h was permitted per meal, ensuring that food intake was limited to 2 h per day, with fasting (allowing only water, tea, and coffee) occurring all other hours. The modified ketogenic diet was roughly 60% fat, 30% protein, 5% fiber, and 5% net carbohydrate by weight and comprised primarily of whole foods (green vegetables, meats, eggs, nuts, seeds, creams, and natural oils). Our patient was encouraged to eat to satiation at every meal and not to restrict his calorie intake. After obtaining written informed consent, we provided him with a booklet containing guidelines, recipes, and space to record daily (bedtime) blood glucose and ketone levels using a blood glucose and ketone monitor (CareSens Dual, Pharmaco Diabetes, Auckland, New Zealand). The lead investigator provided support as needed via email. Aside from the TRKD, there were no other lifestyle changes and our patient continued to smoke 10 cigarettes a day.
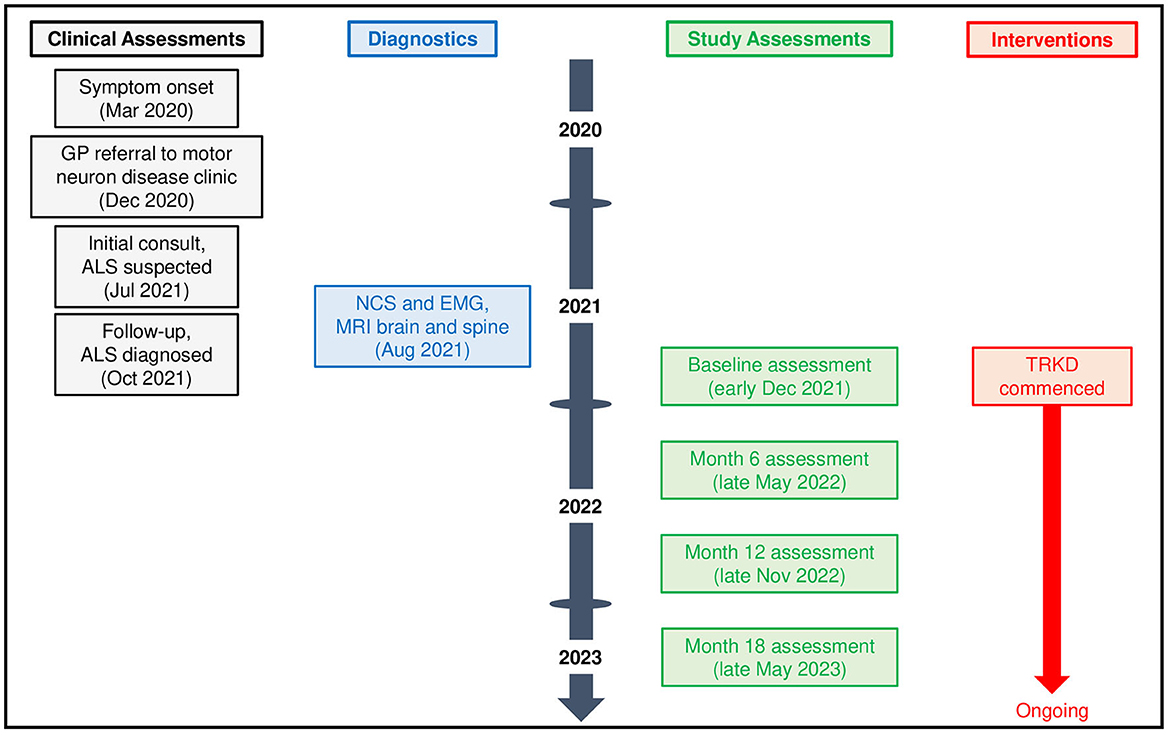
Figure 1 . Patient timeline.
Clinical assessments were conducted during the week preceding the start of the TRKD, with repeat assessments at 6, 12, and 18 months. Clinical assessments evaluated ALS-related function, physical function, pulmonary function, swallowing impairment, neurocognitive status, mood, and quality of life. Assessors were blinded to the intervention. ALS-related function was reported by the patient and caregiver using the Revised ALS Functional Rating Scale (ALSFRS-R) (scores range from 0 to 48, higher numbers indicate improved function) ( 22 ). Physical function was measured by a physiotherapist using the get-up-and-go, 6-min walk, and stair climb tests, with an average from two tests calculated for each measure ( 23 – 25 ). Pulmonary function was measured by a pulmonary clinical nurse specialist using spirometry, which assessed forced expiratory volume, forced vital capacity, maximal inspiratory pressure, and maximal expiratory pressure. Swallowing impairment was assessed by speech and language therapists with videofluoroscopic swallowing study and interpreted using the New Zealand Index for Multidisciplinary Evaluation of Swallowing (NZIMES, available at https://fliphtml5.com/nfqi/zyar/basic ), which delineates the oral phase, oral transit parameters, pharyngeal phase, crico-esophageal parameters, and laryngeal parameters. Neurocognitive status was measured by a neuropsychologist using the Repeatable Battery for the Assessment of Neuropsychological Status (RBANS, with Form A used at baseline and Week 12 and Form B used at Weeks 6 and 18) ( 26 ), Processing Speed from the Wechsler Adult Intelligence Scale—Fourth Edition ( 27 ), the Trail Making Test from the Delis-Kaplan Executive Function System ( 28 ), and the Controlled Oral Word Association Test (COWAT) from the Multilingual Aphasia Examination ( 29 ) (for all tests, higher numbers indicated improved neurocognition), as well as mood using the Depression Anxiety Stress Scale ( 30 ). Quality of life was reported by the patient and caregiver using the Functional Assessment of Chronic Illness Therapy—Fatigue (FACIT-F) (scores range from 0 to 160, higher numbers indicate better quality of life) ( 31 ). Body weight and blood markers were measured at each assessment. We conducted an adverse effects questionnaire with the patient and caregiver at 6, 12, and 18 months.
During the 18-month TRKD, our patient's mean blood glucose and ketone levels were 6.52 +/- 0.91 and 0.77 +/- 0.43 mmol/L, respectively ( Figure 2 ), with improvement or stability documented in most outcome measures ( Tables 1 , 2 ). Regarding function, the ALSFRS-R improved (42–45, representing a 7% improvement from baseline). The get-up-and-go, 6-min walk, and stair climb tests showed decline. Regarding pulmonary function, the forced expiratory volume improved (2.31–2.70 L, representing a 17% improvement), as did forced vital capacity (3.83–4.33 L, representing a 13% improvement). The maximal inspiratory and expiratory pressures declined. Baseline swallowing impairment remained stable aside from the oral phase (none to mild impairment) and laryngeal parameters (moderate to mild impairment). Despite his stabilized swallowing function, our patient opted for a radiologically inserted gastrostomy (RIG) insertion 9 months into the TRKD to mitigate symptoms of aspiration, which existed before the TRKD was implemented and were only triggered by water (to date, the RIG remains solely used for water intake). The neurocognitive tests generally remained stable. Baseline depression and stress levels resolved (moderate to normal). Regarding quality of life, the FACIT-F improved (114–136, representing a 19% improvement), particularly fatigue as measured by the FACIT-F subscale (35–43, representing a 23% improvement). Our patient's weight was 72.2 kg at baseline and 69.0 kg at month 18. Blood investigations for hemoglobin, creatinine, liver function tests, and glycosylated hemoglobin remained normal over 18 months. Blood triglycerides increased from 1.3 to 1.6 mmol/L, high-density lipoprotein remained at 1.1 mmol/L, low-density lipoprotein increased from 3.1 to 5.4 mmol/L, and total cholesterol increased from 4.8 to 7.2 mmol/L. Our patient experienced no significant adverse effects during the TRKD and consistently mentioned enhanced energy, improved sleep, and reductions in his chronic musculoskeletal pain.
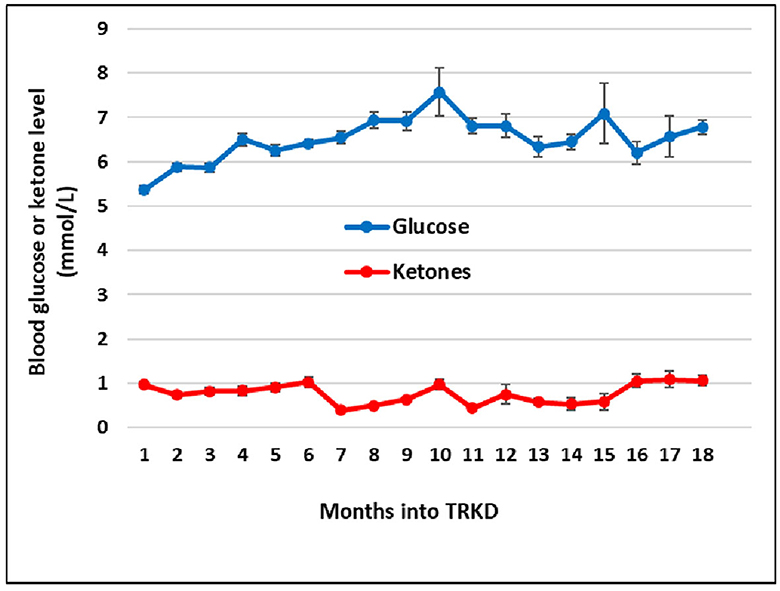
Figure 2 . Mean monthly blood glucose and ketone (beta-hydroxybutyrate) levels during the time-restricted ketogenic diet (TRKD). Error bars indicate standard error.
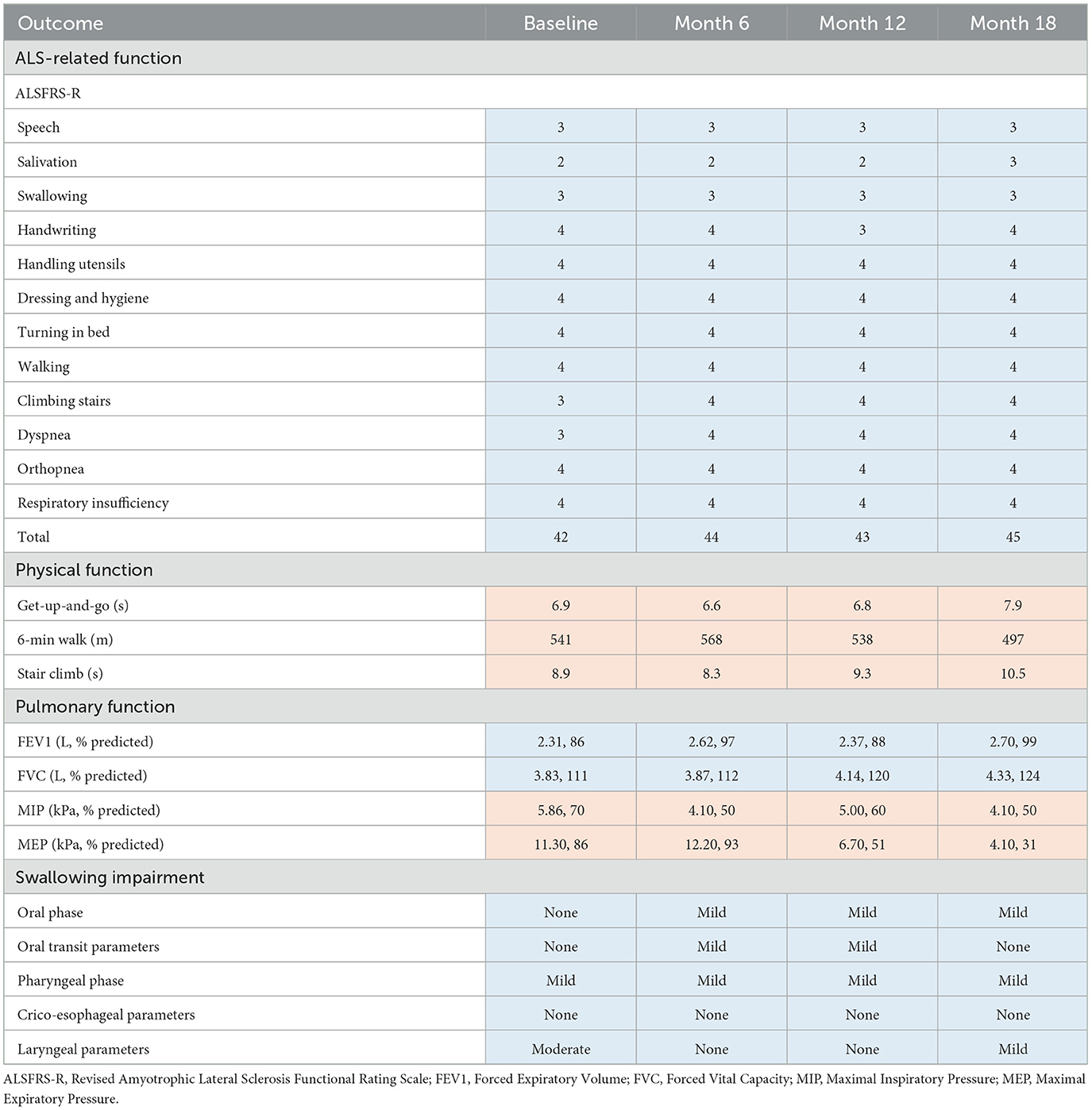
Table 1 . Outcome measures for ALS-related, physical, pulmonary, and swallowing function at baseline, 6 months, 12 months, and 18 months after commencing the time-restricted ketogenic diet (for all tests except the get-up-and-go and stair climb, higher numbers indicate improved outcomes; improved or stabilized outcomes are highlighted in blue and declines are highlighted in red).
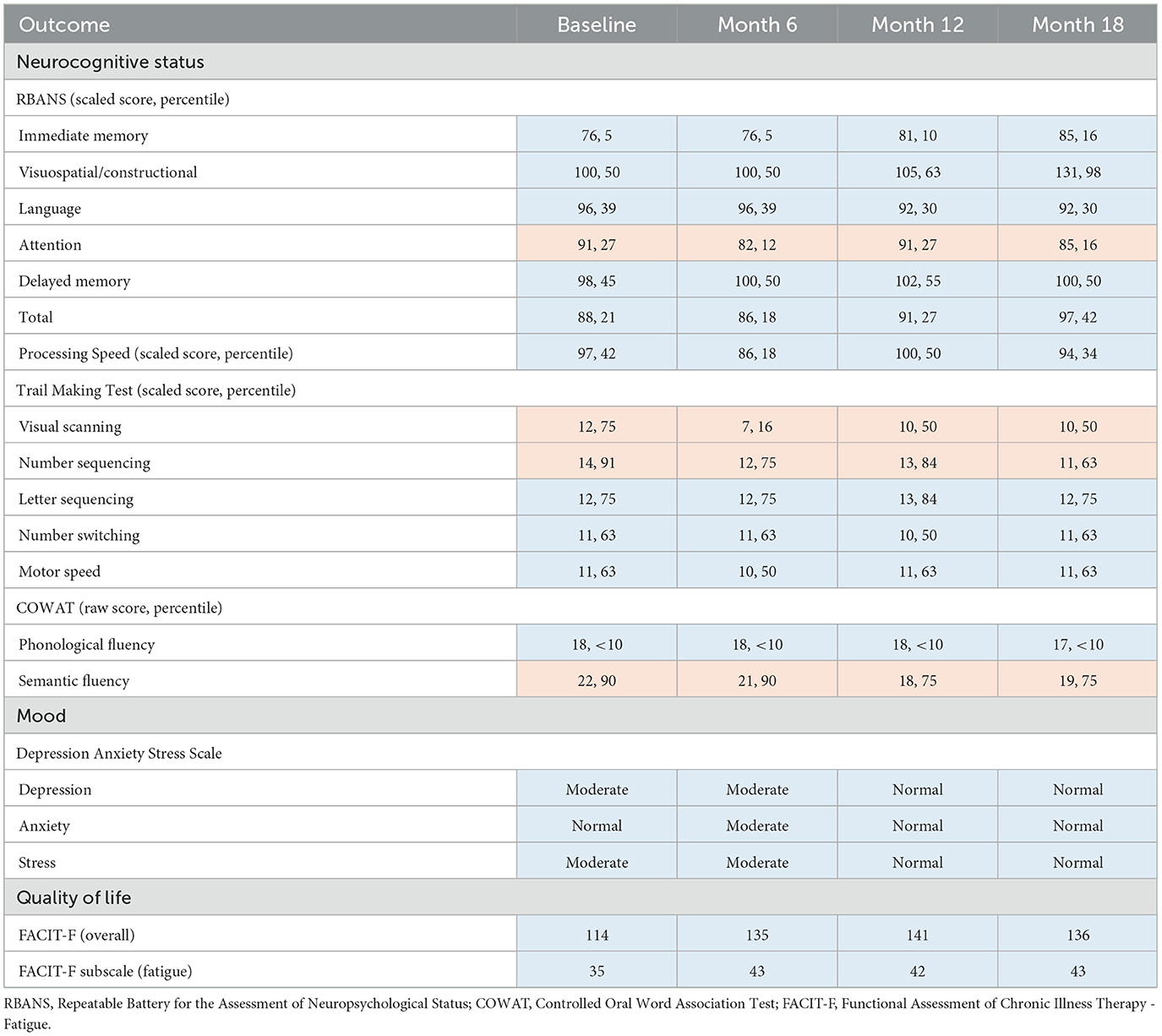
Table 2 . Outcome measures for neurocognitive status, mood, and quality of life at baseline, 6 months, 12 months, and 18 months after commencing the time-restricted ketogenic diet (for all tests, higher numbers indicate improved outcomes; improved or stabilized outcomes are highlighted in blue and declines are highlighted in red).
This case study represents the first documented occurrence of a patient with ALS managed with either a fasting or ketogenic diet protocol, co-administered as a TRKD. We measured improved ALS-related function (7% improvement from baseline), forced expiratory volume (17% improvement), forced vital capacity (13% improvement), depression (normalized), stress levels (normalized), and quality of life (19% improvement), particularly fatigue (23% improvement). His swallowing impairment and neurocognitive status remained stable. Measurable declines were restricted to physical function, maximal inspiratory pressure, and maximal expiratory pressure. Weight loss was attenuated and no significant adverse effects occurred.
