
An official website of the United States government
The .gov means it’s official. Federal government websites often end in .gov or .mil. Before sharing sensitive information, make sure you’re on a federal government site.
The site is secure. The https:// ensures that you are connecting to the official website and that any information you provide is encrypted and transmitted securely.
- Publications
- Account settings
Preview improvements coming to the PMC website in October 2024. Learn More or Try it out now .
- Advanced Search
- Journal List


Recent Reports of Solid-Phase Cyclohexapeptide Synthesis and Applications
Macrocyclic peptides are privileged scaffolds for drug development and constitute a significant portion of macrocyclic drugs on the market today in fields spanning from infectious disease to oncology. Developing orally bioavailable peptide-based drugs remains a challenging task; however, macrocyclization of linear peptides can be an effective strategy to improve membrane permeability, proteolytic stability, oral bioavailability, and overall drug-like characteristics for this class. Significant advances in solid-phase peptide synthesis (SPPS) have enabled the efficient construction of macrocyclic peptide and peptidomimetic libraries with macrolactamization being performed on-resin or in solution phase. The primary goal of this review is to summarize solid-phase cyclohexapeptide synthesis using the on-resin and solution-phase macrocyclization methodologies published since 2013. We also highlight their broad applications ranging from natural product total synthesis, synthetic methodology development, and medicinal chemistry, to drug development and analyses of conformational and physiochemical properties.
1. Introduction
Macrocyclic peptides constitute a significant portion of macrocyclic drugs on the market today and are used in many fields ranging from infectious disease to oncology [ 1 , 2 ]. Recently, reports of antibacterial macrocyclic peptide natural products have demonstrated that macrocyclic peptides are privileged scaffolds for drug development [ 3 ]. Historically, the pharmaceutical industry has been cautious with developing macrocyclic drugs because of concerns of higher cost and synthetic challenges associated with lead optimization and scale-up campaigns [ 1 ]. Head-to-tail cyclization of linear peptides of three to eight amino acids can be challenging, particularly for peptides containing exclusively the L-configuration [ 4 ]. The formation of linear as well as cyclic dimers and oligomers during the cyclization step can compete with head-to-tail cyclization [ 5 ]. Additionally, epimerization of the C -terminal amino acid is commonly encountered during the C -terminal activation step before the cyclization reaction takes place. To date, significant progress has been made in the synthesis of macrocycles, making their efficient construction more feasible [ 1 ]. A common strategy to improve head-to-tail cyclization of small peptides involves the incorporation of the “turn-inducing” functionality into a linear peptide sequence such as glycine, proline, pseudoproline, N -alkyl, or D-amino acid residue [ 4 , 6 , 7 , 8 ]. In the case of the pseudoproline method, a pseudoproline residue, synthesized by the condensation of serine, threonine, or cysteine with an aldehyde or ketone, is incorporated into the peptide sequence pre-cyclization. The pseudoproline residue can then be deprotected post cyclization [ 6 , 7 ]. Coupling reagents in the azabenzotriazole class are the most commonly employed for cyclization of linear peptide precursors, as they give faster rates of cyclization with lower amounts of epimerization, typically less than 10% [ 4 ]. Moreover, linear peptides with the D-configuration at their C -terminal residue have favorable cyclization kinetics [ 4 ]. This may be a result of less steric hindrance during the formation of the peptide bond occurring between the D- and L-amino acids, particularly when bulky side chains are present. Cyclodepsipeptides, with one ester linkage in the macrocyclic backbone [ 9 ], have been utilized in the epimerization-free synthesis of cyclopeptides by employing a key O - N -acyl migration reaction at a serine residue [ 10 , 11 ].
Macrocyclic peptides are known to possess some improved membrane permeability [ 12 , 13 ], proteolytic stability [ 13 , 14 , 15 , 16 ], oral bioavailability [ 17 , 18 ], and overall drug-like characteristics [ 13 , 19 , 20 ] over their linear analogues. Moreover, peptide macrocyclization is a way of locking the peptide sequence in a β-strand conformation [ 15 , 16 ], a conformation often recognized by their enzyme targets, such as proteases [ 15 , 16 , 21 ]. Macrocycles are not completely rigid but still have a degree of flexibility, which facilitates interactions with their receptors [ 1 ]. Additionally, the entropic cost of receptor binding may be reduced for macrocyclic drugs compared to their linear equivalents as a result of conformational pre-organization [ 1 ]. N -Methylation of the cyclic peptide backbone has been shown in some cases to improve peptide metabolic stability [ 14 , 22 ] and oral bioavailability [ 23 ] as a result of changes in peptide conformation or steric hindrance [ 22 ]. The immunosuppressant drug ciclosporin is a macrocyclic undecapeptide bearing seven N -methylated motifs and can be administered as an oral formulation [ 1 , 22 ]. Modification of the cyclohexapeptide backbone to form cyclohexapeptoids can, in some cases, lead to an increase to cell permeability [ 24 ]. Most cyclic peptide drugs on the market today are administered parenterally, and only few are orally bioavailable [ 1 ], highlighting an ongoing challenge. For this reason, the synthesis and evaluation of novel cyclopeptide scaffolds to expand our understanding of their pharmacokinetic (PK) properties is still at the forefront of many research programs [ 2 , 12 , 23 ].
Solid-phase peptide synthesis (SPPS) was first described in 1963 by Merrifield [ 25 ], whereby a growing linear peptide was synthesized in a step-wise fashion while covalently attached to a solid support (resin). In general, excess reagents are used in solid-phase synthesis to help to drive reactions to completion. The solid-supported methodology allows excess reagents to be removed after each step by employing a simple filtration, and the final desired product is obtained after cleavage from the resin [ 25 ]. To date, many new advances in SPPS have allowed for the rapid and efficient construction of peptide or peptidomimetic [ 26 ] libraries for subsequent biological evaluation and high-throughput screening [ 27 , 28 ].
The current literature base surrounding natural and synthetic macrocyclic peptides is extensive. The structures of these macrocycles have a wide-ranging incorporation of natural and unnatural amino acids as well as different ring sizes. Interestingly, cyclohexapeptides—macrocyclic peptides comprising six amino acids in the ring—are one of the most ubiquitous classes of macrocyclic peptides synthesized by SPPS. Macrocyclic hexapeptides can be obtained directly from on-resin or solution-phase cyclization following the cleavage of a linear hexapeptide precursor from resin ( Figure 1 ). This brief review summarizes the preceding five years’ worth of solid-phase cyclohexapeptide synthesis and its applications, which span from natural product total synthesis, synthetic methodology development, and medicinal chemistry, to drug development and analyses of conformational and physiochemical properties.

( A ). Illustration of solid-phase cyclohexapeptide synthesis. ( B ). Resins highlighted in this review.
2. Solid-Phase Synthesis of Cyclohexapeptides Using Solution-Phase Cyclization
In 2013, Wu et al. reported the structures of two new cyclohexapeptides, nocardiamides A ( 1 ) and B ( 2 ), that were isolated from a culture broth of a CNX037 strain of actinomycete, which is a Nocardiopsis species ( Figure 2 ) [ 29 ].

Structures of naturally occurring nocardiamides A ( 1 ) and B ( 2 ).
Their structures were confirmed through an independent total synthesis, which helped to confirm the location of the two D- and L-Val residues. The synthesis of 1 and 2 is shown in Scheme 1 . Their synthesis used 2-chlorotrityl chloride (2-CTC) resin as a solid support to construct the linear hexapeptides 3 and 4 using classical SPPS, followed by cleavage from the resin using a trifluoroacetic acid TFA-based cleavage cocktail solution. Subsequent N,N,N′,N′- tetramethyl- O -(1 H -benzotriazol-1-yl)uronium hexafluorophosphate (HBTU)-mediated solution-phase cyclization of 3 or 4 provided cyclohexapeptides 1 and 2 in 7.2% and 10.7% yields, respectively. Antimicrobial evaluation of 1 – 4 was performed against Escherichia coli , Staphylococcus aureus , Enterococcus faecalis , Bacillus thuringiensis , Bacillus subtilis , Micrococcus luteus , and Candida albicans ; however, only negligible activity was found. Compounds 1 and 2 were also screened against a human colon carcinoma cell line, HCT-116, and no cell cytotoxicity was observed [ 29 ].
In 2013, Cochrane et al. reported the synthesis of the first members of a new class of cyclic-peptide-containing hemicryptophanes (e.g., 8 in Scheme 2 ) [ 30 ]. From this work, the solid-supported linear hexapeptide 5 was prepared from 2-CTC resin by standard microwave-assisted 9-fluorenylmethoxycarbonyl (Fmoc) SPPS ( Scheme 2 ). Cleavage of the hexapeptide from the resin was done using 5% TFA in CH 3 CN/H 2 O (4:1), affording the unprotected linear peptide 6 in 85% yield. Subsequent cyclization of 6 using (benzotriazol-1-yloxy)tripyrrolidinophosphonium hexafluorophosphate (PyBOP) in dimethylformamide (DMF) gave the cyclohexapeptide 7 in quantitative yield after 1 h. Interestingly, cyclization of 6 proceeded much faster than that of its corresponding O -tert-butyl protected linear peptide counterpart. Cyclic hexapeptide 7 was used to produce a new class of cyclohexapeptide-containing hemicryptophanes, which were investigated for their enantioselective binding properties by complexation with carnitine [ 30 ].
Peptide-based therapeutics are notorious for their poor oral bioavailability profiles and low plasma stability, which have limited their use as orally delivered drugs. Although N -methylation of cyclohexapeptides was shown to improve oral bioavailability [ 23 ], Hill et al. demonstrated that cyclohexaleucine peptides 9 and 10 without N -methylation functionality showed some degree of oral bioavailability: 17% and 9%, respectively [ 12 ]. Interestingly, the epimer 10 showed ~2-fold lower oral bioavailability than 9 as a result of its notably higher plasma clearance rate (24.1 versus 4.7 mL/min/kg). The reason was suggested to be caused by differences in solvent exposure to the peptide backbone resulting from the conformational change at a Leu residue. Membrane permeability was measured using RRCK and CACO-2 cell monolayers, the former having a lack of active transporters. In the RRCK assay, compounds 9 and 10 showed 2–3-fold greater membrane permeability than the control standard cyclosporin A (CsA). In the CACO-2 assay, 9 and 10 showed similar permeability to CsA (P app ≈ 5 × 10 −6 cm·s −1 ) [ 12 ]. As shown in Scheme 3 , the cyclohexaleucine peptide 9 was synthesized using SPPS on 2-CTC resin as a linear hexapeptide precursor, followed by cleavage from resin and solution-phase cyclization under dilute conditions. Epimer 10 was formed during the final cyclization step but could be successfully separated out during purification using reverse-phase high-performance liquid chromatography (RP-HPLC). The observed epimerization was due to a well-known epimerization process that takes place during the carboxylic acid activation step prior to cyclization [ 31 , 32 ]. In this example, the epimer ratio of 9 and 10 was 85:15 [ 12 ].
In 2014, Masuda et al. reported the total synthesis and insecticidal activity of the cyclohexapeptide natural products PF1171A ( 11 ), C ( 12 ), F ( 13 ), and G ( 14 ) ( Figure 3 ) [ 33 ]. The producing organisms are commonly fungi, such as Hamigera avellanea [ 34 ], Acremonium [ 35 ] or Penicillium [ 36 ] species. Of particular interest is the high degree of D-amino acid incorporation (D-Ala, D-Aba, D-allo-Ile, and D-Val) within the macrocyclic scaffold. These natural products also contain non-proteinogenic anthranilic acid (Ant) and L-pipecolinic acid (Pip) residues, suggesting that a non-ribosomal biosynthetic pathway may have been used by the producing organisms. The enhanced bioavailability of these natural products can be attributed to improved cell permeability (via passive diffusion) and higher metabolic stability due to their cyclic nature and incorporation of D-amino acids [ 33 ]. The synthesis of 11 – 14 was achieved via the initial construction of linear hexapeptides by SPPS using a trityl alcohol SynPhase Lantern solid support. A representative synthesis for the construction of cyclohexapeptide 11 is shown in Scheme 4 . The SPPS started by attaching Fmoc-D-Ala-OH for 11 – 13 or Fmoc-D-Aba-OH for 14 , as this allowed the final solution-phase HBTU-mediated macrocyclization step to proceed with the least amount of steric hindrance [ 33 ]. The N -methyl-Leu, L-pipecolinic acid, and anthranilic acid functionality seen in 11 - 14 was incorporated into the peptide sequence employing Fmoc-L-MeLeu-OH, Fmoc-L-Pip-OH or Fmoc-Ant-OH during SPPS, respectively. When coupling to the weakly nucleophilic amino function of the N-terminal anthranilic acid residue, the Fmoc-amino acid chloride of D-allo-Ile or D-Val was generated in situ using triphosgene prior to coupling [ 33 ]. The linear hexapeptides were cleaved from the resin using 30% hexafluoroisopropanol (HFIP) in dichloromethane (DCM) and cyclized in solution using 1-[bis(dimethylamino)methylene]-1 H -1,2,3-triazolo[4,5- b ]pyridinium 3-oxide hexafluorophosphate (HATU) and N , N -diisopropylethylamine (DIPEA) in DCM at room temperature for 3 h to afford 11 – 14 . The natural products 11 – 14 were tested in a fourth-instar larvae assay and were all found to have paralytic activity against silkworm larvae. The stereochemistry of the D-Ala side chain was found to be crucial for paralytic activity, as side-chain epimers of 11 and 12 having L-Ala in place of D-Ala showed little activity.