Although fasting and ketogenic diet protocols may individually confer metabolic benefits in ALS, they can be readily combined. Time-restricted feeding eases the burden of organizing multiple meals, which compensates for the extra time required to become familiar with a ketogenic diet, whereas the diet may improve long-term hunger ( 32 ), which increases the tolerability of the fasts. The modified ketogenic diet used here was simple, flexible, palatable, and affordable, which alleviated the restrictions that have been associated with ketogenic diets in the past ( 33 ). Regardless of whether metabolic strategies are isolated or combined, it is important to monitor blood glucose and BHB levels so that difficulties can be detected and resolved. Despite good adherence to the 18-month TRKD, our patient's mean blood glucose levels averaged 6.52 mmol/L and his BHB 0.77 mmol/L, which is in the lower range of physiological ketosis (albeit, still within range). This may relate to his body composition and the ALS process itself. Our patient's lower fat mass probably provided less fuel reserve for fasting-induced ketogenesis. Moreover, many individuals with ALS exhibit skeletal muscle hypermetabolism ( 5 ), which may constitute an adaptive response to decreased energy metabolism efficiency ( 34 ). Hypermetabolism could have increased our patient's ketone utilization, leading to lower blood levels.
During this case study, our patient improved or stabilized in most measures of function, which is consistent with TRKD-induced enhancements in neuron, glial cell, and myocyte metabolism as well as mitochondria function. The ALS community relies on the ALSFRS-R to monitor activities of daily living and disease progression ( 4 , 22 ), which typically declines by 1 point per month ( 35 ). Given our patient's baseline score of 42, his score should have declined to 24 during the TRKD. Instead, it improved to 45. Although ALSFRS-R score changes do not necessarily reflect improvement ( 4 ), and the specific tests of physical function declined at the final assessment, the fact that our patient's score did not decline over 18 months is potentially important. With respect to pulmonary function, forced vital capacity is arguably the most significant spirometric correlate of disease progression in ALS ( 36 ), which shows an average decline of 2–3% predicted per month ( 35 ). Given our patient's baseline of 3.83 L (111% predicted), his score should have declined to at least 2.87 L (75% predicted) during the TRKD. Instead, it improved to 4.33 L (124% predicted). Importantly, the maximal inspiratory and expiratory pressures declined during the TRKD, which is discordant with the improved forced expiratory volume and vital capacity, but these measurements should be viewed cautiously as both tests are notoriously difficult to perform and poorly predictive of respiratory capacity ( 37 ). Lastly, swallowing impairment occurs in 85–92% of patients diagnosed with bulbar-onset ALS and is associated with malnutrition, aspiration pneumonia, faster functional decline, and increased mortality ( 38 ). Importantly, our patient's swallowing function remained stable during the 18-month TRKD, with only minor variations noted in the oral phase (slightly declined) and laryngeal parameters (slightly improved), both of which can be attributed to typical variations seen from swallow to swallow.
Our patient improved or remained stable in most measures of neurocognitive status, mood, and quality of life. Although ALS is often considered a neuromuscular disorder, 50% of patients exhibit impaired executive function, language fluency, or cognition due to pathology in the frontotemporal region ( 39 , 40 ). The RBANS measures a variety of cognitive domains related to memory, visuospatial and constructional ability, expressive language, and attention ( 26 ). Our patient's low baseline RBANS scores (21st percentile) suggested a degree of baseline executive-type impairment, which remained stable (or improved) after the 18-month TRKD (42nd percentile). The COWAT measures verbal fluency ( 29 ). Our patient's low baseline phonological fluency scores (< 10th percentile) indicated substantial language impairment at baseline, which remained stable. The ongoing stability in most of the neurocognitive tests is encouraging and may reflect TRKD-associated enhancements in neocortical neuron metabolism and mitochondria function. From the perspective of our patient and his wife, the greatest benefits associated with the TRKD related to his mood and energy levels. His moderate baseline depression and stress levels resolved. He also improved his quality of life and fatigue scores, which allowed him to maintain an active outdoors lifestyle on his farm.
During the 21 months prior to the TRKD, our patient lost 10 kg of body weight, which was concerning given that weight loss is negatively correlated with survival in ALS ( 7 , 8 ). By contrast, he lost only 3.2 kg of weight during the 18-month TRKD. Although the resumption of his smoking habit may have accounted for a portion of the 10 kg of weight loss, the relative weight preservation during the TRKD hints at a weight-sparing effect. It might seem paradoxical that a strategy with the potential to induce a caloric deficit could somehow lead to a weight-sparing effect in ALS. However, the explanation may lie in a TRKD-induced increased efficiency of ATP synthesis. ALS involves the accumulation of damaged, uncoupled mitochondria ( 41 ), followed by a progressive decrease in energy metabolism efficiency, energy dissipation through thermogenesis, and ATP depletion despite failing attempts by hypermetabolism to compensate for the shortfall. Fasting may counter this process by inducing mitochondria renewal and coupling, which can increase the rate of ATP synthesis—for example, in a study involving uncoupling protein-3 knockout mice, fasted mice showed a four-fold higher rate of ATP synthesis compared to fed mice despite no measurable difference in TCA cycle activity or whole-body energy expenditure ( 42 ). The TRKD may have increased ATP synthesis efficiency in our patient, culminating in less generated energy being “lost” and a subsequent sparing effect on muscle and fat reserves. Consistent with this hypothesis, well-designed trials have shown that simply increasing calorie intake, which would not increase metabolic efficiency, does not significantly attenuate weight loss in people with ALS ( 43 , 44 ).
Given that this is a case study, we cannot draw firm conclusions regarding the mechanism of the documented improvements or potential impact of the TRKD on survival. Clinical features associated with shorter survival times include bulbar-onset ALS, older age, rapid functional decline (as measured by the ALSFRS-R), low forced vital capacity, frontotemporal dementia, and pronounced weight loss ( 3 ). Given that most of these negative prognostic features either improved or stabilized in our patient, it is reasonable to anticipate an ongoing TRKD-induced survival benefit. Alternative explanations for some of the improvements include a practice effect and a placebo effect. Although the former is possible, this seems unlikely given the lengthy 6-month time intervals between assessments. Since it is not possible to blind patients to metabolic strategies, a placebo effect may have partially contributed to the improvements.
In conclusion, this case study represents the first documented occurrence of a patient with ALS managed with either a fasting or ketogenic diet protocol, co-administered as a TRKD. We measured improved or stabilized ALS-related function, forced expiratory volume, forced vital capacity, swallowing impairment, neurocognitive status, depression, stress levels, and quality of life. Measurable declines were restricted to physical function, maximal inspiratory pressure, and maximal expiratory pressure. Our patient remains functionally independent and dedicated to his TRKD. Despite its limitations, this case study is encouraging and serves as a proof-of-concept for further studies involving metabolic strategies in ALS.
Data availability statement
The original contributions presented in the study are included in the article/supplementary material, further inquiries can be directed to the corresponding author.
Ethics statement
Ethical approval was not required for this study involving a human participant because local and institutional review boards do not require ethics approval for case reports/studies, so long as written informed consent is obtained. The study was conducted in accordance with the local legislation and institutional requirements. The participant provided written informed consent to participate in this study. Written informed consent was obtained from the individual for the publication of any potentially identifiable images or data included in this article.
Author contributions
MP: Conceptualization, Formal analysis, Methodology, Supervision, Writing—original draft, Writing—review & editing. SJ: Data curation, Writing—review & editing. PS: Data curation, Writing—review & editing. DC: Data curation, Writing—review & editing. DM: Data curation, Writing—review & editing. RD: Data curation, Formal analysis, Methodology, Writing—review & editing.
The author(s) declare that no financial support was received for the research, authorship, and/or publication of this article.
Conflict of interest
The authors declare that the research was conducted in the absence of any commercial or financial relationships that could be construed as a potential conflict of interest.
Publisher's note
All claims expressed in this article are solely those of the authors and do not necessarily represent those of their affiliated organizations, or those of the publisher, the editors and the reviewers. Any product that may be evaluated in this article, or claim that may be made by its manufacturer, is not guaranteed or endorsed by the publisher.
1. Longinetti E, Fang F. Epidemiology of amyotrophic lateral sclerosis: an update of recent literature. Curr Opin Neurol. (2019) 32:771–6. doi: 10.1097/WCO.0000000000000730
PubMed Abstract | Crossref Full Text | Google Scholar
2. Arthur KC, Calvo A, Price TR, Geiger JT, Chió A, Traynor BJ. Projected increase in amyotrophic lateral sclerosis from 2015 to 2040. Nat Commun. (2016) 7:12408. doi: 10.1038/ncomms12408
3. Masrori P, Van Damme P. Amyotrophic lateral sclerosis: a clinical review. Eur J Neurol. (2020) 27:1918–29. doi: 10.1111/ene.14393
Crossref Full Text | Google Scholar
4. Goutman SA, Hardiman O, Al-Chalabi A, Chió A, Savelieff MG, Kiernan MC, et al. Recent advances in the diagnosis and prognosis of amyotrophic lateral sclerosis. Lancet Neurol. (2022) 21:480–93. doi: 10.1016/S1474-4422(21)00465-8
5. Blasco H, Lanznaster D, Veyrat-Durebex C, Hergesheimer R, Vourch P, Maillot F, et al. Understanding and managing metabolic dysfunction in amyotrophic lateral sclerosis. Expert Rev Neurother. (2020) 20:907–19. doi: 10.1080/14737175.2020.1788389
6. Cozzolino M, Carrì MT. Mitochondrial dysfunction in ALS. Prog Neurobiol. (2012) 97:54–66. doi: 10.1016/j.pneurobio.2011.06.003
7. Dupuis L, Pradat P-F, Ludolph AC, Loeffler J-P. Energy metabolism in amyotrophic lateral sclerosis. Lancet Neurol. (2011) 10:75–82. doi: 10.1016/S1474-4422(10)70224-6
8. Guillot SJ, Bolborea M, Dupuis L. Dysregulation of energy homeostasis in amyotrophic lateral sclerosis. Curr Opin Neurol. (2021) 34:773–80. doi: 10.1097/WCO.0000000000000982
9. Smith EF, Shaw PJ, De Vos KJ. The role of mitochondria in amyotrophic lateral sclerosis. Neurosci Lett. (2019) 710:132933. doi: 10.1016/j.neulet.2017.06.052
10. Zhao J, Wang X, Huo Z, Chen Y, Liu J, Zhao Z, et al. The impact of mitochondrial dysfunction in amyotrophic lateral sclerosis. Cells. (2022) 11:2049. doi: 10.3390/cells11132049
11. Kong J, Xu Z. Massive mitochondrial degeneration in motor neurons triggers the onset of amyotrophic lateral sclerosis in mice expressing a mutant SOD1. J Neurosci. (1998) 18:3241–50. doi: 10.1523/JNEUROSCI.18-09-03241.1998
12. Singh T, Jiao Y, Ferrando LM, Yablonska S, Li F, Horoszko EC, et al. Neuronal mitochondrial dysfunction in sporadic amyotrophic lateral sclerosis is developmentally regulated. Sci Rep. (2021) 11:18916. doi: 10.1038/s41598-021-97928-7
13. Phillips MCL. Metabolic strategies in healthcare: a new era. Aging Dis. (2022) 13:655–72. doi: 10.14336/AD.2021.1018
14. VanItallie TB, Nufert TH. Ketones: metabolism's ugly duckling. Nutr Rev. (2003) 61:327–41. doi: 10.1301/nr.2003.oct.327-341
15. Veech RL, Chance B, Kashiwaya Y, Lardy HA, Cahill GF. Ketone bodies, potential therapeutic uses. IUBMB Life. (2001) 51:241–7. doi: 10.1080/152165401753311780
16. Masino SA, Ruskin DN. Ketogenic diets and pain. J Child Neurol. (2013) 28:993–1001. doi: 10.1177/0883073813487595
17. Shimazu T, Hirschey MD, Newman J, He W, Shirakawa K, Le Moan N, et al. Suppression of oxidative stress by β-hydroxybutyrate, an endogenous histone deacetylase inhibitor. Science. (2013) 339:211–4. doi: 10.1126/science.1227166