Structures of naturally occurring cyclohexapeptides 11 – 14 .
In 2014, Peña et al. reported the synthesis and antimalarial and antitrypanosomal activity of seven novel cyclohexapeptides 15 – 21 with 20 and 21 incorporating interesting thiazole functionality [ 37 ]. The study was prompted by earlier reports of cyanobacterium Microcystis aeruginosa PCC 7806 natural products, aerucyclamides A–D ( Figure 4 ), which displayed promising micromolar IC 50 (50% of maximal inhibitory concentration) values against a K1 chloroquine-resistant strain of Plasmodium falciparum [ 38 , 39 ]. The SPPS of linear hexapeptide precursors was conducted using 2-CTC resin and standard Fmoc chemistry ( Scheme 5 ). The first Fmoc-amino acid was loaded onto 2-CTC resin in DCM in the presence of DIPEA, followed by the capping of unreacted resin sites with methanol. For the synthesis of 20 and 21 , the 2-CTC resin was first loaded with an Fmoc-protected thiazole residue (Fmoc-Thz-OH) using analogous conditions. Subsequent amino acids were installed via iterative deprotection (20% piperidine in DMF) and coupling ( N,N' -diisopropylcarbodiimide (DIC) and 3-hydroxytriazolo[4,5- b ]pyridine (HOAt) in DMF) steps. After cleavage of linear hexapeptides from the resin using 1% TFA in DCM, macrocyclizations were performed using HBTU, DIPEA, and catalytic 4-dimethylaminopyridine (DMAP) in DCM under dilute conditions (1–5 mM). The coupling site for macrocyclization was chosen to occur at N -terminal glycine for 15 , C -terminal glycine for 17 – 19 , or C -terminal thiazole for 20 – 21 , as this provided the least degree of steric hindrance, resulting in favorable yields [ 37 ]. Moreover, cyclization of linear precursors to afford 17 – 21 took place at a C -terminal glycine or thiazole to prevent epimerization.

Structures of aerucyclamides A–D.
Cyclohexapeptides 15 – 21 were screened with P. falciparum K1 and infective T. b. brucei assays. Of particular interest was the promising antimalarial activity shown by 17 , 20 , and 21 against P. falciparum K1, with EC 50 (50% of maximal effective concentration) values of 0.19, 0.19, and 0.41 µM, respectively, and no observed cell cytotoxicity against murine macrophages [ 37 ]. Also noteworthy was the antitrypanosomal activity of 15 and 19 – 21 against T. b. brucei , with EC 50 values of 1.06, 2.1, 3.0, and 2.8 µM, respectively [ 37 ].
In 2015, Wong et al. reported the total synthesis of dichotomin A ( 22 ) ( Figure 5 ) from linear peptide precursors 23 and 24 that contain penicillamine-derived pseudoproline residue ( Scheme 6 ) [ 8 ]. The utilization of a pseudoproline residue in place of the proteinogenic Val residue during the synthesis of dichotomin A was a protecting group stratergy and a way to improve head-to-tail cyclization of linear peptide precursors because of the ability of pseudoproline to induce a turn or “kink” in the peptide backbone as well as to aid peptide solubility [ 8 ]. The pseudoproline residue was deprotected and converted into the desired Val residue post cyclization to afford dichotomin A ( 22 ). Dichotomin A was prepared from two different disconnection sites within the macrocyclic ring ( Scheme 6 ). The first linear peptide precursor 23 contained a non-epimerizable C -terminal glycine and an N -terminal O -tert-butyl protected threonine residue. The second peptide precursor 24 contained an epimerizable N -terminal leucine and a C -terminal phenylalanine, creating a more sterically hindered cyclization site and providing a way to test the robustness of this methodology [ 8 ]. Linear peptides 23 and 24 containing pseudoproline residues were synthesized on 2-CTC resin using standard Fmoc-strategy SPPS with HBTU as the coupling reagent and 10% piperidine in DMF for Fmoc deprotection. Cleavage from resin was done using HFIP in DCM while keeping the side-chain protecting groups intact. The conformations of 23 and 24 were established using 1 H nuclear magnetic resonance (NMR) spectroscopy and rotating-frame Overhauser effect spectroscopy (ROESY) experiments, which also confirmed a single set of resonance structures for each peptide. Macrocycle 25 was obtained from linear peptides 23 or 24 containing a pseudoproline residue in less than 3 h and in good to excellent yields (78–88%) using 4-(4,6-dimethoxy-1,3,5-triazin-2-yl)-4-methylmorpholinium tetrafluoroborate (DMTMM·BF 4 ). In contrast, cyclization of analogous linear peptide precursors containing a native Val residue had much slower reaction kinetics, requiring 3 days for reaction completion and with lower yields (33–36%). The pseudoproline and O -tert-butyl-Thr residues in 25 were simultaneously deprotected using trifluoromethanesulfonic acid (TFMSA)/water (2:1, v / v ) to give 26 followed by desulfurization using NiCl 2 and NaBH 4 in MeOH to afford dichotomin A ( 22 ) in 24% yield.

Structure of dichotomin A ( 22 ).
In 2015, Prompanya et al. isolated a cyclohexapeptide from a culture of the marine sponge-associated fungus Aspergillus similanensis KUFA 0013 and named it similanamide ( 27 ) ( Figure 6 ) [ 40 ]. Masuda et al. undertook the total synthesis of similanamide ( 27 ) in 2015 [ 41 ] but noticed discrepancies when comparing the 1 H and 13 C-NMR data of their synthesized similanamide ( 27 ) with reported data [ 40 ]. The reported data for similanamide ( 27 ) did, however, agree favorably with a previously synthesized diastereomer 28 [ 41 ]. This led to the structural revision of similanamide ( 27 ) to the structurally similar diastereomer 28 in 2015 [ 41 ].

Structures of cyclohexapeptides 27 and 28 .
In 2016, Amso et al. reported the synthesis of cyclohexapeptide dianthin G (cyclo-Pro-Leu-Thr-Leu-Phe-Gly, 29 ) as well as nine of its analogues, including N α -methylated derivatives ( 31 – 35 ) and conformationally constrained cyclic “dicarba” bridged analogues (e.g., 30 ) ( Figure 7 ) [ 42 , 43 ]. The in vitro osteoblast proliferation activity of the synthesized compounds was determined. Dianthin G ( 29 ) and its N α -methylated derivatives ( 31 – 35 ) were synthesized by SPPS followed by cleavage and solution-phase head-to-tail macrolactamization.

Structures of dianthin G ( 29 ), “dicarba” analogue 30 , and N -methyl analogues 31 – 35 .
The SPPS of native cyclohexapeptide dianthin G ( 29 ) was reported previously [ 43 ] but was later adapted for the synthesis of methylated analogues 31 – 34 ( Scheme 7 ). The N α -methylated derivatives 31 – 34 were synthesized from aminomethyl polystyrene resin and the 3-(4-hydroxymethylphenoxy)propionic acid) (HMPP) linker; however, the synthesis of 35 required the use of a more hindered 2-CTC resin to prevent the formation of undesired diketopiperazine by-products [ 42 ]. A representative synthesis of cyclohexapeptide 31 is shown in Scheme 7 . The aminomethyl polystyrene resin was first loaded with Fmoc-Gly-HMPP-OH to form 36 . The solid-supported linear pentapeptide 37 was constructed on-resin using classical SPPS. The terminal amino function was activated by reacting with 2-nitrobenzenesulfonyl chloride to form 38 , followed by methylation and deprotection to afford 39 . After attaching the final proline residue, hexapeptide 40 was cleaved from the resin to form 41 , which was cyclized using HBTU-mediated solution-phase cyclization to afford 31 .
Cyclic dicarba analogues contained a non-native dicarba bridge, for example, 30 , and were synthesized by on-resin Grubbs’ ring-closing metathesis (RCM) and then cleaved from resin to form 30 as an inseparable mixture of cis and trans isomers [ 42 ]. An N α -methyl amide bond scan of the synthesized dianthin G, which was done to investigate the effect that altering amide bonds had on osteoblast proliferation, found that all native peptide bonds contained in the primary sequence of dianthin G ( 29 ) were of importance for osteoblast proliferation activity. From in vitro studies, native dianthin G ( 29 ) and a dicarba bridged analogue (at 10 −8 M) were found to increase the numbers of human osteoblasts without having a significant effect on osteoclast differentiation or development. An inseparable Z/E mixture of olefins in a 2:1 ratio with a β-sheet-like secondary structure similar to that of native dianthin G ( 29 ) was determined through spectroscopic analysis of the dicarba analogue. It was suggested that this secondary structure is important for the bone activity associated with dianthin peptides.
In 2017, Asfaw et al. reported the synthesis of cyclohexapeptide wollamide B ( 42 ) and 24 of its analogues [ 44 ]. The Fmoc-based SPPS of a linear hexapeptide precursor was followed by solution-phase macrocyclization and cleavage of protecting groups, as described in Scheme 8 . The first amino acid (Fmoc-Leu-OH) was loaded onto 2-CTC resin by using DIPEA/DCM followed by capping with methanol. HATU and DIPEA in NMP were used during the coupling steps to elongate the peptides. The N -terminal Fmoc group was deprotected after each round of coupling using 20% piperidine in DMF. The resin was treated with 20% HFIP in DCM to complete cleavage of the linear hexapeptide precursors. Macrocyclization was done using HATU, hydroxybenzotriazole (HOBt), and DIPEA in DMF to produce a crude cyclic hexapeptide that was then purified via column chromatography. Lastly, the Trt and Boc side-chain protecting groups were removed using a TFA/triisopropylsilane (TIPS)/H 2 O solution (95/2.5/2.5) to afford wollamide B ( 47 ) in 91% yield after purification by silica gel chromatography.
The antimycobacterial activities as well as the in vitro drug metabolism and PK (absorption, distribution, metabolism, and excretion—ADME) profiles of 42 and structural analogues were investigated. Wollamide B ( 42 ) was found to have notable aqueous solubility, moderate affinity for plasma protein albumin, modest lipophilicity, poor passive permeability through artificial membranes, significant in vitro plasma stability, high microsomal metabolic stability, and no toxicity in HepG2 cells for concentrations of up to 50 µM. Notably, five of the synthesized wollamide B analogues ( 43 – 47 ; Figure 8 ) displayed potent antimycobacterial activity (minimum inhibitory concentration (MIC) of ≤3.1 µM) and no toxicity in HepG2 cells for concentrations of up to 100 µM. Compounds 43 and 46 also showed an optimal balance between antimycobacterial activity and PK properties. Overall, the synthesized wollamides displayed notable plasma stability and aqueous solubility with moderate to low metabolic stability.

Structures of cyclohexapeptides 43 – 47 .
In 2017, our group reported the total synthesis of the natural products wollamides A ( 48 ) and B ( 42 ) and desotamide B ( 49 ) ( Figure 9 ) using SPPS of linear hexapeptide precursors followed by cleavage and solution-phase cyclization [ 45 ]. A representative synthesis that was used to access wollamide B ( 42 ) is highlighted in Scheme 9 . The first Fmoc amino acid (Fmoc-Asn(Trt)-OH) was loaded onto 2-CTC resin with the aid of DIPEA in DCM. The hexapeptide sequence corresponding to wollamide B (D-Orn-Trp-Leu-D-Leu-Val-Asn) was synthesized on-resin via repeated coupling and deprotection steps, as shown in Scheme 9 . Cleavage of the linear hexapeptide from the resin using the mild cleavage reagent HFIP resulted in 50 having side-chain residue protecting groups still attached. The solution-phase macrocyclization was done using HBTU and DIPEA in DMF, providing the protected cyclohexapeptide 51 . Wollamide B ( 42 ) was obtained after removal of the side-chain protecting groups of 51 and final purification using flash column chromatography on silica gel. An optimization study investigated the efficiency of the macrocyclization step occurring at each of the six peptide bond sites. This determined that macrocyclization of the linear hexapeptide precursor between terminal L-Leu and D-Leu residues provided the most efficient macrocyclization without any detectable epimerization [ 45 ].

Wollamides A ( 48 ), and B ( 42 ) and desotamide B ( 49 ).
In 2018, our group reported the antitubercular and antibacterial activities of wollamides A ( 48 ) and B ( 42 ) and desotamide B ( 49 ) as well as structural analogues thereof [ 46 ]. The optimized synthetic route to access these compounds was described previously [ 45 ]. The 27 peptides’ library was screened against a panel of Gram-positive and -negative bacterial pathogens, which discovered that wollamides A ( 48 ) and B ( 42 ) and the position-II L-Ile analogue 52 exhibited promising antibacterial activity against Mycobacterium tuberculosis , with MIC values of 1.56 µg/mL and favorable selectivity indexes (SIs) of >100 ( Figure 10 ) [ 46 ]. The cyclic nature of the wollamide cyclohexapeptides was crucial for their antibacterial activity, as the corresponding linear hexapeptide precursor did not show activity even at the highest concentration tested (200 µg/mL) [ 46 ]. The residues at positions II and VI were found to have a major impact on the activity and selectivity, and hence further structure–activity relationship (SAR) studies that focus on optimizing these residues are highly warranted.