18. de Cabo R, Mattson MP. Effects of intermittent fasting on health, aging, and disease. N Engl J Med. (2019) 381:2541–51. doi: 10.1056/NEJMra1905136
19. Zhao Z, Lange DJ, Voustianiouk A, MacGrogan D, Ho L, Suh J, et al. A ketogenic diet as a potential novel therapeutic intervention in amyotrophic lateral sclerosis. BMC Neurosci. (2006) 7:29. doi: 10.1186/1471-2202-7-29
20. Zhao W, Varghese M, Vempati P, Dzhun A, Cheng A, Wang J, et al. Caprylic triglyceride as a novel therapeutic approach to effectively improve the performance and attenuate the symptoms due to the motor neuron loss in ALS disease. PLoS ONE. (2012) 7:e49191. doi: 10.1371/journal.pone.0049191
21. Shefner JM, Al-Chalabi A, Baker MR, Cui L-Y, de Carvalho M, Eisen A, et al. A proposal for new diagnostic criteria for ALS. Clin Neurophysiol. (2020) 131:1975–8. doi: 10.1016/j.clinph.2020.04.005
22. Cedarbaum JM, Stambler N, Malta E, Fuller C, Hilt D, Thurmond B, et al. The ALSFRS-R: a revised ALS functional rating scale that incorporates assessments of respiratory function. J Neurol Sci. (1999) 169:13–21. doi: 10.1016/S0022-510X(99)00210-5
23. Mathias S, Nayak US, Isaacs B. Balance in elderly patients: the ‘get-up and go' test. Arch Phys Med Rehabil. (1986) 67:387–9.
Google Scholar
24. Enright PL. The six-minute walk test. Respir Care. (2003) 48:783−5.
25. Bean JF, Kiely DK, LaRose S, Alian J, Frontera WR. Is stair climb power a clinically relevant measure of leg power impairments in at-risk older adults? Arch Phys Med Rehabil. (2007) 88:604–9. doi: 10.1016/j.apmr.2007.02.004
26. Randolph C. Repeatable Battery for the Assessment of Neuropsychological Status (RBANS). San Antonio, Harcourt, TX: The Psychological Corporation (1998).
27. Wechsler D. Wechsler Adult Intelligence Scale–Fourth Edition (WAIS–IV). San Antonio, TX: Pearson (2008).
28. Delis DC, Kaplan EF, Kramer JH. Delis-Kaplan Executive Function System . San Antonio, TX: Psychological Corporation. (2001).
29. Benton AL, Hamsher K, Rey GL, Sivan AB. Multilingual Aphasia Examination (3rd ed). Iowa City, IA: AJA Associates (1994).
30. Lovibond SH, Lovibond PF. Manual for the Depression Anxiety Stress Scales (2nd ed.). Sydney: Psychology Foundation of Australia (1995).
31. Yellen SB, Cella DF, Webster K, Blendowski C, Kaplan E. Measuring fatigue and other anemia-related symptoms with the functional assessment of cancer therapy (FACT) measurement system. J Pain Symptom Manage. (1997) 13:63–74. doi: 10.1016/s0885-3924(96)00274-6
32. Ludwig DS, Willett WC, Volek JS, Neuhouser ML. Dietary fat: from foe to friend? Science. (2018) 362:764–70. doi: 10.1126/science.aau2096
33. Cervenka MC, Henry BJ, Felton EA, Patton K, Kossoff EH. Establishing an adult epilepsy diet center: experience, efficacy and challenges. Epilepsy Behav. (2016) 58:61–8. doi: 10.1016/j.yebeh.2016.02.038
34. Konrad C, Kawamata H, Bredvik KG, Arreguin AJ, Cajamarca SA, Hupf JC, et al. Fibroblast bioenergetics to classify amyotrophic lateral sclerosis patients. Mol Neurodegener. (2017) 12:76. doi: 10.1186/s13024-017-0217-5
35. Atassi N, Berry J, Shui A, Zach N, Sherman A, Sinani E, et al. The PRO-ACT database: design, initial analyses, and predictive features. Neurology. (2014) 83:1719–25. doi: 10.1212/WNL.0000000000000951
36. Pirola A, De Mattia E, Lizio A, Sannicolò G, Carraro E, Rao F, et al. The prognostic value of spirometric tests in amyotrophic lateral sclerosis patients. Clin Neurol Neurosurg. (2019) 184:105456. doi: 10.1016/j.clineuro.2019.105456
37. Caruso P, Albuquerque AL, Santana PV, Cardenas LZ, Ferreira JG, Prina E, et al. Diagnostic methods to assess inspiratory and expiratory muscle strength. J Bras Pneumol. (2015) 41:110–23. doi: 10.1590/S1806-37132015000004474
38. Perry BJ, Nelson J, Wong JB, Kent DM. The cumulative incidence of dysphagia and dysphagia-free survival in persons diagnosed with amyotrophic lateral sclerosis. Muscle Nerve. (2021) 64:83–6. doi: 10.1002/mus.27244
39. Lomen-Hoerth C, Murphy J, Langmore S, Kramer JH, Olney RK, Miller B. Are amyotrophic lateral sclerosis patients cognitively normal? Neurology. (2003) 60:1094–7. doi: 10.1212/01.wnl.0000055861.95202.8d
40. Ringholz GM, Appel SH, Bradshaw M, Cooke NA, Mosnik DM, Schulz PE. Prevalence and patterns of cognitive impairment in sporadic ALS. Neurology. (2005) 65:586–90. doi: 10.1212/01.wnl.0000172911.39167.b6
41. Dupuis L, di Scala F, Rene F, de Tapia M, Oudart H, Pradat P-F, et al. Up-regulation of mitochondrial uncoupling protein 3 reveals an early muscular metabolic defect in amyotrophic lateral sclerosis. FASEB J. (2003) 17:2091–3. doi: 10.1096/fj.02-1182fje
42. Cline GW, Vidal-Puig AJ, Dufour S, Cadman KS, Lowell BB, Shulman GI. In vivo effects of uncoupling protein-3 gene disruption on mitochondrial energy metabolism. J Biol Chem . (2001) 276:20240–4. doi: 10.1074/jbc.M102540200
43. Ludolph AC, Dorst J, Dreyhaupt J, Weishaupt JH, Kassubek J, Weiland U, et al. Effect of high-caloric nutrition on survival in amyotrophic lateral sclerosis. Ann Neurol. (2020) 87:206–16. doi: 10.1002/ana.25661
44. Wills A-M, Hubbard J, Macklin EA, Glass J, Tandan R, Simpson EP, et al. Hypercaloric enteral nutrition in patients with amyotrophic lateral sclerosis: a randomised, double-blind, placebo-controlled phase 2 trial. Lancet. (2014) 383:2065–72. doi: 10.1016/S0140-6736(14)60222-1
Keywords: motor neuron disease, amyotrophic lateral sclerosis, neurodegeneration, energy metabolism, mitochondria dysfunction, metabolic strategy, fasting, ketogenic diet
Citation: Phillips MCL, Johnston SE, Simpson P, Chang DK, Mather D and Dick RJ (2024) Time-restricted ketogenic diet in amyotrophic lateral sclerosis: a case study. Front. Neurol. 14:1329541. doi: 10.3389/fneur.2023.1329541
Received: 29 October 2023; Accepted: 27 December 2023; Published: 18 January 2024.
Reviewed by:
Copyright © 2024 Phillips, Johnston, Simpson, Chang, Mather and Dick. This is an open-access article distributed under the terms of the Creative Commons Attribution License (CC BY) . The use, distribution or reproduction in other forums is permitted, provided the original author(s) and the copyright owner(s) are credited and that the original publication in this journal is cited, in accordance with accepted academic practice. No use, distribution or reproduction is permitted which does not comply with these terms.
*Correspondence: Matthew C. L. Phillips, Matthew.Phillips@waikatodhb.health.nz
Disclaimer: All claims expressed in this article are solely those of the authors and do not necessarily represent those of their affiliated organizations, or those of the publisher, the editors and the reviewers. Any product that may be evaluated in this article or claim that may be made by its manufacturer is not guaranteed or endorsed by the publisher.