Synthesis and structure–activity relationship (SAR) studies of cyclohexapeptides 48 , 52 , and 42 .
3. Solid-Phase Cyclohexapeptide Synthesis Using On-Resin Cyclization
In 2015, Lewis et al. synthesized two cyclic hexapeptides, 53 and 54 ( Figure 11 ), in order to probe their cell permeability and PK properties, including oral bioavailability [ 23 ]. They demonstrated that the tri- N -methylated peptide 54 experienced increased cell permeability, higher plasma protein binding, and decreased clearance rates compared to the non-methylated variant 53 [ 23 ]. This resulted in a favorable bioavailability of 30% for the tri- N -methylated cyclohexapeptide 54 . The cyclic hexapeptides cyclo-Leu-D-Leu–Leu-Leu-D-Pro-Tyr ( 53 ) and cyclo-Leu-NMe-D-Leu-NMe-Leu-Leu-D-Pro-NMe-Tyr ( 54 ) were synthesized using traditional SPPS starting from a trityl resin preloaded with allyl ester Fmoc-Tyr, which was resin-linked via the Tyr side-chain hydroxyl group ( Scheme 10 ). The resin-linked linear hexapeptide 55 was constructed using a sequence of coupling and deprotection steps. The allyl and N -terminal Fmoc groups were removed using Pd(PPh 3 ) 4 and 10% piperidine/THF, respectively, to give 56 , which was cyclized on-resin using HATU/HOBt to give the resin-attached cyclohexapeptide 57 . Cleavage of 57 from the resin using TFA provided 53 . Cyclohexapeptide 54 was synthesized from 57 via the global and selective introduction of N -methyl groups using a LiO t Bu base, followed by MeI to provide resin-attached trimethylated derivative 58 ( Scheme 10 ). Cleavage of 58 from the resin afforded 54 . An alternative synthesis of 54 was also investigated and involved a stepwise construction of the trimethylated linear hexapeptide using traditional SPPS followed by cleavage and solution-phase macrocyclization. Although two routes for the synthesis of 54 were investigated, the first route involving global N -methylation of the resin-bound cyclohexapeptide 57 followed by cleavage was more efficient and provided crude 54 in higher purity before final purification.

Structures of cyclohexapeptides 53 and 54 .
In 2015, Wodtke et al. reported the design and synthesis of cyclohexapeptide analogues 59 – 61 containing an amino acid sequence inspired by the Asp-Glu-Lys-Ser (DEKS) motif of N -terminal telopeptide of type I collagen [ 47 ]. It was suggested that the DEKS motif adopts a β-turn conformation upon docking with its receptor; in addition, the β-turn conformation of the DEKS motif can be stabilized by incorporating it into a cyclized peptide backbone along with strategic introduction of D-Pro and Lys(4-fluorobenzoyl) residues [ 47 ]. The presence of the 4-fluorobenzoyl group in the second Lys of 59 – 61 could have an application as a radiolabeling site, if required, by incorporating fluorine-18 [ 47 ]. The cyclohexapeptide 59 was resistant to bovine trypsin-mediated degradation over 30 min, during which time its corresponding linear analogue was completely digested. This demonstrated how cyclized peptides offer enhanced metabolic stability over their linear counterparts [ 47 ].
The synthesis of cyclohexapeptide 59 – 61 was carried out according to Scheme 11 . Firstly, Fmoc-Lys-OAll or Fmoc-Hnl-OAll was loaded onto 2-CTC resin (attached via side chain) in THF and DIPEA followed by capping unreacted resin sites with methanol. The subsequent Fmoc-amino acids were added to the sequence using standard microwave-assisted SPPS, 20% piperidine, and 0.1M HOBt in DMF for the Fmoc deprotection steps and Fmoc-amino acid, HBTU, and DIPEA in NMP for the coupling steps. The resin-bound linear hexapeptide was cyclized on-resin using HATU and DIPEA in DMF after deprotection of the C -terminal allyl and N -terminal Fmoc groups with Pd(PPh 3 ) 4 and 20% piperidine in DMF, respectively. The 4-fluorobenzoyl moiety was installed onto the lysine side chain using 4-fluorobenzoyl chloride after firstly removing the 1-(4,4-dimethyl-2,6-dioxocyclohex-1-ylidene)-3-ethyl (Dde) protecting group with hydrazine. The cyclohexapeptide was cleaved from the resin with simultaneous removal of the side-chain protecting groups using a cleavage cocktail solution comprising TFA/triethylsilane (TES) (95/2.5/2.5) to give 59 – 60 in good yields. Compound 61 was synthesized by cleaving the cyclohexapeptide 62 from the resin using mild acid conditions for TFA/TES/DCM (1:5:94) to give 62 with side-chain protecting groups attached. Finally, Dess–Martin periodinane oxidation of 62 followed by removal of the side-chain protecting groups provided 61 in good yield.
In 2016, Jikyo reported the synthesis of cyclic hexapeptides 65a – d using an on-resin head-to-tail cyclization strategy on trichloroacetimidate Wang resin ( Scheme 12 ) [ 48 ]. The D-Ser side chain of Fmoc-D-Ser-OAll was anchored to the trichloroacetimidate Wang resin using BF 3 ·OEt 2 in dry TFA. Iterative coupling and deprotection steps using BOP/HOBt/DIPEA and 20% piperidine/DMF, respectively, for five cycles constructed resin-bound linear hexapeptide intermediates having Fmoc/Boc/OAll protecting groups intact. Pd(PPh 3 ) 4 in CHCl 3 /AcOH/ N -methylmorpholine (NMM) was used under anhydrous conditions to complete C- terminal O -allyl deprotection before the addition of 20% piperidine in DMF to remove N -terminal Fmoc, which afforded anchored linear peptides 63a – d having deprotected C - and N- terminals. On-resin cyclization to afford 64a – d was achieved with PyBOP/HOBt/DIPEA as the coupling agent, and cleavage from the resin using 95% TFA resulted in cyclohexapeptides 65a – d in 13–63% yields. Interestingly, on-resin cyclization of 64c , having two D-Pro residues in the chain, gave the highest yield of cyclized product 65c with a yield of 63%.
In 2018, Chen et al. reported the synthesis and antibacterial evaluation of cyclohexapeptides desotamide B ( 49 ) and wollamide B ( 42 ) in addition to a series of their structural analogues by utilizing an on-resin head-to-tail cyclization strategy ( Scheme 13 ) [ 49 ]. Fmoc-Asp-OAll was first anchored to the Rink Amide AM resin through the use of the coupling reagent O -(1H-6-chlorobenzotriazole-1-yl)-1,1,3,3-tetramethyluronium hexafluorophosphate (HCTU)/DIPEA to form the resin-bond amino acid 66 . SPPS was used to form the linear peptide 67 , which contained different Fmoc protected amino acids. The allyl group was uncapped with Pd(PPh 3 ) 4 and phenylsilane. The N -terminal amino group was then released by using a treatment of 20% piperidine/DMF to form the linear peptide precursor 68 . The on-resin cyclization step included treatment with (7-azabenzotriazol-1-yloxy)tripyrrolidinophosphonium hexafluorophosphate (PyAOP)/HOAt/NMM in NMP for 12 h to produce the protected cyclohexapeptide 69 on-resin. The target cyclohexapeptides were produced after final cleavage and global deprotection with TFA/phenol/water/TIPS (88:5:5:2, v / v / v / v ).
Cyclohexapeptide 71 ( Figure 12 ) showed inhibitory antibacterial activity for methicillin-resistant S. aureus (MRSA)2 (MIC = 128 µg/mL), MRSA4 (MIC = 32 µg/mL), MRSA5 (MIC = 64 µg/mL), and S. aureus (MIC = 64 µg/mL), which was about 2-fold increase in activity compared to desotamide B ( 49 ). It was therefore suggested that replacing Val with Ile improves bioactivity. Compounds 70 and 72 showed a loss of activity (MIC > 128 µg/mL), suggesting that D-Leu is necessary for antibacterial activity. The loss of activity of 73 suggested that D-Orn may be required for the antibacterial activity of wollamide B ( 42 ). However, it was also suggested that D-Orn may increase cytotoxicity, as wollamide B ( 42 ) showed higher cytotoxicity than 73 in MCF-7 and HepG-2 assays . Nearly all of the synthesized cyclopeptides lacked cytotoxicity (IC 50 > 100 µM) against both human tumor cells MCF-7 and HepG-2, except for wollamide B ( 42 ), which exhibited cytotoxicity against HepG-2 (IC 50 = 79.2 µM).

Structures of desotamide B ( 49 ), wollamide B ( 42 ), and cyclohexapeptide analogues 70 – 73 .
In 2018, Fagundez et al. synthesized cyclohexapeptides via Fmoc/SPPS by using 2-CTC resin followed by macrolactamization either on-resin ( 75 – 78 ) ( Figure 13 ) or in solution phase after cleavage of linear peptide precursors from resin [ 50 ]. A general method for the on-resin cyclization of cyclohexapeptides 75 – 78 is shown in Scheme 14 . The synthesis of 74 consists of the peptide sequence being synthesized on the resin in a similar fashion as is discussed above and being cleaved before undergoing macrocyclization in the solution phase. The compounds produced through on-resin cyclization allowed new derivatives to be synthesized as a result of the presence of a free carboxylic acid. Solution-phase cyclization resulted in the ability to produce more diverse cyclic peptides; however, the on-resin route was more convenient, as larger amounts of product with higher yield and acceptable purity could be produced.

Structures of cyclohexapeptides 74 – 78 .
The activity against the chloroquine-resistant K1 strain of P. falciparum for each of the synthesized cyclopeptides was determined. Two cyclopentapeptides, which were also synthesized and compared to the cyclic hexapeptides, were found to have less activity. Six of the synthesized cyclic hexapeptides exhibited sub-micromolar activity against P. falciparum K1. Hexapeptides 75 and 76 exhibited a free carboxylic group from Glu, which permitted the production of derivatives or products with more soluble salts. Cyclic hexapeptide 74 cyclo-Cys(Trt)-Gly-Thr( t Bu)-Gly-Cys(Trt)-Gly was determined to be very active as well as selective against P. falciparum (EC 50 = 28 nM). It was suggested that the biological activity of 74 was affected by substitution of the larger hydrophobic amino acids Phe, Met, and Ile contained in 17 and 19 by Gly, as well as by the retention of one Thr and two Cys.
Together, in the on-resin head-to-tail cyclization strategy, the peptide stays anchored to the resin throughout the final cyclization step, whereas in classical methods reported in previous studies, cleavage of the on-resin linear precursors occurred before cyclization in the solution phase. It was suggested that the on-resin head-to-tail cyclization strategy could improve the process of synthesizing cyclic peptides as well as reduce the quantity of product lost during synthesis.
4. Conclusions
In conclusion, cyclohexapeptides represent an important class of natural products and medicinal molecules. Compared to their linear hexapeptide counterparts, macrocyclic hexapeptides often possess improved cell permeability, higher metabolic stability, and enhanced bioavailability as a result of their cyclic nature and incorporation of unnatural amino acids. SPPS has enabled the rapid, efficient synthesis of hexapeptide and peptidomimetic libraries for subsequent biological evaluation and high-throughput screening. In this review, we summarize recent methods used in the successful construction of cyclohexapeptides using SPPS followed by on-resin or solution-phase cyclization. We also highlight recent advances in solid-phase hexapeptide synthesis and their applications, ranging from natural product total synthesis, synthetic methodology development, medicinal activities, and drug development, to analyses of conformational and physiochemical properties.
Head-to-tail cyclization of linear peptides can be accomplished by either on-resin or solution-phase macrolactamization once they are released from the resin. The advantage of on-resin cyclization is that the formation of linear and/or cyclic oligomeric side products can be minimized. In addition, cyclization on-resin can shorten the synthetic route and minimize purification steps, although the reaction progress is more challenging to monitor. In the case of solution-phase cyclization, performing the reaction under dilute conditions (1–5 mM) can favor cyclization over dimer/oligomer formation. In some examples, adding the linear peptide precursor in a dropwise fashion to maintain high dilution was used successfully. Utilizing a turn-inducing pseudoproline residue can greatly enhance cyclization rates, although it is required to be deprotected post cyclization, with additional reaction step(s). On the other hand, epimerization of the C -terminal amino acid during cyclization can be attenuated by employing efficient coupling reagents, such as the azabenzotriazole class. Furthermore, epimerization can be completely avoided by choosing to cyclize at a non-epimerizable residue such as C -terminal Gly (when applicable) or by employing the method of O - N -acyl migration on cyclodepsipeptides precursors. Among various solid supports, 2-CTC resin remains one of the most popular resins in SPPS because of its mild acidolytic cleavage conditions as well as steric effect, preventing diketopiperazine formation.

Solid-phase synthesis of nocardiamides A ( 1 ) and B ( 2 ) on 2-chlorotrityl chloride (2-CTC) resin. Reagents and conditions: (a) Fmoc-Tyr( t Bu)-OH; (b) HBTU, DIPEA, and DMF (r.t., 1.5 h); (c) MeOH (r.t., 0.5 h); (d) 20% piperidine/DMF (r.t., 20 min); (e) Fmoc-AA-OH, HBTU, DIPEA, and DMF (r.t., 0.5 h); (f) TFA/thioanisole/PhOH/1,2-ethanedithiol/H 2 O (82.5/5/5/2.5/5) (r.t.; 31.1% yield for 3 and 47.8% for 4 after RP-HPLC purification); (g) HBTU, DIPEA, and DMF (r.t.; 7.2% yield for nocardiamide A ( 1 ) and 10.7% for nocardiamide B ( 2 ) after RP-HPLC purification).

Solid-phase synthesis of cyclohexapeptide 7 on 2-chlorotrityl chloride (2-CTC) resin. Reagents and conditions: (a) 1. Fmoc-Gly-OH, DIPEA, and DCM/DMF (4/1) (r.t., 16 h); 2. MeOH (r.t., 20 min); (b) two treatments with 20% piperidine/DMF (r.t., 5 min); (c) Fmoc-Tyr( t Bu)-OH, HATU (0.5M), DIPEA, and DMF (70 °C, 5 min, microwave heating); (d) Fmoc-Gly-OH, HATU (0.5M), DIPEA, and DMF (70 °C, 5 min, microwave heating); (e) three treatments with 5% TFA in CH 3 CN/H 2 O (4:1) (r.t., 20 min, 85%); (f) PyBOP and DMF (r.t., 1 h; quantitative yield after purification by flash chromatography (silica gel)).

Solid-phase synthesis of 9 and 10 on 2-chlorotrityl chloride (2-CTC) resin. Reagents and conditions: (a) Fmoc-Leu-OH, DIPEA, and DCM (r.t., overnight); (b) treated twice with 50% piperidine/DMF (r.t., 10 min); (c) Fmoc-Leu-OH, HATU, DIPEA, and DMF (r.t., 2 h); (d) TFA/TIPS/H 2 O (95/2.5/2.5) (r.t., 2 h); (e) dropwise addition of linear peptide solution in DMF to solution of PyBOP, DIPEA, and DMF (over 3 h, r.t., overnight; 17% yield for 9 and 3% for 10 after preparative RP-HPLC purification).

Solid-phase peptide synthesis of 11 on trityl alcohol lantern. Reagents and conditions: (a) AcCl and DCM (r.t., 4 h); (b) Fmoc-D-Ala-OH, DIPEA, and DCM (r.t., 12 h); (c) 20% piperidine/DMF (r.t., 1 h); (d) Fmoc-AA-OH, DIC, HOBt, and DMF (r.t., 12 h); (e) 30% hexafluoroisopropanol (HFIP)/DCM (r.t., 1 h); (f) HATU, DIPEA, and DCM (r.t., 3 h); yields (over 13 steps): 29% ( 11 ), 41% ( 12 ), 39% ( 13 ), and 29% ( 14 )).

Solid-phase peptide synthesis of 15 – 21 on 2-chlorotrityl chloride (2-CTC) resin. Reagents and conditions: (a) Fmoc-AA-OH (or Fmoc-Thz-OH in the synthesis of 20 and 21 ), DIPEA, and DCM (r.t., 1 h, capped with MeOH, r.t., 0.5 h); (b) 1. deprotection: 20% piperidine/DMF (r.t., 2 × 5 min followed by 1 × 10 min); 2. coupling: Fmoc-AA-OH, HOAt, DIC, and DMF (r.t., 2 h); 3. steps 1–2 repeated; (c) cleavage: four treatments with 1% TFA/DCM (r.t., 3 min); (d) macrocyclization: (concentration of 1–5 mM) HBTU, DIPEA, DMAP, and DCM (r.t., 3–5 days; cyclization yields: 59% ( 15 ), 55% ( 16 ), 48% ( 17 ), 66% ( 18 ), 54% ( 19 ), 83% ( 20 ), and 40% ( 21 )).

Solid-phase peptide synthesis of dichotomin A ( 22 ) on 2-chlorotrityl chloride (2-CTC) resin. Reagents and conditions: (a) 1. Fmoc-Gly-OH (for 23 ) or Fmoc-Phe-OH (for 24 ), DIPEA, and DCM; 2. DCM/MeOH/DIPEA (17:2:1); (b) 10% piperidine/DMF; (c) Fmoc-AA-OH, HBTU, DIPEA, and NMP; (d) 20% HFIP/DCM; (e) (concentration of 1 mM) DMTMM·BF 4 , DIPEA, and DMF (r.t., 3 h; yield of 25 : 84% from 23 and 87% from 24 )); (f) TFMSA/water (2:1) (r.t., 4 h, 33%); (g) NiCl 2 , NaBH 4 , and MeOH (0 °C, 0.5 h, 24%).

Representative solid-phase peptide synthesis of 31 on aminomethyl polystyrene resin. Reagents and conditions: (a) Fmoc-Gly-O-HMMP-OH, DIC, DCM, and DMF (r.t., 4 h); (b) deprotection: 20% piperidine/DMF (r.t., 2 × 5 min); (c) coupling: Fmoc-AA-OH, HATU, DIPEA, and DMF (r.t., 45 min); (d) 2-nitrobenzenesulfonyl chloride, sym-collidine, and NMP (r.t., 2 × 15 min); (e) dimethyl sulfate, DBU, and NMP (r.t., 2 × 5 min); (f) 2-mercaptoethanol, DBU, and NMP (r.t., 2 × 5 min); (g) TFA/TIPS/H 2 O (95/2.5/2.5) (r.t., 3 h); (h) HBTU, 6-Cl-HOBt, DIPEA, DCM, and DMF (r.t., 36 h; overall yields: 52% ( 29 ), 38% ( 31 ), 22% ( 32 ), 51% ( 33 ), 43% ( 34 ), 39% ( 35 )).

Solid-phase peptide synthesis of 42 on 2-chlorotrityl chloride (2-CTC) resin. Reagents and conditions: (a) Fmoc-Leu-OH, DIPEA, and DCM (r.t., 2 h); (b) 20% piperidine/DMF (r.t., 2 × 10 min); (c) Fmoc-AA-OH, HBTU, DIPEA, and NMP (r.t., 1 h); (d) 20% HFIP/DCM (r.t., 1 h); (e) (concentration of 1 mM) HATU, HOBt, DIPEA, and DMF (0 °C to r.t.; r.t., 3 days; cyclization yield: 68%); (f) TFA/TIPS/H 2 O (95/2.5/2.5) (r.t., 3 h; yield of 42 : 91%).

Solid-phase peptide synthesis of 42 on 2-chlorotrityl chloride (2-CTC) resin. Reagents and conditions: (a) Fmoc-Asn(Trt)-OH, DIPEA, and DCM (r.t., 3 h); (b) 25% 4-methylpiperidine/DMF; (c) Fmoc-Val-OH, DIC, HOBt, and DMF/DCM (1:1) (r.t., 4 h); (d) Fmoc-D-Leu-OH, DIC, HOBt, and DMF/DCM (1:1) (r.t., 4 h); (e) Fmoc-Leu-OH, DIC, HOBt, and DMF/DCM (1:1) (r.t., 4 h); (f) Fmoc-Trp(Boc)-OH, DIC, HOBt, and DMF/DCM (1:1) (r.t., 4 h); (g) Fmoc-D-Orn(Boc)-OH, DIC, HOBt, and DMF/DCM (1:1) (r.t., 4 h); (h) HFIP/DCM (1:4) (r.t., 0.5 h); (i) (concentration of 1 mM) HBTU, DIPEA, and DMF (r.t., 0.5 h; cyclization yield of 51 : 72%); ( j ) TFA/TIPS/DCM (50:5:45) (r.t., 0.5 h, 42%).

Solid-phase synthesis of 53 and 54 on trityl resin. Reagents and conditions: (a) 20% 4-methylpiperidine/DMF (r.t., 2 × 5 min); (b) Fmoc-AA-OH, PyOxim, DIPEA, and DMF/NMP (1:1) (r.t., 45 min); (c) Pd(PPh 3 ) 4 and 10% piperidine/THF (r.t., 2 h); (d) HATU, HOBt, DIPEA, and DMF/NMP (1:1) (r.t., 18 h); (e) 10% TFA/DCM (r.t., 4 × 5 min); (f) LiO t Bu and THF (r.t., 0.5 h); (g) MeI and DMSO (r.t., 0.5 h).

Solid-phase peptide synthesis of 59–62 on 2-chlorotrityl chloride (2-CTC) resin. Reagents and conditions: (a) 1. (for X=NH) Fmoc-Lys-OAll, DIPEA, and THF (r.t., 2 h); 2. DCM/MeOH/DIPEA (17:1:2) (r.t., 3 × 2 min); 1. (for X=O), Fmoc-Hln-OAll, pyridine, and DCM/DMF (1:1) (r.t., 64 h); 2. DCM/MeOH/DIPEA (17:1:2) (r.t., 3 × 2 min); (b) 1. deprotection: 20% piperidine, 0.1M HOBt, and DMF (microwave irradiation: 35 W, 75 °C, 30 s, followed by 44 W, 75 °C, 3 min); 2. coupling: Fmoc-AA-OH, HBTU, DIPEA, and DMF (microwave irradiation: 21 W, 75 °C, 5 min); 3. steps 1–2 repeated; (c) Pd(PPh 3 ) 4 , and DCM/NMM/acetic acid (8:2:1) (r.t., 4 h); (d) 20% piperidine/DMF (r.t., 2 × 8 min); (e) HATU, DIPEA, and DMF (r.t., 4 h); (f) 2% N 2 H 4 and DMF (r.t., 5–12 × 5 min); (g) 4-fluorobenzoyl chloride, NEt 3 , and DCM (r.t., 2 h); (h) TFA/TES/H 2 O (95/2.5/2.5) (r.t., 3 h); (i) TFA/TES/DCM (1:5:94) (r.t., 0.5 h); (j) Dess–Martin periodinane and DCM (r.t., 3 h); (k) TFA/DCM (9:1) (r.t., 1 h; overall yields: 80% ( 59 ), 30% ( 60 ), and 25% ( 61 )).

Solid-phase peptide synthesis (SPPS) of cyclohexapeptides 65a – d . Reagents and conditions: (a) Fmoc-D-Ser-OAll, BF 3 ·OEt 2 , and dry TFA (r.t., 1 h); (b) SPPS for five cycles: deprotection: 20% piperidine/DMF; coupling: Fmoc-AA-OH, BOP, HOBt, DIPEA, and DMF; (c) Pd(PPh 3 ) 4 and PhSiH 3 ; (d) 20% piperidine/DMF; (e) PyBOP, HOBt, DIPEA, and DMF (r.t., 17 h); (f) 95% TFA (r.t., 2 h; yields: 15% ( 65a ), 38% ( 65b ), 63% ( 65c ), and 13% ( 65d ).

Representative solid-phase peptide synthesis (SPPS) of 49 on Rink amide resin. Reagents and conditions: (a) 20% piperidine/DMF (r.t., 5 × 10 min); (b) Fmoc-Asp-OAll, HCTU, DIPEA, and DMF (r.t., 1 h); (c) SPPS: coupling: Fmoc-AA-OH, HCTU, DIPEA, and DMF (r.t., 1 h); deprotection: 20% piperidine/DMF (r.t.; 5 × 10 min); coupling/deprotection steps repeated. (d) Pd(PPh 3 ) 4 , phenylsilane, and DCM; (e) PyAOP, HOAt, NMM, and NMP (r.t., 12 h); (f) TFA/H 2 O/phenol/TIPS (88:5:5:2, v/v/v/v ) (r.t., 2 h).