An official website of the United States government
The .gov means it’s official. Federal government websites often end in .gov or .mil. Before sharing sensitive information, make sure you’re on a federal government site.
The site is secure. The https:// ensures that you are connecting to the official website and that any information you provide is encrypted and transmitted securely.
- Publications
- Account settings
Preview improvements coming to the PMC website in October 2024. Learn More or Try it out now .
- Advanced Search
- Journal List
- J Int Soc Sports Nutr
Ketogenic diet benefits body composition and well-being but not performance in a pilot case study of New Zealand endurance athletes
Auckland University of Technology (AUT). Sports Performance Research Institute New Zealand (SPRINZ). AUT Millennium, 17 Antares Place, Mairangi Bay, Auckland, New Zealand
Matthew Wood
Mikki williden, simon chatterton, associated data.
The data that support the findings of this study are available on request from the corresponding author.
Low-carbohydrate, high-fat and ketogenic diets are increasingly adopted by athletes for body composition and sports performance enhancements. However, as yet, there is no consensus on their efficacy in improving performance. There is also no comprehensive literature on athletes’ experiences while undertaking this diet. The purpose of this pilot work was two-fold: i. to examine the effects of a non-calorie controlled ketogenic diet on body composition and performance outcomes of endurance athletes, and ii. to evaluate the athletes’ experiences of the ketogenic diet during the 10-week intervention.
Using a case study design, five New Zealand endurance athletes (4 females, 1 male) underwent a 10-week ketogenic dietary intervention. Body composition (sum of 8 skinfolds), performance indicators (time to exhaustion, VO 2 max, peak power and ventilatory threshold), and gas exchange thresholds were measured at baseline and at 10 weeks. Mean change scores were calculated, and analysed using t-tests; Cohen’s effect sizes and 90% confidence limits were applied to quantify change. Individual interviews conducted at 5 weeks and a focus group at 10 weeks assessed athletes’ ketogenic diet experiences. Data was transcribed and analysed using thematic analysis.
All athletes increased their ability to utilise fat as a fuel source, including at higher exercise intensities. Mean body weight was reduced by 4 kg ± SD 3.1 ( p = 0.046; effect size (ES):0.62), and sum of 8 skinfolds by 25.9 mm ± SD 6.9; ES: 1.27; p = 0.001). Mean time to exhaustion dropped by ~2 min (±SD 0.7; p = 0.004; ES: 0.53). Other performance outcomes showed mean reductions, with some increases or unchanged results in two individuals (VO2 Max: −1.69 ml.kg.min ± SD 3.4 ( p = 0.63); peak power: -18 W ± SD 16.4 ( p = 0.07), and VT2: -6 W ± SD 44.5 ( p = 0.77). Athletes reported experiencing reduced energy levels initially, followed by a return of high levels thereafter, especially during exercise, but an inability to easily undertake high intense bouts. Each athlete reported experiencing enhanced well-being, included improved recovery, improvements in skin conditions and reduced inflammation.
Conclusions
Despite performance decrements and some negative experiences, athletes were keen to pursue a modified low-carbohydrate, high-fat eating style moving forward due to the unexpected health benefits they experienced.
Trial registration
ACTRN: ACTRN12617000613303 . Registered 28 April 2017, retrospectively registered.
Low-carbohydrate, high-fat (LCHF) diets, including their extreme version i.e., ketogenic diets have recently become popular dietary regimes for athletes for several reasons. However, there is no consensus regarding the efficacy of ketogenic diets on sports performance. The overarching mainstream nutrition philosophy for endurance athletes, is one that emphasises a carbohydrate-dominant, low fat paradigm. Under these dietary conditions, athletes utilise carbohydrate as their predominant fuel source to fuel high volumes of aerobic exercise [ 1 ]. The appeal of LCHF eating for endurance athletes is likely due to the shift in fuel utilisation, from a carbohydrate-centric model to one that utilises fat predominantly, of which stores are unlimited compared to carbohydrate (i.e., muscle glycogen). This metabolic shift, seen after a period of dietary alteration is often referred to as being ‘fat-adapted’, which has been well-documented in studies since the 1980s [ 2 , 3 ].
Despite the physiological advantage of utilising fat as a fuel source during sub-maximal exercise, to date there is no conclusive evidence to suggest that this results in subsequent performance enhancement [ 4 ]. Some individual responses to a ketogenic diet have shown dramatic benefits in both fat metabolism and performance, and are worth further investigation [ 5 , 6 ]. However, studies have also shown a reduction in maximal aerobic performance [ 7 ] with some evidence indicating a negative effect on exercise intensity >70% of VO 2 max [ 8 , 9 ]. The majority of these studies have been criticised for not being long enough to allow for the full adaptive mechanisms to occur, which appear to require at least 21 days.
Despite the lack of peer-reviewed evidence for performance enhancement, athletes continue to be intrigued with the LCHF dietary paradigm. It is likely that this is the case for two reasons that go beyond the desire to obtain the extra performance edge: 1. a reduction in body fat that is frequently cited when athletes undertake LCHF or ketogenic diets [ 10 ], and 2. the anecdotal benefits that are cited by athletes eating this way. There is very little athlete-specific literature, particularly of a qualitative nature, addressing non-performance outcomes of LCHF or ketogenic eating in an athlete cohort. In a translational case study design, this pilot study set out to investigate the effect of a 10-week ketogenic diet on body composition and performance outcomes in five New Zealand endurance athletes, as well as to evaluate, qualitatively, the athletes’ experiences of undertaking the ketogenic diet during their training season.
Study design
This was a pilot, 10-week intervention undertaken with five case studies. A control group was not included. The study took place at AUT, Human Potential Centre; ethics was approved by the AUT Ethics Committee (application 15/415).
Participants
Five recreational endurance athletes (four females, one male) consented to participate in this study. All participants were known to the primary investigator and were all highly motivated athletes regularly involved in high-level competitive endurance sport for at least five years. All participants were non-smokers, healthy and injury-free as per a health screening questionnaire and not consuming a low carbohydrate diet (defined as carbohydrates less than 45% of total energy intake). See Table Table1 1 for participant demographics. The athletes were curious about the efficacy of the ketogenic diet on their body composition and sports performance. None of them had any previous experience with, or pre-conceptions about low carbohydrate or ketogenic diets prior to this trial.
Participant demographics
Study protocol
Prior to the start of the intervention, participants underwent a series of tests and a full consultation for dietary instruction and planning as follows:
- Performance test. Participants reported to the lab in the morning in a fasted state and underwent a performance test on three occasions: i. one week prior to the intervention (familiarisation), ii. immediately prior to the intervention (baseline), and iii. Immediately post the intervention (post). To determine V . O 2peak and gas exchange thresholds (GET) an incremental cycle test was performed using an electromagnetically controlled cycle ergometer (Ergoselect 100, Ergoline, Bitz, Germany) in a temperature-controlled laboratory (21 °C, 65% rH). The test commenced at 30 W and increased by 30 W every 3 min until volitional exhaustion. Participants were instructed to maintain a cadence of 80 revolutions.min −1 . Oxygen uptake ( V . O 2 ) was measured continuously using a breath-by-breath metabolic system (Metamax 3b, Cortex, Leipzig, Germany), and heart rate was continuously measured using a short-range telemetry device (Suunto M2, Suunto, Vantaa, Finland). The V . O 2peak was defined as the highest 30s V . O 2 value and the GET was identified independently by two experienced investigators using the V-slope method [ 11 ].
- Dietary instruction. An initial consultation was conducted with each participant by the primary researcher/Registered Dietitian directly after the performance test. Participants were provided with a daily macronutrient prescription of <50 g total carbohydrate, 1.5 g.kg protein and ad libitum fat. A detailed explanation of the practical application of the ketogenic dietary principles along with appropriate tracking and monitoring procedures was provided. Participants were provided with a sample diet and were advised to add variations as long as they adhered to the carbohydrate and protein macronutrient thresholds. They were instructed to track their diet using a dietary analysis programme Easy Diet Diary® (Xyris Software (Australia) Pty Ltd). Diets were monitored weekly by the primary researcher, and participants were contacted and offered additional support if they deviated. The primary researcher maintained regular contact with all participants throughout the 10-week study duration.
- Exercise instruction. The participants were all seasoned athletes, and participated on a regular basis in endurance events, i.e., mountain biking, road biking, running and multisport events (which included running, cycling and kayaking), both recreationally and competitively. Hence, their training protocols did not vary much from month-to-month. They were instructed to continue with their existing training volumes for the duration of the intervention.
- Ketone blood testing. Each participant was shown how to measure blood ketone levels via finger prick, and provided with a FreeStyle Optium ketone meter and ketone strips. They were instructed to measure their blood ketone levels daily between 2 pm and 4 pm. Nutritional ketosis was defined as a blood ketone (beta-hydroxybutyrate) level > 0.5 mmol/l.
- Anthropometric testing. Body weight and skinfolds were measured by an ISAK level one accredited anthropometrist, prior to and at the conclusion of the intervention, at the same time of the day. A sum of (∑) 8-site skinfold ISAK protocol was applied: triceps, biceps, subscapular, iliac crest, supraspinale, abdominal, front thigh and medial calf [ 12 ].
- Interviews and focus group. A 20–30 min individual interview was conducted on the phone during week 5 week and a 60-min focus group was conducted in person once the intervention had concluded. The importance of this qualitative work was to assess both the individual and the groups’ overall experiences of being on this diet for 10 weeks. The group session provided a chance for athletes to compare experiences and translate findings to future practice.
Data analysis
Due to the explorative nature of this study, and our small sample size, quantitative data is presented as individual responses. Data was analysed using mean change scores, with Cohen’s effect sizes and associated confidence limits applied to quantify magnitude of change. We also elected to apply a probability statistic using a student’s t-test to determine the statistical meaning of the change. All statistics were generated and applied using Microsoft Excel 2016. We acknowledge that applying statistical models hold limited meaning in this context and it is not our intention to make any inferences about these outcomes to athlete populations. As such, outcomes with a significance level of p < 0.05 should be considered a trend only; and p < 0.01 significant only in the sense that further work is required to substantiate these findings. Interviews and the focus group were recorded and transcribed, after which data was analysed using thematic analysis. The data for the interviews and focus groups were combined and is presented as key themes with supporting transcripts.
Diet and ketosis
The diets were geared to induce nutritional ketosis, which all the athletes achieved by the end of week 2. All athletes adhered to the macronutrient thresholds provided for the 10 weeks, apart from on two occasions during the first two weeks, once, where protein intake exceeded the recommended threshold over several days in one athlete and alcohol intake was excessive in another athlete, thereby preventing ketosis from being achieved. Table Table2 2 presents an example of a day’s food intake for each participant along with the average energy and macronutrient breakdown for that day. The sample day was selected at random during week 5. The athletes varied little from the sample diets provided to them throughout the 10 weeks, with adherence to the diets verified by blood ketones always staying above 0.5 mmol/l from week 2 onwards. Blood ketones ranged from 0.5–4.2 mmol/l; females ranged 0.5–1.9 mmol/l, and never exceeded 1.9 mmol/l. The male athlete ranged 1.0–3.5 mmol/l, went below 1.0 mmol/l on two occasions (0.8 and 0.6 mmol/l) and measured 4.2 mmol/l on one occasion.
Participants’ dietary data and macronutrient composition
a granola was made in a batch and consisted of a range of nuts, seeds, coconut threads and coconut oil
b egg muffins
c T = tablespoon
d tsp. = teaspoon
Substrate oxidation
Figure Figure1 1 presents the pre and post intervention fuel utilisation (also termed metabolic efficiency) curves, along with associated peak fat oxidation (g/min) and fat max (%Wmax) for each participant.