Solid-phase peptide synthesis of 75 – 78 on 2-chlorotrityl chloride (2-CTC) resin. Reagents and conditions: (a) Fmoc-Glu-OAll, DIPEA, and DMF; (b) capped with MeOH; (c) deprotection: 20% piperidine/DMF; (d) coupling: Fmoc-AA-OH, HBTU, DIPEA, and DMF (r.t., 1–2 h); (e) Pd(PPh 3 ) 4 and 10% piperidine/THF (r.t., 3 h); (f) DIC, Cl-HOBt, and DMF/DCM (8:2) (r.t., overnight); (g) 1% TFA/DCM (r.t., 2–3 min; overall yield: 51% ( 76 ), 63% ( 77 ), 46% ( 78 ), and 80% ( 79 )).
Abbreviations
The following abbreviations are used in this manuscript:
ADME | Absorption, distribution, metabolism and excretion |
BOP | (Benzotriazol-1-yloxy)tris(dimethylamino)phosphonium hexafluorophosphate |
CsA | Cyclosporin A |
2-CTC | 2-Chlorotrityl chloride |
DBU | 1,8-Diazabicyclo[5.4.0]undec-7-ene |
DCM | Dichloromethane |
Dde | 1-(4,4-Dimethyl-2,6-dioxocyclohex-1-ylidene)-3-ethyl |
DIC | -Diisopropylcarbodiimide |
DIPEA | -Diisopropylethylamine |
DMAP | 4-Dimethylaminopyridine |
DMF | Dimethylformamide |
DMTMM.BF | 4-(4,6-Dimethoxy-1,3,5-triazin-2-yl)-4-methylmorpholinium tetrafluoroborate |
EC | 50% of Maximal effective concentration |
Fmoc | 9-Fluorenylmethoxycarbonyl |
Fmoc-AA-OH | Fmoc protected amino acid |
HATU | 1-[Bis(dimethylamino)methylene]-1 -1,2,3-triazolo[4,5- ]pyridinium 3-oxide hexafluorophosphate |
HBTU | -Tetramethyl- (1 -benzotriazol-1-yl)uronium hexafluorophosphate |
HCTU | -(1 -6-Chlorobenzotriazole-1-yl)-1,1,3,3-tetramethyluronium hexafluorophosphate |
HFIP | 1,1,1,3,3,3-Hexafluoro-2-propanol |
HMPP | 3-(4-Hydroxymethylphenoxy)propionic acid |
HOAt | 3-Hydroxytriazolo[4,5- ]pyridine |
HOBt | Hydroxybenzotriazole |
IC | 50% of Maximal inhibitory concentration |
MIC | Minimum inhibitory concentration |
MRSA | Methicillin-resistant |
NMM | -Methylmorpholine |
NMP | -Methyl-2-pyrrolidone |
NMR | Nuclear magnetic resonance |
PK | Pharmacokinetic |
PyAOP | (7-Azabenzotriazol-1-yloxy)tripyrrolidinophosphonium hexafluorophosphate |
PyBOP | (Benzotriazol-1-yloxy)tripyrrolidinophosphonium hexafluorophosphate |
PyOxim | [Ethyl cyano(hydroxyimino)acetato- ]tri-1-pyrrolidinylphosphonium hexafluorophosphate |
RP-HPLC | Reverse phase-high-performance liquid chromatography |
r.t. | Room temperature |
SAR | Structure-activity relationship |
SI | Selectivity index |
SPPS | Solid-phase peptide synthesis |
TES | Triethylsilane |
TFA | Trifluoroacetic acid |
TFMSA | Trifluoromethanesulfonic acid |
THF | Tetrahydrofuran |
TIPS | Triisopropylsilane |
WA | Wollamide A |
WB | Wollamide B |
This work was partly supported by the National Institute of General Medical Sciences of the National Institutes of Health under Award No. P20GM103466. A.F. was supported by the National Institute of General Medical Sciences of the National Institutes of Health under Award No. R25GM113747. The content is solely the responsibility of the authors and does not necessarily represent the official views of the National Institutes of Health.
Conflicts of Interest
The authors declare no conflict of interest.
Recent Reports of Solid-Phase Cyclohexapeptide Synthesis and Applications
Total Page: 16
File Type: pdf , Size: 1020Kb
- Abstract and Figures
- Public Full-text
- Peptoid Residues Make Diverse, Hyperstable Collagen Triple Helices Peptoid Residues Make Diverse, Hyperstable Collagen Triple Helices Julian L. Kessler1, Grace Kang1, Zhao Qin2, Helen Kang1, Frank G. Whitby3, Thomas E. Cheatham III4, Christopher P. Hill3, Yang Li1,*, and S. Michael Yu1,5 1Department of Biomedical Engineering, University of Utah, Salt Lake City, Utah 84112, USA 2Department of Civil & Environmental Engineering, Collagen of Engineering & Computer Science, Syracuse University, Syracuse, New York 13244, USA 3Department of Biochemistry, University of Utah School of Medicine, Salt Lake City, UT 84112, USA 4Department of Medicinal Chemistry, College of Pharmacy, L. S. Skaggs Pharmacy Research Institute, University of Utah, Salt Lake City, Utah 84112, USA 5Department of Pharmaceutics and Pharmaceutical Chemistry, University of Utah, Salt Lake City, Utah 84112, USA *Corresponding Author: Yang Li ( [email protected] ) Abstract The triple-helical structure of collagen, responsible for collagen’s remarkable biological and mechanical properties, has inspired both basic and applied research in synthetic peptide mimetics for decades. Since non-proline amino acids weaken the triple helix, the cyclic structure of proline has been considered necessary, and functional collagen mimetic peptides (CMPs) with diverse sidechains have been difficult to produce. Here we show that N-substituted glycines (N-glys), also known as peptoid residues, exhibit a general triple-helical propensity similar to or greater than proline, allowing synthesis of thermally stable triple-helical CMPs with unprecedented sidechain diversity. We found that the N-glys stabilize the triple helix by sterically promoting the preorganization of individual CMP chains into the polyproline-II helix conformation. Our findings were supported by the crystal structures of two atomic-resolution N-gly-containing CMPs, as well as experimental and computational studies spanning more than 30 N-gly-containing peptides. [Show full text]
- A Global Review on Short Peptides: Frontiers and Perspectives † molecules Review A Global Review on Short Peptides: Frontiers and Perspectives † Vasso Apostolopoulos 1 , Joanna Bojarska 2,* , Tsun-Thai Chai 3 , Sherif Elnagdy 4 , Krzysztof Kaczmarek 5 , John Matsoukas 1,6,7, Roger New 8,9, Keykavous Parang 10 , Octavio Paredes Lopez 11 , Hamideh Parhiz 12, Conrad O. Perera 13, Monica Pickholz 14,15, Milan Remko 16, Michele Saviano 17, Mariusz Skwarczynski 18, Yefeng Tang 19, Wojciech M. Wolf 2,*, Taku Yoshiya 20 , Janusz Zabrocki 5, Piotr Zielenkiewicz 21,22 , Maha AlKhazindar 4 , Vanessa Barriga 1, Konstantinos Kelaidonis 6, Elham Mousavinezhad Sarasia 9 and Istvan Toth 18,23,24 1 Institute for Health and Sport, Victoria University, Melbourne, VIC 3030, Australia; [email protected] (V.A.); [email protected] (J.M.); [email protected] (V.B.) 2 Institute of General and Ecological Chemistry, Faculty of Chemistry, Lodz University of Technology, Zeromskiego˙ 116, 90-924 Lodz, Poland 3 Department of Chemical Science, Faculty of Science, Universiti Tunku Abdul Rahman, Kampar 31900, Malaysia; [email protected] 4 Botany and Microbiology Department, Faculty of Science, Cairo University, Gamaa St., Giza 12613, Egypt; [email protected] (S.E.); [email protected] (M.A.) 5 Institute of Organic Chemistry, Faculty of Chemistry, Lodz University of Technology, Zeromskiego˙ 116, 90-924 Lodz, Poland; [email protected] (K.K.); [email protected] (J.Z.) 6 NewDrug, Patras Science Park, 26500 Patras, Greece; [email protected] 7 Department of Physiology and Pharmacology, [Show full text]
- (12) Patent Application Publication (10) Pub. No.: US 2011/0082079 A1 Spetzler Et Al US 2011 0082079A1 (19) United States (12) Patent Application Publication (10) Pub. No.: US 2011/0082079 A1 Spetzler et al. (43) Pub. Date: Apr. 7, 2011 (54) GLUCAGON-LIKE PEPTDE-1 DERVATIVES Publication Classification AND THEIR PHARMACEUTICAL USE (51) Int. Cl. A638/22 (2006.01) (75) Inventors: Jane Spetzler, Bronsho (DK); C07K I4/575 (2006.01) Lauge Schäffer, Lyngby (DK); A6IP3/10 (2006.01) Jesper Lau, Farum (DK); Thomas A6IP 9/12 (2006.01) Kruse, Herlev (DK); Patrick A6IP3/04 (2006.01) William Garibay, Holte (DK); A6IP 9/10 (2006.01) Steffen Runge, Frederiksberg A6IPI/00 (2006.01) (DK); Henning Thogersen, Farum A6IPL/04 (2006.01) (DK); Ingrid Petersson, Frederiksberg (DK) (52) U.S. Cl. .......................... 514/7.2:530/399; 514/11.7 (57) ABSTRACT (73) Assignee: Novo Nordisk A/S, Bagsvard (DK) The invention relates to protracted Glucagon-Like Peptide-1 Appl. No.: (GLP-1) derivatives and therapeutic uses thereof. The GLP-1 (21) 12/676,451 derivative of the invention comprises a modified GLP-1 (7- PCT Fled: 37) sequence having a total of 2-12 amino acid modifications, (22) Sep. 5, 2008 including Glu22 and Arg26, and being derivatised with an PCT NO.: PCT/EP2008/061755 albumin binding residue or pegylated in position 18, 20, 23. (86) 30, 31, 34, 36, 37, or 39. These compounds are useful in the S371 (c)(1), treatment or prevention of diabetes type 2 and related dis (2), (4) Date: Oct. 13, 2010 eases. The compounds are potent, stable, have long half-lives, a high affinity of binding to albumin, and/or a high affinity of (30) Foreign Application Priority Data binding to the extracellular domain of the GLP-1 receptor (GLP-1R), all of which is of potential relevance for the overall Sep. [Show full text]
- Protein Side-Chain Translocation Mutagenesis Via Incorporation of Peptoid Residues † Byoung-Chul Lee and Ronald N ARTICLES pubs.acs.org/acschemicalbiology Protein Side-Chain Translocation Mutagenesis via Incorporation of Peptoid Residues † Byoung-Chul Lee and Ronald N. Zuckermann* Biological Nanostructures Facility, The Molecular Foundry, Lawrence Berkeley National Laboratory, 1 Cyclotron Rd., Berkeley, California 94720, United States bS Supporting Information ABSTRACT: For the last few decades, chemistry has played an important role in protein engineering by providing a variety of synthetic tools such as chemoselective side-chain modifications, chemical conjugation, incorporation of non-natural amino acids, and the development of protein-mimetic heteropolymers. Here we study protein backbone engineering in order to better understand the molecular mechanism of protein function and to introduce protease stable, non-natural residues into a protein structure. Using a combination of genetic engineering and chemical synthesis, we were able to introduce peptoid residues (N-substituted glycine residues) at defined positions into bovine pancreatic ribonuclease A. This results in a side-chain translocation from the Cα carbon to the neighboring backbone nitrogen atom. To generate these peptoid substitutions, we removed the N-terminal S-peptide of the protein by proteolysis and chemically conjugated synthetic peptide-peptoid hybrids to the new N-terminus. A triple peptoid mutant containing a catalytic  4 À1 À1 His12 peptoid mutation was active with a kcat/Km value of 1.0 10 M s . This kcat/Km value is only 10-fold lower than the control wild-type conjugate and comparable in magnitude to many other natural enzymes. The peptoid mutations increased the chain flexibility at the site of peptoid substitution and at its C-terminal neighboring residue. [Show full text]
- Discrete Arginine Topologies Guide Escape of Miniature Proteins from Early Endosomes to the Cytoplasm Jacob S Yale University EliScholar – A Digital Platform for Scholarly Publishing at Yale Yale Medicine Thesis Digital Library School of Medicine 10-1-2012 Discrete arginine topologies guide escape of miniature proteins from early endosomes to the cytoplasm Jacob S. Appelbaum Yale University Follow this and additional works at: http://elischolar.library.yale.edu/ymtdl Part of the Medicine and Health Sciences Commons Recommended Citation Appelbaum, Jacob S., "Discrete arginine topologies guide escape of miniature proteins from early endosomes to the cytoplasm" (2012). Yale Medicine Thesis Digital Library. 3362. http://elischolar.library.yale.edu/ymtdl/3362 This Open Access Dissertation is brought to you for free and open access by the School of Medicine at EliScholar – A Digital Platform for Scholarly Publishing at Yale. It has been accepted for inclusion in Yale Medicine Thesis Digital Library by an authorized administrator of EliScholar – A Digital Platform for Scholarly Publishing at Yale. For more information, please contact [email protected] . Discrete arginine topologies guide escape of miniature proteins from early endosomes to the cytoplasm. A Dissertation Presented to the Faculty of the Graduate School of Yale University in Candidacy for the Degree of Doctor of Philosophy by Jacob S. Appelbaum Dissertation Director: Alanna Schepartz i October 2012 Abstract Discrete arginine topologies guide escape of miniature proteins from early endosomes to the cytoplasm. Jacob S. Appelbaum 2012 Polypeptides and peptide mimetics sample a wide chemical space with broad potential to modulate cellular function, but their application to cytoplasmic targets is limited be cause when added to cells their cytosolic concentration remains low. This limitation is due to a diffusion barrier (the plasma membrane) and absence of dedicated import ma chinery. [Show full text]
- PYY(3-36) Analogues: Structure PYY(3-36) analogues: Structure- activity relationships in energy homeostasis A thesis submitted for the degree of Doctor of Philosophy, Imperial College London 2011 Iain David Pritchard Section of Investigative Medicine Division of Diabetes, Endocrinology and Metabolism Department of Medicine Imperial College London Abstract The developed world is currently in the grip of an obesity epidemic. As a result, there is much ongoing research into the development of an effective anti-obesity agent. Peptide YY (PYY) is a 36 amino acid gastro-intestinal hormone released post- prandially by L-cells in the gastro-intestinal tract in proportion to the calorie content of a meal. The predominant form of the hormone found in circulation is the truncated PYY(3-36). Administration of PYY(3-36) at physiological doses to humans has been shown to reduce food intake. However, due to enzymatic degradation these effects are short lived, reducing the hormone’s utility as an anti-obesity pharmaceutical agent. A series of analogues of PYY(3-36) were designed either with amino acid substitutions in specific parts of the peptide sequence and/or with chemical modifications to the native sequence with the aim of increasing resistance to enzymatic activity whilst retaining or even enhancing the peptide’s bioactivity. The analogues were tested for resistance to degradation by different proteolytic enzymes in comparison to natural PYY(3-36). Their affinity to the Y2 receptor, for which PYY(3-36) is a natural agonist was then investigated. Finally, the effects of peripheral administration of selected analogues on food intake in overnight fasted mice were investigated. [Show full text]
- Peptides As Therapeutics with Enhanced Bioactivity Send Orders of Reprints at [email protected] Current Medicinal Chemistry, 2012, 19, 4451-4461 4451 Peptides As Therapeutics with Enhanced Bioactivity D. Goodwin1, P. Simerska1 and I. Toth*,1,2, 1The University of Queensland, School of Chemistry and Molecular Biosciences; 2School of Pharmacy, St. Lucia 4072, Queensland, Australia Abstract: The development of techniques for efficient peptide production renewed interest in peptides as therapeutics. Numerous modi- fications for improving stability, transport and affinity profiles now exist. Several new adjuvant and carrier systems have also been developed, enhancing the immunogenicity of peptides thus allowing their development as vaccines. This review describes the established and experimental approaches for manufacturing peptide drugs and highlights the techniques currently used for improving their drug like properties. Keywords: Peptide, drug delivery, vaccine, manufacture, bioavailability, peptide therapeutic, immunogenicity, peptide drug, peptide synthe- sis, clinical trials. INTRODUCTION side-chain reactivity, degree of modification, incorporation of un- natural components, in addition to the required purity, solubility, Natural and synthetic peptides have shown promise as pharma- stability and scale. There are two strategies for peptide production - ceutics with the potential to treat a wide variety of diseases. This chemical synthesis and biological manufacturing. potential is often overshadowed by the inability of the peptides to reach their targets in an active form in vivo. The delivery of active Chemical Peptide Synthesis peptides is challenging due to inadequate absorption through the Chemical synthesis has been used for the production of peptides mucosa and rapid breakdown by proteolytic enzymes. Peptides are in both research and industry and led to the development of the usually selective and efficacious, therefore need only be present in majority of peptide drugs [2]. [Show full text]
- Versatile Oligo(N-Substituted) Glycines: the Many Roles Of WILEY-VCH K+V Fotosatz GmbH Nielsen Beerfelden 1. UK 19.11.2003 H.J. Maier 1 1 Versatile OligoN-Substituted) Glycines: The Many Roles of Peptoids in Drug Discovery James A. Patch, Kent Kirshenbaum, Shannon L. Seurynck, Ronald N. Zuckermann, and Annelise E. Barron 1.1 Introduction Despite their wide range of important bioactivities, polypeptides are generally poor drugs. Typically, they are rapidly degraded by proteases in vivo, and are fre- quently immunogenic. This fact has stimulated widespread efforts to develop pep- tide mimics for biomedical applications, a task that presents formidable chal- lenges in molecular design. Chemists seek efficient routes to peptidomimetic compounds with enhanced pharmacological properties, which retain the activities of their biological counterparts. Since peptides play myriad roles in living sys- tems, it is likely that no individual strategy will suffice. Indeed, a wide variety of different peptidomimetic oligomer scaffolds have been explored [1]. In order to ad- dress multiple design criteria for applications ranging from medicinal chemistry to materials science, researchers have worked to identify a non-natural chemical scaffold that recapitulates the desirable attributes of polypeptides. These include good solubility in aqueous solution, access to facile sequence-specific assembly of monomers containing chemically diverse side chains, and the capacity to form stable, biomimetic folded structures. Among the first reports of chemically diverse peptide mimics were those of %N- substituted) glycine oligomers %peptoids) [2]. Sequence-specific oligopeptoids have now been studied for over a decade, and have provided illustrative examples of both the potential of peptidomimetics and the obstacles faced in translating this potential into clinically useful compounds. [Show full text]
- 1 Peptide Therapeutics Universal Free E-Book Store Donate Us, In order to keep our Service Alive, We have to pay for placing files (Abstracts, Books, Literature & Software) on File Hosting Servers, Your donations will make our process of payment a bit easier, Please use any one of the Payment Gateway for Donation. Never matter what amount you donate (10’s or 100’s or 1000’s). Universal Free E-Book Store Universal Free E-Book Store PEPTIDE CHEMISTRY AND DRUG DESIGN Universal Free E-Book Store Universal Free E-Book Store PEPTIDE CHEMISTRY AND DRUG DESIGN Edited by BEN M. DUNN Universal Free E-Book Store Copyright © 2015 by John Wiley & Sons, Inc. All rights reserved Published by John Wiley & Sons, Inc., Hoboken, New Jersey Published simultaneously in Canada No part of this publication may be reproduced, stored in a retrieval system, or transmitted in any form or by any means, electronic, mechanical, photocopying, recording, scanning, or otherwise, except as permitted under Section 107 or 108 of the 1976 United States Copyright Act, without either the prior written permission of the Publisher, or authorization through payment of the appropriate per-copy fee to the Copyright Clearance Center, Inc., 222 Rosewood Drive, Danvers, MA 01923, (978) 750-8400, fax (978) 750-4470, or on the web at www.copyright.com. Requests to the Publisher for permission should be addressed to the Permissions Department, John Wiley & Sons, Inc., 111 River Street, Hoboken, NJ 07030, (201) 748-6011, fax (201) 748-6008, or online at http://www.wiley.com/go/permissions. Limit of Liability/Disclaimer of Warranty: While the publisher and author have used their best efforts in preparing this book, they make no representations or warranties with respect to the accuracy or completeness of the contents of this book and specifically disclaim any implied warranties of merchantability or fitness for a particular purpose. [Show full text]
- Prion-Specific Peptoid Reagents Prionspezifische Peptoidreagentien Reactifs Peptoides Specifiques Des Prions (19) TZZ_¥_5B_T (11) EP 1 931 695 B1 (12) EUROPEAN PATENT SPECIFICATION (45) Date of publication and mention (51) Int Cl.: of the grant of the patent: C07K 7/06 (2006.01) C07K 7/08 (2006.01) 10.04.2013 Bulletin 2013/15 A61K 38/08 (2006.01) A61K 38/10 (2006.01) A61K 31/16 (2006.01) G01N 33/68 (2006.01) (2006.01) (2006.01) (21) Application number: 06814415.3 G01N 33/50 A61P 25/28 C07K 14/47 (2006.01) (22) Date of filing: 08.09.2006 (86) International application number: PCT/US2006/035226 (87) International publication number: WO 2007/030804 (15.03.2007 Gazette 2007/11) (54) Prion-specific peptoid reagents Prionspezifische Peptoidreagentien Reactifs peptoides specifiques des prions (84) Designated Contracting States: (74) Representative: Marshall, Cameron John et al AT BE BG CH CY CZ DE DK EE ES FI FR GB GR Carpmaels & Ransford HU IE IS IT LI LT LU LV MC NL PL PT RO SE SI One Southampton Row SK TR London WC1B 5HA (GB) (30) Priority: 09.09.2005 US 715761 P 14.10.2005 US 726686 P (56) References cited: 13.01.2006 US 758934 P WO-A1-94/06451 WO-A2-02/052046 WO-A2-2005/016127 WO-A2-2005/060697 (43) Date of publication of application: US-A- 5 965 695 US-A1- 2003 040 468 18.06.2008 Bulletin 2008/25 • HAYNES, RUSSELL D. ET AL: "Comblike, (60) Divisional application: Monodisperse Polypeptoid Drag-Tags for DNA 11171585.0 / 2 383 281 Separations by End-Labeled Free-Solution Electrophoresis (ELFSE)" BIOCONJUGATE (73) Proprietor: Novartis AG CHEMISTRY, vol. [Show full text]
- Molecular Modeling Studies of Peptoid Polymers AIMS Materials Science, 4(5): 1029-1051. DOI: 10.3934/matersci.2017.5.1029 Received: 05 June 2017 Accepted: 25 July 2017 Published: 12 September 2017 http://www.aimspress.com/journal/Materials Review Molecular modeling studies of peptoid polymers Laura J. Weiser and Erik E. Santiso * Department of Chemical and Biomolecular Engineering, NC State University, Campus Box 7905, Raleigh, NC 27695-7905, United States * Correspondence: Email: [email protected] ; Tel: +1-919-515-2520. Abstract: Peptoids, or poly-N-substituted glycines, are synthetic polymers composed of a protein backbone with side chains attached to the nitrogen atoms rather than the α-carbons. Peptoids are biomimetic and protease resistant and have been explored for a variety of applications including pharmaceuticals and coatings. They are also foldamer-type materials that can adopt diverse structures based on the sequences of their side chains. Design of new peptoid sequences may lead to the creation of many interesting materials. Given the large number of possible peptoid side chains, computer models predicting peptoid structure-side chain relationships are desirable. In this paper, we provide a survey of computational efforts to understand and predict peptoid structures. We describe simulations at several levels of theory, along with their assumptions and results. We also discuss some challenges for future peptoid computational research. Keywords: peptoids; foldamer; molecular simulation; biomimetic Abbreviations AMBER Assisted Model Building with Energy Refinement B3LYP Becke, [Show full text]