Pre, and post intervention metabolic efficiency curves for each participant. * PF ox : Peak Fat Oxidation (g/min). † Fat max : Maximum fat oxidation at % WR max
Each curve displays the cross-over point i.e., the point at which peak fat oxidation is reached, and the point at which carbohydrate takes over as the predominant fuel source. Mean peak absolute fat oxidation and standard deviation (SD) increased by 41.3% (0.6 ± 0.1 to 0.8 ± 0.1 g.min −1 , p = 0.001). All of the athletes increased their peak fat oxidation. The exercise intensity relative to V . O 2max at which peak absolute fat oxidation occurred (Fat max ) increased by 31.2% from pre- to post-intervention (48.2 ± 8.7 to 63.2 ± 5.7% V . O 2max , p = 0.06). Similarly, Fat max relative to WR max increased by 21.5% from pre- to post-intervention (39.5 ± 11.9 to 48.0 ± 8.9%WR max , p = 0.18). Two out of the five athletes showed an increase in Fat max relative to WR max , and the remaining three showed no change.
Body composition/performance
Figure Figure2 2 shows individual pre and post scores for anthropometric and performance data. All five participants had reductions in body weight and skinfolds. The mean loss in weight and SD was −4.0 ± 3.1 kg ( p = 0.046), and in skinfolds was −25.9 ± 6.9 mm ( p = 0.001). The skinfold outcome showed a large effect size (1.27) with associated confidence limits not crossing zero, together indicating clinical significance in the context of their limited application to this data.