- De Novo Structure Prediction and Experimental Characterization of Folded Peptoid Oligomers De novo structure prediction and experimental characterization of folded peptoid oligomers Glenn L. Butterfossa,1, Barney Yoob,1, Jonathan N. Jaworskic,2, Ilya Chornyd, Ken A. Dille,3, Ronald N. Zuckermannc, Richard Bonneaua, Kent Kirshenbaumb, and Vincent A. Voelzf,3 aCenter for Genomics and Systems Biology, New York University, NY 10003; bDepartment of Chemistry, New York University, NY 10003; cMolecular Foundry, Lawrence Berkeley National Laboratory, 1 Cyclotron Road, Berkeley, CA 94720; dSimprota Corporation, San Francisco, CA 94158; eLaufer Center for Physical and Quantitative Biology, Stony Brook University, Stony Brook, NY 11794-5252; and fDepartment of Chemistry, Temple University, Philadelphia, PA 19122 Contributed by Ken A. Dill, July 12, 2012 (sent for review June 12, 2012) Peptoid molecules are biomimetic oligomers that can fold into un- ique three-dimensional structures. As part of an effort to advance computational design of folded oligomers, we present blind-struc- ture predictions for three peptoid sequences using a combination of Replica Exchange Molecular Dynamics (REMD) simulation and Quantum Mechanical refinement. We correctly predicted the struc- ture of a N-aryl peptoid trimer to within 0.2 Å rmsd-backbone and a cyclic peptoid nonamer to an accuracy of 1.0 Å rmsd-backbone. Fig. 1. Peptoid vs. peptide architecture. Peptoids are oligomers of N-substi- X-ray crystallographic structures are presented for a linear N-alkyl tuted glycine units. In contrast to peptides, peptoids feature an achiral back- bone with side chain groups presented on the nitrogen atom. The relevant peptoid trimer and for the cyclic peptoid nonamer. The peptoid backbone dihedral angles are ω [Cαði − 1Þ;Cði − 1Þ;N;Cα], φ [Cði − 1Þ;N;Cα;C], macrocycle structure features a combination of cis and trans back- ψ [N; Cα;C;Nði þ 1Þ], and χ1 [Cα;N;NCα;Cβ]. [Show full text]
Information
- Author Services
Initiatives
You are accessing a machine-readable page. In order to be human-readable, please install an RSS reader.
All articles published by MDPI are made immediately available worldwide under an open access license. No special permission is required to reuse all or part of the article published by MDPI, including figures and tables. For articles published under an open access Creative Common CC BY license, any part of the article may be reused without permission provided that the original article is clearly cited. For more information, please refer to https://www.mdpi.com/openaccess .
Feature papers represent the most advanced research with significant potential for high impact in the field. A Feature Paper should be a substantial original Article that involves several techniques or approaches, provides an outlook for future research directions and describes possible research applications.
Feature papers are submitted upon individual invitation or recommendation by the scientific editors and must receive positive feedback from the reviewers.
Editor’s Choice articles are based on recommendations by the scientific editors of MDPI journals from around the world. Editors select a small number of articles recently published in the journal that they believe will be particularly interesting to readers, or important in the respective research area. The aim is to provide a snapshot of some of the most exciting work published in the various research areas of the journal.
Original Submission Date Received: .
- Active Journals
- Find a Journal
- Proceedings Series
- For Authors
- For Reviewers
- For Editors
- For Librarians
- For Publishers
- For Societies
- For Conference Organizers
- Open Access Policy
- Institutional Open Access Program
- Special Issues Guidelines
- Editorial Process
- Research and Publication Ethics
- Article Processing Charges
- Testimonials
- Preprints.org
- SciProfiles
- Encyclopedia
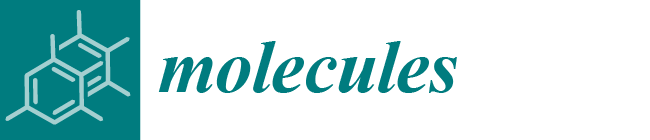
Journal Menu
- Molecules Home
- Aims & Scope
- Editorial Board
- Reviewer Board
- Topical Advisory Panel
- Instructions for Authors
- Special Issues
- Sections & Collections
- Article Processing Charge
- Indexing & Archiving
- Editor’s Choice Articles
- Most Cited & Viewed
- Journal Statistics
- Journal History
- Journal Awards
- Society Collaborations
- Conferences
- Editorial Office
Journal Browser
- arrow_forward_ios Forthcoming issue arrow_forward_ios Current issue
- Vol. 29 (2024)
- Vol. 28 (2023)
- Vol. 27 (2022)
- Vol. 26 (2021)
- Vol. 25 (2020)
- Vol. 24 (2019)
- Vol. 23 (2018)
- Vol. 22 (2017)
- Vol. 21 (2016)
- Vol. 20 (2015)
- Vol. 19 (2014)
- Vol. 18 (2013)
- Vol. 17 (2012)
- Vol. 16 (2011)
- Vol. 15 (2010)
- Vol. 14 (2009)
- Vol. 13 (2008)
- Vol. 12 (2007)
- Vol. 11 (2006)
- Vol. 10 (2005)
- Vol. 9 (2004)
- Vol. 8 (2003)
- Vol. 7 (2002)
- Vol. 6 (2001)
- Vol. 5 (2000)
- Vol. 4 (1999)
- Vol. 3 (1998)
- Vol. 2 (1997)
- Volumes not published by MDPI
- Vol. 1 (1996)
Find support for a specific problem in the support section of our website.
Please let us know what you think of our products and services.
Visit our dedicated information section to learn more about MDPI.
Solid Phase Synthesis
- Special Issue Editors
Special Issue Information
Benefits of publishing in a special issue.
- Published Papers
A special issue of Molecules (ISSN 1420-3049). This special issue belongs to the section " Organic Chemistry ".
Deadline for manuscript submissions: closed (15 May 2018) | Viewed by 39794
Share This Special Issue
Special issue editor.
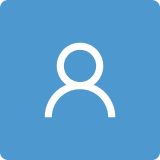
Dear colleagues,
This Special Issue of Molecules is dedicated to “Solid-Phase Synthesis”. The concept of solid-phase (SP) peptide synthesis was revealed by Bruce Merrifield in 1963 and its potential for general organic chemistry was soon recognized. However, it was not until the onset of combinatorial chemistry in early 1990s when the application of SP organic synthesis started to flourish.
Today, SP synthesis is widely used particularly in the area of drug discovery. Critical advantages of SP synthesis include very efficient isolation of intermediates by washing resin beads. Consequently, high boiling solvents, such as DMF and DMSO, can be used beneficially without the need to evaporate them.
Drug discovery efforts benefited from the time efficient synthesis of compounds decks for high throughput screening as well as dedicated libraries for hit/lead optimization. Diversity oriented synthesis (DOS) that was mainly focused on synthesis of structurally dissimilar compounds has now shifted to biology oriented synthesis (BIOS) focused on the design of structures resembling natural products, including complex chiral molecular scaffolds with three-dimensional architecture.
This Special Issue will provide an opportunity to publish research results dedicated to all aspects of SP synthesis including, but not limited to, development of new chemical transformations, new resins, linkers, methodology, catalyst development, combinatorial synthesis, solid-phase reagents, synthesis of drug-like molecules, peptides, preparation of compound decks for high throughput screening, etc.
Prof. Viktor Krchnak Guest Editor
Manuscripts should be submitted online at www.mdpi.com by registering and logging in to this website . Once you are registered, click here to go to the submission form . Manuscripts can be submitted until the deadline. All submissions that pass pre-check are peer-reviewed. Accepted papers will be published continuously in the journal (as soon as accepted) and will be listed together on the special issue website. Research articles, review articles as well as short communications are invited. For planned papers, a title and short abstract (about 100 words) can be sent to the Editorial Office for announcement on this website.
Submitted manuscripts should not have been published previously, nor be under consideration for publication elsewhere (except conference proceedings papers). All manuscripts are thoroughly refereed through a single-blind peer-review process. A guide for authors and other relevant information for submission of manuscripts is available on the Instructions for Authors page. Molecules is an international peer-reviewed open access semimonthly journal published by MDPI.
Please visit the Instructions for Authors page before submitting a manuscript. The Article Processing Charge (APC) for publication in this open access journal is 2700 CHF (Swiss Francs). Submitted papers should be well formatted and use good English. Authors may use MDPI's English editing service prior to publication or during author revisions.
- development of new chemical transformations
- methodology
- catalyst development
- combinatorial synthesis
- solid-phase reagents
- synthesis of drug-like molecules
- preparation of compound decks for high throughput screening
- Ease of navigation: Grouping papers by topic helps scholars navigate broad scope journals more efficiently.
- Greater discoverability: Special Issues support the reach and impact of scientific research. Articles in Special Issues are more discoverable and cited more frequently.
- Expansion of research network: Special Issues facilitate connections among authors, fostering scientific collaborations.
- External promotion: Articles in Special Issues are often promoted through the journal's social media, increasing their visibility.
- e-Book format: Special Issues with more than 10 articles can be published as dedicated e-books, ensuring wide and rapid dissemination.
Further information on MDPI's Special Issue polices can be found here .
Published Papers (8 papers)
Jump to: Review
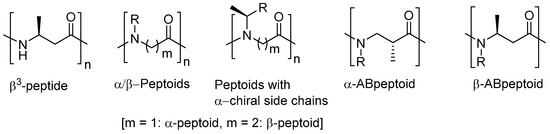
Graphical abstract
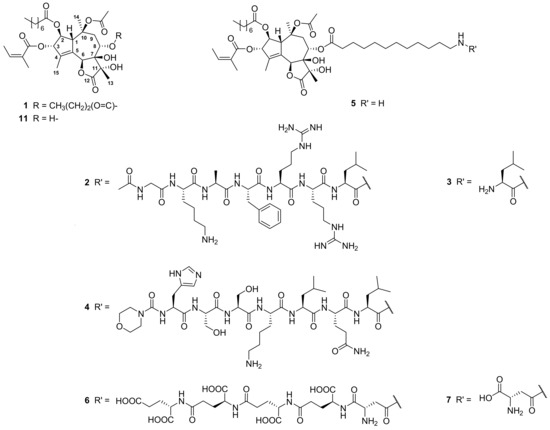
Jump to: Research
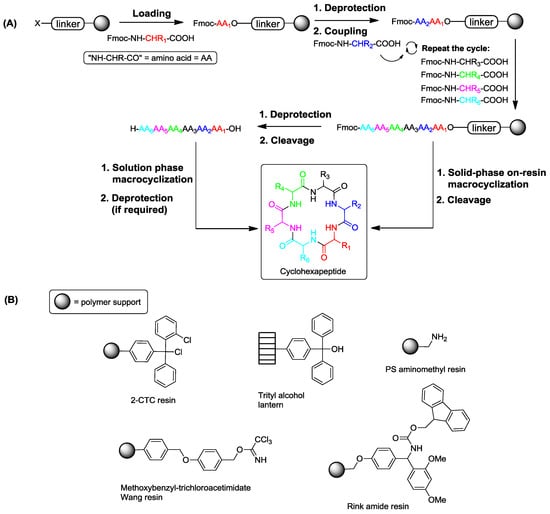
Further Information
Mdpi initiatives, follow mdpi.
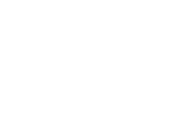
Subscribe to receive issue release notifications and newsletters from MDPI journals
- DOI: 10.1039/C4RA02458C
- Corpus ID: 93657369
Solid-phase peptide synthesis: an overview focused on the preparation of biologically relevant peptides
- Published 25 July 2014
- Chemistry, Materials Science
- RSC Advances
Figures and Tables from this paper
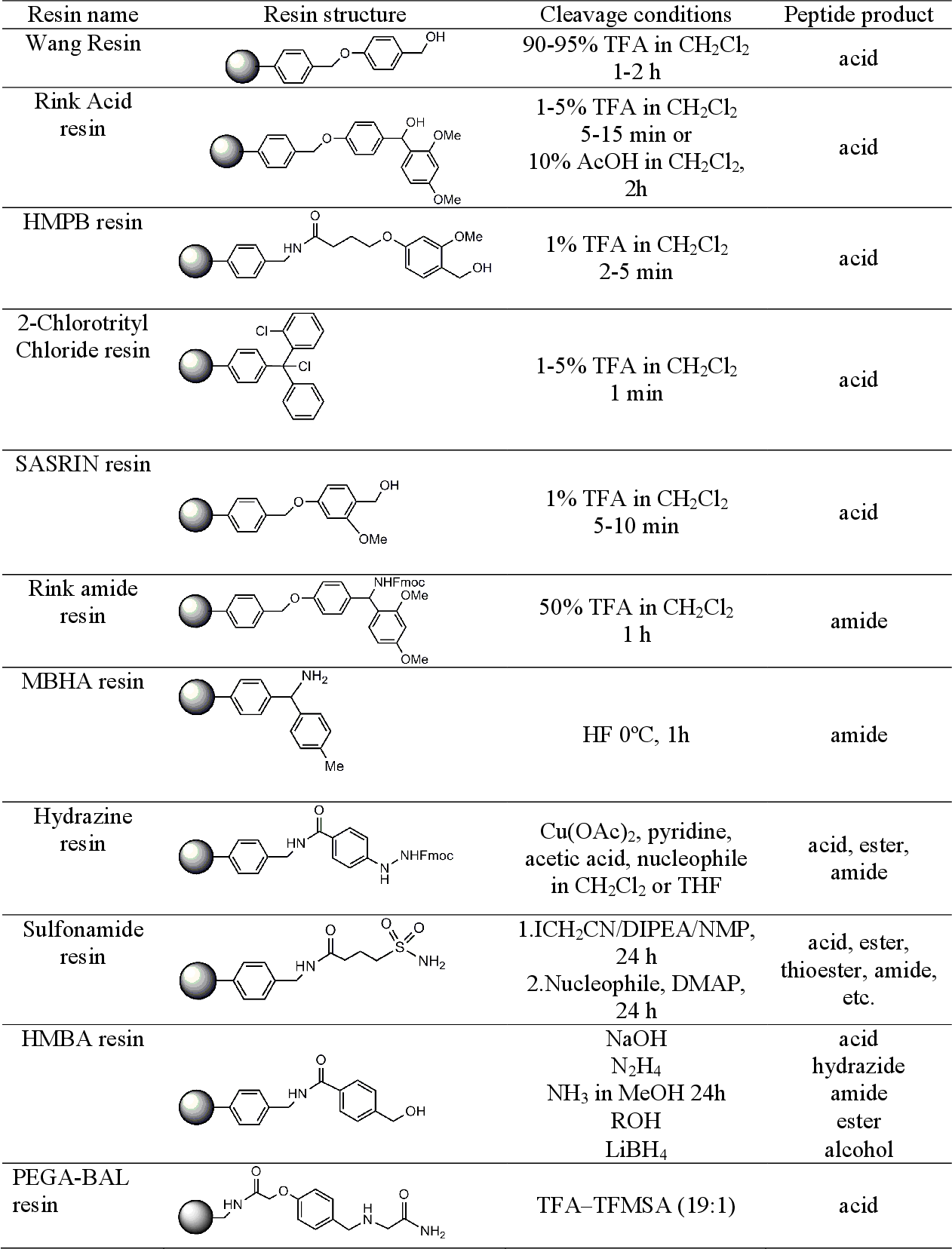
140 Citations
Challenges and perspectives in chemical synthesis of highly hydrophobic peptides, recent reports of solid-phase cyclohexapeptide synthesis and applications, heterocycles as a peptidomimetic scaffold: solid-phase synthesis strategies, decarboxylative couplings for late-stage peptide modifications., arylglycine: a focus on amino acid preparation and peptide synthesis, soluble non-cross-linked poly(norbornene) supports for peptide synthesis with minimal reagents., design and validation of a frugal, automated, solid-phase peptide synthesizer, applications of chemical ligation in peptide synthesis via acyl transfer., synthesis of self-assembling peptide-based hydrogels for regenerative medicine using solid-phase peptide synthesis., acylbenzotriazoles: new allies for short linear and cyclic peptide constructs, 82 references, solid-phase synthesis of lipidated ras peptides employing the ellman sulfonamide linker., fmoc solid phase peptide synthesis: a practical approach (practical approach series), solid‐phase peptide synthesis using acetonitrile as a solvent in combination with peg‐based resins, solid-phase peptide synthesis: from standard procedures to the synthesis of difficult sequences, acid-mediated prevention of aspartimide formation in solid phase peptide synthesis., highly efficient solid phase synthesis of large polypeptides by iterative ligations of bis(2-sulfanylethyl)amido (sea) peptide segments, a new approach for the synthesis of o-glycopeptides through a combination of solid-phase glycosylation and fluorous tagging chemistry (shgpft)., wang linker free of side reactions., total chemical synthesis of proteins., solid-phase synthesis of phosphopeptides., related papers.
Showing 1 through 3 of 0 Related Papers
- Advanced search
- Peer review
- Record : found
- Abstract : found
- Article : found

Recent Reports of Solid-Phase Cyclohexapeptide Synthesis and Applications

Read this article at
- Review article
- Invite someone to review
Macrocyclic peptides are privileged scaffolds for drug development and constitute a significant portion of macrocyclic drugs on the market today in fields spanning from infectious disease to oncology. Developing orally bioavailable peptide-based drugs remains a challenging task; however, macrocyclization of linear peptides can be an effective strategy to improve membrane permeability, proteolytic stability, oral bioavailability, and overall drug-like characteristics for this class. Significant advances in solid-phase peptide synthesis (SPPS) have enabled the efficient construction of macrocyclic peptide and peptidomimetic libraries with macrolactamization being performed on-resin or in solution phase. The primary goal of this review is to summarize solid-phase cyclohexapeptide synthesis using the on-resin and solution-phase macrocyclization methodologies published since 2013. We also highlight their broad applications ranging from natural product total synthesis, synthetic methodology development, and medicinal chemistry, to drug development and analyses of conformational and physiochemical properties.
Related collections
MDPI: Coronavirus
Most cited references 48
- Article : not found
The exploration of macrocycles for drug discovery--an underexploited structural class.

Macrocyclic drugs and clinical candidates: what can medicinal chemists learn from their properties?
Orally absorbed cyclic peptides., author and article information , contributors, affiliations, author notes, author information.
Licensee MDPI, Basel, Switzerland. This article is an open access article distributed under the terms and conditions of the Creative Commons Attribution (CC BY) license ( http://creativecommons.org/licenses/by/4.0/ ).
Comment on this article
Similar content 81 .
- Disintegration of solid foods in human stomach. Authors: F. Kong , Kameshwar Singh
- Exploring the Solubility and Bioavailability of Sodium Salt and Its Free Acid Solid Dispersions of Dolutegravir Authors: Dani Lakshman Yarlagadda , Akshatha M. Nayak , Bheemisetty Brahmam …
- A phase I study of intravenous and oral rucaparib in combination with chemotherapy in patients with advanced solid tumours Authors: Richard Wilson , TR Evans , Mark Middleton …
Most referenced authors 1,059

An official website of the United States government
The .gov means it’s official. Federal government websites often end in .gov or .mil. Before sharing sensitive information, make sure you’re on a federal government site.
The site is secure. The https:// ensures that you are connecting to the official website and that any information you provide is encrypted and transmitted securely.
- Publications
- Account settings
- My Bibliography
- Collections
- Citation manager
Save citation to file
Email citation, add to collections.
- Create a new collection
- Add to an existing collection
Add to My Bibliography
Your saved search, create a file for external citation management software, your rss feed.
- Search in PubMed
- Search in NLM Catalog
- Add to Search
Solid-Phase Synthesis and Antibacterial Activity of Cyclohexapeptide Wollamide B Analogs
Affiliations.
- 1 Department of Pharmaceutical Sciences, The Daniel K. Inouye College of Pharmacy , University of Hawai'i at Hilo , 34 Rainbow Drive , Hilo , Hawaii 96720 , United States.
- 2 Department of Chemical Biology & Therapeutics , St. Jude Children's Research Hospital , 262 Danny Thomas Place, MS#1000 , Memphis , Tennessee 38105 , United States.
- 3 Center for Infectious and Inflammatory Diseases , Texas A&M Health Science Center , 2121 West Holcombe Blvd , Houston , Texas 77030 , United States.
- PMID: 29431987
- DOI: 10.1021/acscombsci.7b00189
Herein we report the antibacterial structure-activity relationships of cyclic hexapeptide wollamide analogs derived from solid-phase library synthesis. Wollamide B, a cyclic hexapeptide natural product, has been previously found to have activity against Mycobacterium bovis. To further evaluate its antimycobacterial/antibacterial potential, 27 peptides including wollamides A/B, and desotamide B, were synthesized and subsequently tested against a panel of clinically significant bacterial pathogens. Biological evaluation revealed that the cyclic scaffold, amide functionality in position I, tryptophan residue in position V, and the original stereochemistry pattern of the core scaffold were key for antituberculosis and/or antibacterial activity. In addition, against M. tuberculosis and Gram-positive bacteria, residues in position II and/or VI greatly impacted antibacterial activity and selectivity. Wollamides A (3) and B (2) along with their corresponding II (l-Leu) analog 10 retained the most promising antituberculosis activity, with the lowest minimum inhibitory concentration (MIC) against virulent M. tuberculosis H37Rv (MIC = 1.56 μg/mL), as well as desirable selectivity indices (>100). Importantly, the antimicrobial activities of wollamides A and B do not result from disruption of the bacterial membrane, warranting further investigation into their mechanism of action.
Keywords: antibacterial; antituberculosis; cyclic hexapeptides; desotamide; solid-phase peptide synthesis; structure−activity relationships; wollamide.
PubMed Disclaimer
Similar articles
- Structure-Activity Relationships of Wollamide Cyclic Hexapeptides with Activity against Drug-Resistant and Intracellular Mycobacterium tuberculosis . Khalil ZG, Hill TA, De Leon Rodriguez LM, Lohman RJ, Hoang HN, Reiling N, Hillemann D, Brimble MA, Fairlie DP, Blumenthal A, Capon RJ. Khalil ZG, et al. Antimicrob Agents Chemother. 2019 Feb 26;63(3):e01773-18. doi: 10.1128/AAC.01773-18. Print 2019 Mar. Antimicrob Agents Chemother. 2019. PMID: 30602509 Free PMC article.
- Solid-Phase Synthesis of Wollamide Cyclohexapeptide Analogs. Prior AM, Sun D. Prior AM, et al. Methods Mol Biol. 2020;2103:175-187. doi: 10.1007/978-1-0716-0227-0_11. Methods Mol Biol. 2020. PMID: 31879925
- Total Synthesis and Antibacterial Study of Cyclohexapeptides Desotamide B, Wollamide B and Their Analogs. Chen YX, Liu C, Liu N, Wu Y, Zhao QJ, Hu HG, Li X, Zou Y. Chen YX, et al. Chem Biodivers. 2018 Jan;15(1). doi: 10.1002/cbdv.201700414. Epub 2017 Dec 27. Chem Biodivers. 2018. PMID: 29125222
- Recent Reports of Solid-Phase Cyclohexapeptide Synthesis and Applications. Prior AM, Hori T, Fishman A, Sun D. Prior AM, et al. Molecules. 2018 Jun 18;23(6):1475. doi: 10.3390/molecules23061475. Molecules. 2018. PMID: 29912160 Free PMC article. Review.
- Full solid-phase total synthesis of macrocyclic natural peptides using four-dimensionally orthogonal protective groups. Itoh H, Inoue M. Itoh H, et al. Org Biomol Chem. 2019 Jul 21;17(27):6519-6527. doi: 10.1039/c9ob01130g. Epub 2019 Jun 24. Org Biomol Chem. 2019. PMID: 31232404 Review.
- Wollamide Cyclic Hexapeptides Synergize with Established and New Tuberculosis Antibiotics in Targeting Mycobacterium tuberculosis. Rollo RF, Mori G, Hill TA, Hillemann D, Niemann S, Homolka S, Fairlie DP, Blumenthal A. Rollo RF, et al. Microbiol Spectr. 2023 Aug 17;11(4):e0046523. doi: 10.1128/spectrum.00465-23. Epub 2023 Jun 8. Microbiol Spectr. 2023. PMID: 37289062 Free PMC article.
- Compelling Cyclic Peptide Scaffolds for Antitubercular Action: An Account (2011-21) of the Natural Source. Chowdhury A, Bandyopadhyay A. Chowdhury A, et al. Curr Protein Pept Sci. 2022;23(12):823-836. doi: 10.2174/1389203723666220930111259. Curr Protein Pept Sci. 2022. PMID: 36200246 Review.
- Bifurcation drives the evolution of assembly-line biosynthesis. Booth TJ, Bozhüyük KAJ, Liston JD, Batey SFD, Lacey E, Wilkinson B. Booth TJ, et al. Nat Commun. 2022 Jun 17;13(1):3498. doi: 10.1038/s41467-022-30950-z. Nat Commun. 2022. PMID: 35715397 Free PMC article.
- Revealing the Mechanisms of Synergistic Action of Two Magainin Antimicrobial Peptides. Bechinger B, Juhl DW, Glattard E, Aisenbrey C. Bechinger B, et al. Front Med Technol. 2020 Dec 21;2:615494. doi: 10.3389/fmedt.2020.615494. eCollection 2020. Front Med Technol. 2020. PMID: 35047895 Free PMC article. Review.
Publication types
- Search in MeSH
Related information
- PubChem Compound (MeSH Keyword)
Grants and funding
- R15 AI092315/AI/NIAID NIH HHS/United States
- P20 GM103466/GM/NIGMS NIH HHS/United States
LinkOut - more resources
Full text sources.
- American Chemical Society
Other Literature Sources
- scite Smart Citations
- MedlinePlus Health Information
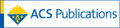
- Citation Manager
NCBI Literature Resources
MeSH PMC Bookshelf Disclaimer
The PubMed wordmark and PubMed logo are registered trademarks of the U.S. Department of Health and Human Services (HHS). Unauthorized use of these marks is strictly prohibited.
Europe PMC requires Javascript to function effectively.
Either your web browser doesn't support Javascript or it is currently turned off. In the latter case, please turn on Javascript support in your web browser and reload this page.

Scheme 3. Solid-phase synthesis of 9 and 10 on 2-chlorotrityl chloride (2-CTC) resin. Reagents and conditions: (a) Fmoc-Leu-OH, DIPEA, and DCM (r.t., overnight); (b) treated twice with 50% piperidine/DMF (r.t., 10 min); (c) Fmoc-Leu-OH, HATU, DIPEA, and DMF (r.t., 2 h); (d) TFA/TIPS/H2O (95/2.5/2.5) (r.t., 2 h); (e) dropwise addition of linear peptide solution in DMF to solution of PyBOP, DIPEA, and DMF (over 3 h, r.t., overnight; 17% yield for 9 and 3% for 10 after preparative RP-HPLC purification).

Contexts in source publication
- Dianqing Sun

- Gudapati Krupamai

- Meth Mol Biol

- Bimal Krishna Banik
- George Nkrumah Enninful

- BIOORG CHEM
- Xiaobin Zhuo
- J HETEROCYCLIC CHEM

- Dessy Yulyani Kurnia

- Achmad Zainuddin

- MINI-REV MED CHEM

- Rita Mourya

- NAT PROD RES
- Iqra Kanwal
- Farkhanda Mushtaq

- Farzana Shaheen
- ORG BIOMOL CHEM

- Bradley D. Smith
- Recruit researchers
- Join for free
- Login Email Tip: Most researchers use their institutional email address as their ResearchGate login Password Forgot password? Keep me logged in Log in or Continue with Google Welcome back! Please log in. Email · Hint Tip: Most researchers use their institutional email address as their ResearchGate login Password Forgot password? Keep me logged in Log in or Continue with Google No account? Sign up

IMAGES
VIDEO
COMMENTS
The primary goal of this review is to summarize solid-phase cyclohexapeptide synthesis using the on-resin and solution-phase macrocyclization methodologies published since 2013. We also highlight their broad applications ranging from natural product total synthesis, synthetic methodology development, and medicinal chemistry, to drug development ...
The primary goal of this review is to summarize solid-phase cyclohexapeptide synthesis using the on-resin and solution-phase macrocyclization methodologies published since 2013. We also highlight their broad applications ranging from natural product total synthesis, synthetic methodology development, and medicinal chemistry, to drug development ...
Solid-phase peptide synthesis (SPPS) was first described in 1963 by Merrifield [ 25 ], whereby a growing linear peptide was synthesized in a step-wise fashion while covalently attached to a solid support (resin). In general, excess reagents are used in solid-phase synthesis to help to drive reactions to completion.
Solid-phase cyclohexapeptide synthesis using the on-resin and solution-phase macrocyclization methodologies published since 2013 are summarized to highlight their broad applications ranging from natural product total synthesis, synthetic methodology development, and medicinal chemistry, to drug development and analyses of conformational and physiochemical properties. Macrocyclic peptides are ...
The primary goal of this review is to summarize solid-phase cyclohexapeptide synthesis using the on-resin and solution-phase macrocyclization methodologies published since 2013. We also highlight ...
Recent Reports of Solid-Phase Cyclohexapeptide Synthesis and Applications. https://doi.org/10.3390/molecules23061475 Journal: Molecules, 2018, № 6, p. 1475 ...
Solid-phase synthesis of antibacterial cyclohexapeptides including wollamides A, B and desotamide B has been developed. ... Recent Reports of Solid-Phase Cyclohexapeptide Synthesis and Applications. Prior AM, Hori T, Fishman A, Sun D. Molecules, 23(6):E1475, 18 Jun 2018 Cited ...
Solid-phase peptide synthesis (SPPS) was first described in 1963 by Merrifield [25], whereby a growing linear peptide was synthesized in a step-wise fashion while covalently attached to a solid support (resin). In general, excess reagents are used in solid-phase synthesis to help to drive reactions to completion.
Herein we report the antibacterial structure-activity relationships of cyclic hexapeptide wollamide analogs derived from solid-phase library synthesis. Wollamide B, a cyclic hexapeptide natural product, has been previously found to have activity against Mycobacterium bovis .
This Special Issue of Molecules is dedicated to "Solid-Phase Synthesis". The concept of solid-phase (SP) peptide synthesis was revealed by Bruce Merrifield in 1963 and its potential for general organic chemistry was soon recognized. However, it was not until the onset of combinatorial chemistry in early 1990s when the application of SP ...
Solid-Phase Synthesis of Wollamide Cyclohexapeptide Analogs Methods Mol Biol. 2020;2103:175-187. doi: 10.1007/978-1-0716-0227-0_11. ... This chapter describes the detailed synthesis of antitubercular wollamide analogs using solid-phase synthesis of linear hexapeptide precursors, followed by solution-phase HBTU-mediated macrocyclization and ...
Download scientific diagram | Synthesis and structure-activity relationship (SAR) studies of cyclohexapeptides 48, 52, and 42. from publication: Recent Reports of Solid-Phase Cyclohexapeptide ...
Recent Reports of Solid-Phase Cyclohexapeptide Synthesis and Applications Recent Reports of Solid-Phase Cyclohexapeptide Synthesis and Applications. Access Restriction Open. Requires : PDF viewer Plugin. Author: Prior, Allan M. ♦ Hori, Taylor ♦ Sun, Dianqing ♦ Fishman, Ashriel:
Solid-phase cyclohexapeptide synthesis using the on-resin and solution-phase macrocyclization methodologies published since 2013 are summarized to highlight their broad applications ranging from natural product total synthesis, synthetic methodology development, and medicinal chemistry, to drug development and analyses of conformational and ...
The primary goal of this review is to summarize solid-phase cyclohexapeptide synthesis using the on-resin and solution-phase macrocyclization methodologies published since 2013. We also highlight their broad applications ranging from natural product total synthesis, synthetic methodology development, and medicinal chemistry, to drug development ...
Recent Reports of Solid-Phase Cyclohexapeptide Synthesis and Applications. Prior AM, Hori T, Fishman A, Sun D. Molecules, 23(6):E1475, 18 Jun 2018 Cited by: 2 articles | PMID: 29912160 | PMCID: PMC6100019. Review Free to read & use
P20 GM103466/GM/NIGMS NIH HHS/United States. Herein we report the antibacterial structure-activity relationships of cyclic hexapeptide wollamide analogs derived from solid-phase library synthesis. Wollamide B, a cyclic hexapeptide natural product, has been previously found to have activity against Mycobacterium bovis. To further evaluate its a ….
Recent Reports of Solid-Phase Cyclohexapeptide Synthesis and Applications. Prior AM, Hori T, Fishman A, Sun D. Molecules, 23(6):E1475, 18 Jun 2018 Cited by: 2 articles | PMID: 29912160 | PMCID: PMC6100019. Review Free full text in Europe PMC ...
from publication: Recent Reports of Solid-Phase Cyclohexapeptide Synthesis and Applications | Macrocyclic peptides are privileged scaffolds for drug development and constitute a significant ...
The total synthesis of the cyclic hexapeptides PF1171A, C, F, and G has been achieved by solid-phase synthesis of a linear precursor and solution-phase macrolactamization. The synthesis includes a solid-phase peptide coupling with the weakly nucleophilic amino group of an anthranilic acid residue. This was efficiently achieved by in situ generation of an Fmoc-amino acid chloride using ...
from publication: Recent Reports of Solid-Phase Cyclohexapeptide Synthesis and Applications | Macrocyclic peptides are privileged scaffolds for drug development and constitute a significant ...