Individual responses and effect sizes of anthropometry and performance variables
All participants showed a decrease in time to exhaustion (TTE), the mean time and SD reduction was 2 ± 0.7 min ( p = 0.004). More varied responses were noted in the change in VO2 Max (mean change −1.69 ± 3.4 ml.kg.min ( p = 0.63)), peak power (mean change −18 ± 16.4 W ( p = 0.07)) and ventilatory threshold, VT2 (mean change −6 ± 44.5 W ( p = 0.77)), with some athletes improving (VT2 and VO2 max) or staying the same (VT2 and peak power).
Athlete experiences
Table Table3 3 presents the key positive and negative themes extracted from the focus group. Overall, there were more positive themes than negative; with an improved sense of general health and well-being coming through as a strong theme. The majority of the negative experiences related to tiredness, experienced in the first few weeks of the intervention, and a feeling of loss of power, experienced towards the last few weeks. Each athlete reported the following subjective parameters: enhanced well-being, improved recovery, improved skin conditions and reduced inflammation.
Themes from interviews and focus group with supporting transcripts
This study presents a real-life insight into the lives of five seasonal endurance athletes who by virtue of their own curiosity wished to experience the effects of a ketogenic diet on their sports performance. Overall, participants were able to increase the substrate utilisation of free fatty acids, reduce body fat and experience positive health benefits, but their maximal aerobic performance was compromised.
Body composition
The reduced body fat can likely be explained by a resultant calorie deficit created by the diet, as participants reported enhanced feelings of satiety and a reduction in overall food intake. This outcome was unsurprising and comparable to findings in previous research on both strength and endurance athletes [ 13 – 15 ]. Initial weight reduction can be associated with a loss in body water through glycogen depletion, [ 16 , 17 ], and this was also likely the case in this study; however fat loss was evident as per skinfold changes. A further theory relating to weight loss, which is as yet, rigorously tested, is an increased drive for fat breakdown rather than storage as circulating levels of insulin remain low during ketogenic diets [ 18 ]. Perhaps a combination of all three mechanisms can explain the weight loss. A limitation of the study was a lack of energy comparison prior to, and during the study, which would have provided some clarity about these mechanisms.
Metabolic efficiency
All participants had a greater fatty acid oxidation at a higher given intensity at the end of the trial compared to baseline. This finding of enhanced fat utilisation aligns with those of several other groups that have incorporated ketogenic and non-ketogenic dietary protocols [ 5 , 19 , 20 ]. Furthermore, this substrate utilisation alteration can be attributed to the change in diet as training was kept relatively consistent throughout the intervention. Our participants also had a higher oxygen cost at sub-maximal workloads due to the higher use of fat as an energy substrate. However, this did not benefit exercise capacity.
Performance
On the whole, maximal aerobic performance was reduced, another comparable outcome to similar research [ 21 – 23 ], the exception being one athlete in Phinney et al.’s 4-week cycling study [ 5 ], and Zajac et al.’s eight off-road cyclists [ 6 ], who all showed performance increases. The performance decrement in our study, and others, is likely due to changes in metabolic pathways that impair glycogen metabolism at higher exercise intensities [ 24 – 26 ]. Specifically, a down-regulation of the carbohydrate oxidative enzyme, pyruvate dehydrogenase (PDH), which via conversion of pyruvate to acetyl-coenzyme A, links the glycolytic pathway with the Krebs cycle [ 27 ]. PDH is said to be reduced rapidly through a reduction in circulating insulin and an increase in circulating levels of free fatty acids [ 28 ]. Evidence suggests that PDH is upregulated upon carbohydrate reintroduction [ 25 ]; however, there is little insight into its fate along with other mitochondrial enzymes in the context of low carbohydrate availability. Despite similarities in findings with other studies, some of these studies are limited by short-duration low carbohydrate diets [ 28 – 30 ]. Future research with chronically fat-adapted athletes is needed to investigate these micro-level mechanisms alongside performance outcomes. Recently, Volek et al.’s work [ 19 ] with chronically fat-adapted ultra-endurance athletes (>6 months) not only demonstrated a 2.3 times greater fat oxidation rate in the LCHF group compared with the mainstream dietary group, but also demonstrated no difference in resting and replete muscle glycogen stores between groups. Authors suggest a homeostatic muscle glycogen repletion mechanism arising from hepatic gluconeogenesis, which might serve to provide clues into why many athletes report optimal performance, anecdotally, when having eaten in an LCHF manner for extended periods of time. While this is a plausible speculation, a similar study by Webster et al., [ 20 ] showed no difference in gluconeogenic rates during exercise in fasting LCHF and mixed-diet athletes. In fact, glucose was produced endogenously to a greater extent in the mixed diet group, and was attributed to greater rates of hepatic glycogenolysis. Researchers concluded that gluconeogenesis during exercise may remain stable across a range of dietary regimes after an overnight fast, but that hepatic glycogenolysis is influenced by dietary carbohydrate. Further exploration of fuel contributions to gluconeogenesis and the effect of different feeding protocols on endogenous glucose producing mechanisms is warranted. It is important to note that both of these studies did not incorporate a performance measure, leaving the questions to this key issue unanswered [ 19 , 20 ].
This is one of the few studies to report specifically on endurance athletes’ experiences of undertaking a ketogenic diet. Athletes reported similar negative physiological experiences to those reported by athletes in comparable ketogenic diet studies [ 13 , 14 , 31 ]. However, they also reported experiencing benefits throughout the trial. One of these benefits was enhanced recovery; possibly, the rise in blood ketones had some influence, as beta-hydroxybutyrate has been associated with upregulating antioxidant gene expression and decreasing reactive oxygen species [ 32 ]. However, further research is required to substantiate this within athletic populations.
From a physical well-being perspective, the cases of improved skin, and the resolution of an ongoing prostate issue, were major points of discussion of benefits experienced. We speculate that it is the reduction of systemic inflammation as a result of a lower total sugar [ 33 ] and Omega 6 fatty acid intake, thereby rebalancing the Omega 6:3 fatty acid ratio in an anti-inflammatory direction [ 34 ] that gave rise to these outcomes. All participants were consuming high-Omega 6 industrial seed oils prior to the study (used as cooking fat and derived from processed foods). During the study these fats were replaced with coconut oil, butter and olive oil; i.e., fats containing minimal Omega 6 fatty acid content.
Being a translational study, we followed up participants informally 12 months after the study concluded. They were all still competing in endurance events, and while not eating a ketogenic diet, none of them had returned to their previous high carbohydrate, low fat style of eating. Collectively, they reported that once the study concluded they gradually increased their carbohydrate intake until the point at which they felt their performance at high intensities return. They were still restricting carbohydrate and eating more fat than mainstream guidelines recommend, and reported having discovered the optimal macronutrient ratio that satisfied a performance, body composition and a health goal.
This study had several limitations: Its design as a pilot case study, with no standardisation of training prevents any inference from being made to athletic populations. However, it is still relevant to both the researcher and the practitioner as it provides insights into what is considered important for athletes, particularly those in the 40+ age range. i.e., alongside improving performance, they are also more cognisant of their overall health and well-being. A lesson learned from undertaking this research, and a key consideration for researchers and practitioners, is to encourage a reduction in athlete training intensity and volume in the early weeks of embarking on a ketogenic diet. This will likely induce less early fatigue and other negative symptoms related to training, and allow for metabolic adaptations to occur in a lower stress milieu.
Despite a decrease in performance, athletes reduced body fat and experienced unexpected well-being benefits. While performance outcomes are key to the field of sports science and medicine, what might be overlooked, is the integration of health and well-being, alongside performance. Further research, both conceptual and translational, should challenge this type of diet further to understand its potential uses to achieve what an endurance athlete and their support team should ultimately be striving for i.e., optimal body composition, health and performance.
Acknowledgements
This study was funded by AUT, Sports Performance Research Institute, New Zealand. The funding body had no input into any aspect of the study.
Availability of data and materials
Abbreviations, authors’ contributions.
The study was designed by CZ and MaW; data were collected and analysed by CZ, MaW, EM, and MW; data interpretation and manuscript preparation were undertaken by CZ, MaW, MW and SC. All authors approved the final version of the paper.
Ethics approval and consent to participate
Ethics was approved by the AUT Ethics Committee (application 15/415).
Consent for publication
All participants provided their consent for their individual data to be published.
Competing interests
The corresponding author, CZ, has co-authored two books called “What The Fat? - Fat’s in, Sugar’s out”, and “What The Fat – Sports performance”. These books were constructed and published after the study was concluded.
Publisher’s Note
Springer Nature remains neutral with regard to jurisdictional claims in published maps and institutional affiliations.
Contributor Information
Caryn Zinn, Phone: +64 9 921 9999, Email: [email protected] .
Matthew Wood, Email: [email protected] .
Mikki Williden, Email: moc.liamg@nedilliwm .
Simon Chatterton, Email: moc.liamtoh@ttahc_nomis .
Ed Maunder, Email: moc.liamg@rednuamnje .
- Alzheimer's disease & dementia
- Arthritis & Rheumatism
- Attention deficit disorders
- Autism spectrum disorders
- Biomedical technology
- Diseases, Conditions, Syndromes
- Endocrinology & Metabolism
- Gastroenterology
- Gerontology & Geriatrics
- Health informatics
- Inflammatory disorders
- Medical economics
- Medical research
- Medications
- Neuroscience
- Obstetrics & gynaecology
- Oncology & Cancer
- Ophthalmology
- Overweight & Obesity
- Parkinson's & Movement disorders
- Psychology & Psychiatry
- Radiology & Imaging
- Sleep disorders
- Sports medicine & Kinesiology
- Vaccination
- Breast cancer
- Cardiovascular disease
- Chronic obstructive pulmonary disease
- Colon cancer
- Coronary artery disease
- Heart attack
- Heart disease
- High blood pressure
- Kidney disease
- Lung cancer
- Multiple sclerosis
- Myocardial infarction
- Ovarian cancer
- Post traumatic stress disorder
- Rheumatoid arthritis
- Schizophrenia
- Skin cancer
- Type 2 diabetes
- Full List »
share this!
June 10, 2021
Case study shows patient on ketogenic diet living fully with IDH1-mutant glioblastoma
by Boston College
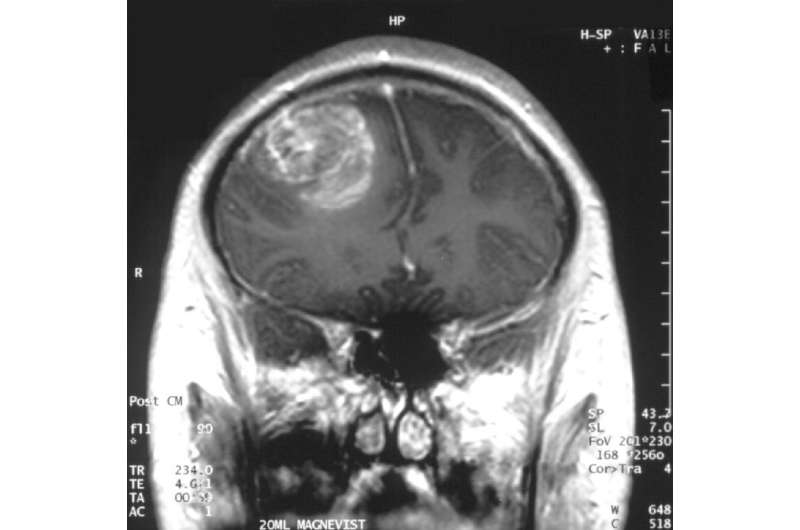
A British man who rejected the standard of care to treat his brain cancer has lived with the typically fatal glioblastoma tumor growing very slowly after adopting a ketogenic diet, providing a case study that researchers say reflects the benefits of using the body's own metabolism to fight this particularly aggressive cancer instead of chemo and radiation therapy.
Published recently in the journal Frontiers in Nutrition, the report is the first evaluation of the use of ketogenic metabolic therapy (KMT) without chemo or radiation interventions, on a patient diagnosed with IDH1-mutant glioblastoma (GBM). Ketogenic therapy is a non-toxic nutritional approach, viewed as complementary or alternative, that uses a low-carbohydrate, high-fat diet to manage a range of cancers, including glioblastoma.
In this particular case, the patient's tumor contained a mutation, known as the IDH1. This mutation is acquired by chance and is known to improve overall survival. So the findings are particularly relevant to other patients whose tumors contain this mutation, according to Boston College Professor of Biology Thomas Seyfried, a co-author of the report and a leading researcher who has long advocated the benefits of KMT to treat disease.
"As GBM, like most malignant cancers, is dependent on fermentation for energy synthesis and survival, the simultaneous restriction of fermentable fuels, such as glucose and glutamine, while elevating non-fermentable ketone bodies, offers a non-toxic therapeutic strategy for managing GBM," said Seyfried. "Further studies will be needed to test this hypothesis in other patients diagnosed with GBM."
Glioblastoma kills about 15,000 people each year and remains largely unmanageable. While the standard of care has shifted to new immunotherapies, the median survival time of 11 to 15 months for GBM has not improved significantly for more than 100 years, according to the co-authors.
A number of high-profile cases have illustrated the deadly progression of GBM. The late U.S. Sen. John McCain was diagnosed at age 80 with glioblastoma in July 2017, and died in August 2018. U.S. Sen. Edward M. Kennedy lived for a year after his diagnosis with GBM, dying at age 77. Beau Biden, son of U.S. President Joe Biden, was diagnosed at age 44 with GBM in August 2013, and died in May 2015.
The current standard of care for GBM involves surgical resection, radiation, and chemotherapy, which have been shown in studies to result in significant toxicity. However, large-scale clinical trials for alternative therapies are difficult to initiate, leading researchers to carefully examine individual cases.
The patient in this case was diagnosed with glioblastoma in 2014 and eschewed the traditional standard of care and instead embarked on a self-directed ketogenic diet—low in carbohydrates and high in fat—in an effort to manage his cancer.
The researchers found "the patient's tumor continued to grow very slowly over a three-year period without expected vasogenic edema until 2017," according to Seyfried and his co-authors, Boston College researcher Purna Mukherjee, Aditya Shivane, MD, of University Hospital Plymouth NHS Trust, in the United Kingdom, U.S.-based nutritionist Miriam Kalamian, Joseph Maroon, MD, of the University of Pittsburgh, and Giulio Zuccoli, MD, of the Drexel University School of Medicine
At that point, the patient underwent "surgical debulking" of his tumor. The pathology specimen confirmed the diagnosis of GBM and that the patient's tumor also contained the IDH1 mutation.
"Following surgery, the patient continued with a self-administered ketogenic diet to maintain low Glucose Ketone Index (GKI) values, indicative of therapeutic ketosis," the researchers report. "In light of continued slow progression of the residual tumor, the patient intensified his KMT starting in October, 2018 with inclusion of mindfulness techniques to reduce stress. While Magnetic Resonance Imaging (MRI) shows slow interval tumor progression, the patient remains alive with a good quality of life at the time of this report." He is now at 82 months from original diagnosis.
"We were surprised to discover that KMT could work synergistically with the IDH1 mutation to simultaneously target the two major metabolic pathways needed to drive the growth of GBM," said Seyfried. "Glucose drives the glycolysis pathway, while glutamine drives the glutaminolysis pathway."
He added: "No tumor, including GBM, can survive without glucose and glutamine. Our study has identified a novel mechanism by which an acquired somatic mutation acts synergistically with a low-carbohydrate, high-fat diet to provide long-term management of a deadly brain tumor."
Despite the compelling interest in such individual case studies, the co-authors note, "We cannot predict if the therapeutic response to KMT seen in our GBM patient will also be seen in other similarly treated GBM patients. For those GBM patients not fortunate enough to have acquired the spontaneous IDH1 mutation in their tumor, glutamine targeting drugs used with KMT will be necessary to reduce tumor growth."
The researchers noted that additional studies they've conducted have shown that the simultaneous targeting of glucose and glutamine availability, using KMT and the a pan-glutaminase inhibitor—known as 6-diazo-5-oxo-L-norleucine (DON)—can significantly prolong survival in preclinical syngeneic glioblastomas in mice. Earlier research has shown that ketogenic diets can facilitate delivery of small-molecule therapeutic drugs through the blood brain barrier without toxicity.
Explore further
Feedback to editors

Research shows 'profound' link between dietary choices and brain health
20 hours ago

Component of keto diet plus immunotherapy may reduce prostate cancer
Apr 27, 2024

Study finds big jump in addiction treatment at community health clinics

Positive childhood experiences can boost mental health and reduce depression and anxiety in teens

Gene linked to epilepsy and autism decoded in new study
Apr 26, 2024

Blood test finds knee osteoarthritis up to eight years before it appears on X-rays

Researchers find pregnancy cytokine levels impact fetal brain development and offspring behavior

Study finds biomarkers for psychiatric symptoms in patients with rare genetic condition 22q

Clinical trial evaluates azithromycin for preventing chronic lung disease in premature babies

Scientists report that new gene therapy slows down amyotrophic lateral sclerosis disease progression
Related stories.

Cancer-fighting combination targets glioblastoma
May 30, 2019

Lower-carb diet slows growth of aggressive brain tumor in mouse models
Apr 14, 2016

A breakthrough for understanding glioblastoma—origin cells for deadly brain tumors identified
Aug 2, 2018

Researchers clarify role of mutations in glioblastoma
Jul 11, 2018

Research going keto to fight cancer
Sep 30, 2019

Brain tumor surgery that pushes boundaries boosts patients survival
Feb 6, 2020
Recommended for you

Study identifies driver of liver cancer that could be target for treatment

Analysis identifies 50 new genomic regions associated with kidney cancer risk

Biomarkers identified for successful treatment of bone marrow tumors

Study finds vitamin D alters mouse gut bacteria to give better cancer immunity
Apr 25, 2024

Targeting specific protein regions offers a new treatment approach in medulloblastoma
Let us know if there is a problem with our content.
Use this form if you have come across a typo, inaccuracy or would like to send an edit request for the content on this page. For general inquiries, please use our contact form . For general feedback, use the public comments section below (please adhere to guidelines ).
Please select the most appropriate category to facilitate processing of your request
Thank you for taking time to provide your feedback to the editors.
Your feedback is important to us. However, we do not guarantee individual replies due to the high volume of messages.
E-mail the story
Your email address is used only to let the recipient know who sent the email. Neither your address nor the recipient's address will be used for any other purpose. The information you enter will appear in your e-mail message and is not retained by Medical Xpress in any form.
Newsletter sign up
Get weekly and/or daily updates delivered to your inbox. You can unsubscribe at any time and we'll never share your details to third parties.
More information Privacy policy
Donate and enjoy an ad-free experience
We keep our content available to everyone. Consider supporting Science X's mission by getting a premium account.
E-mail newsletter

IMAGES
VIDEO
COMMENTS
The ketogenic diet is a high-fat, low-carbohydrate diet that induces ketone body production through fat metabolism with the goal of mimicking a fasting state, shifting the predominant caloric source from carbohydrate to fat [ 1, 2 ]. The classic ketogenic diet is typically composed of a 4: 1 ratio (in grams) of fat to protein and carbohydrates.
Mohorko et al. conducted a 12-week ketogenic diet study on obese patients who were calorie restricted (1200-1500 kcal) for the first two weeks and then were instructed to eat ad-libitum for hunger for the remaining weeks while eating the macronutrient composition necessary to remain in a state of nutritional ketosis. BHB was measured ...
A century ago, the ketogenic diet was a standard of care in diabetes, used to prolong the life of children with type 1 diabetes and to control the symptoms of type 2 diabetes in adults ().Because all forms of diabetes share a basic pathophysiological problem, carbohydrate intolerance, restriction of carbohydrate on a ketogenic diet (typically ≤50 g/d with >70% fat) often produced rapid and ...
2.1. Data Sources. A systematic literature search of Embase, PubMed, and Cochrane Library was performed to identify randomized controlled trials (RCTs) (published until 11 February 2020) of patients with overweight or obesity and with or without T2DM that evaluated the effects of a ketogenic diet on glycemic control, weight loss, and lipid profile as primary endpoints.
This case study explores the relationship between acute pancreatitis and the ketogenic diet, a dietary approach characterized by low carbohydrate and high fat intake. The report details the experience of a 47-year-old woman who developed intense abdominal pain and vomiting following her self-prescribed ketogenic diet for weight loss.
Systematic reviews and meta-analyses of randomized clinical trials (RCTs) have reported the benefits of ketogenic diets (KD) in various participants such as patients with epilepsy and adults with overweight or obesity. Nevertheless, there has been little synthesis of the strength and quality of this evidence in aggregate. To grade the evidence from published meta-analyses of RCTs that assessed ...
A ketogenic diet primarily consists of high fat intake, moderate protein consumption, and low carbohydrate intake. The macronutrient distribution typically ranges from approximately 55% to 60% fat, 30% to 35% protein, and 5% to 10% carbohydrates. For instance, in a 2000 kcal per day diet, the carbohydrate allowance would amount to approximately ...
A British man who rejected the standard of care to treat his brain cancer has lived with the typically fatal glioblastoma tumor growing very slowly after adopting a ketogenic diet, providing a case study that researchers say reflects the benefits of using the body's own metabolism to fight this particularly aggressive cancer instead of chemo and radiation therapy.
This meta-study employed the meta-analysis model of random effects or of fixed effects to analyze the average difference before and after KD and the corresponding 95% CI, thereby evaluating the ...
A 2013 meta-analysis of randomized controlled trials testing very-low-carbohydrate ... p < 0.0001); this was not the case with the low-fat diet (−0.16 ± 0.27 kg; ... For example, in one study of a ketogenic diet for type 2 diabetes, researchers encouraged unlimited meat, poultry, seafood, and eggs, while cutting intake of whole ...
Low carbohydrates diets (LCDs), which provide 20-120 g of carbohydrates per day, have long been used as therapeutic options in the treatment of severe obesity, type 2 diabetes mellitus and other morbid conditions, with good results in terms of weight loss and control of the main metabolic parameters, at least in the short and medium term. According to the caloric content and the ...
Ketogenic diet therapy (KDT) is an established nonpharmacologic treatment in various types of epilepsy. We aim to evaluate the quality of the systematic reviews and meta-analyses (SRMAs) of KDT ...
The ketogenic diet (KD) is a high-fat, adequate-protein, and very-low-carbohydrate diet regimen that mimics the metabolism of the fasting state to induce the production of ketone bodies. The KD ...
In the Yale study, published in the Jan. 20 issue of Nature Metabolism, researchers found that the positive and negative effects of the diet both relate to immune cells called gamma delta T-cells, tissue-protective cells that lower diabetes risk and inflammation. A keto diet tricks the body into burning fat, said lead author Vishwa Deep Dixit ...
A small clinical trial led by Stanford Medicine found that the metabolic effects of a ketogenic diet may help stabilize the brain. April 1, 2024 - By Nina Bai. A study led by researchers at Stanford Medicine showed that diet can help those with serious mental illness. nishihata. For people living with serious mental illness like schizophrenia ...
5. ). Next, Ludwig minimizes the concerns of the increase in LDL cholesterol seen in those consuming a ketogenic diet, a concern also shared by the National Lipid Association (. 4. ). Indeed, nearly every study examining participants on a ketogenic diet has shown an increase in LDL cholesterol, apolipoprotein-B, or both (. 6.
Background: The ketogenic diet (KD) has been reported to play an important role in the development of cancer by an abundance of pre-clinical experiments; however, their conclusions have been controversial. We therefore aimed to perform a systematic review and meta-analysis of animal studies evaluating the effects of KD on cancer.Methods: Relevant studies were collected by searching PubMed ...
This case study represents the first documented occurrence of a patient with ALS managed with either a fasting or ketogenic diet protocol, co-administered as a TRKD. We measured improved or stabilized ALS-related function, forced expiratory volume, forced vital capacity, swallowing, neurocognitive status, mood, and quality of life.
Methods. Using a case study design, five New Zealand endurance athletes (4 females, 1 male) underwent a 10-week ketogenic dietary intervention. Body composition (sum of 8 skinfolds), performance indicators (time to exhaustion, VO 2 max, peak power and ventilatory threshold), and gas exchange thresholds were measured at baseline and at 10 weeks. Mean change scores were calculated, and analysed ...
The patient in this case was diagnosed with glioblastoma in 2014 and eschewed the traditional standard of care and instead embarked on a self-directed ketogenic diet—low in carbohydrates and ...
The study also shows that the ketogenic diet is one of the useful dietary intervention approaches used to change eating habits that will show results relatively quickly. ... The association between Helicobacter pylori and obesity: A systematic review and meta-analysis of case-control studies. Clin. Diabetes Endocrinol. 2021, 7, 15.
However, possibly the first case of ketoacidosis in a nondiabetic patient was reported in 2015, in a lactating woman on a ketogenic diet. [11]. A later case study purported to be the first of a healthy, nondiabetic, and non-lactating woman experiencing ketoacidosis. [12]
Diet Analysis Case Study For the Keto Diet Kerry, a 21 year old college student, would like to lose 30 pounds after gaining weight the first few years of college. Upon seeing the weight loss success of some of her family members on the Keto diet, she decides to try it herself. Here is Kerry's information: Height: 5'5" Current Weight: 158 ...
A new study in female rat models, conducted by researchers at the University of California, Davis, has found that following the ketogenic — or keto diet, for short — may significantly decrease ...