Thank you for visiting nature.com. You are using a browser version with limited support for CSS. To obtain the best experience, we recommend you use a more up to date browser (or turn off compatibility mode in Internet Explorer). In the meantime, to ensure continued support, we are displaying the site without styles and JavaScript.
- View all journals
- Explore content
- About the journal
- Publish with us
- Sign up for alerts
- Open access
- Published: 21 February 2023

Life on Mars, can we detect it?
- Carol R. Stoker ORCID: orcid.org/0000-0001-7265-292X 1
Nature Communications volume 14 , Article number: 807 ( 2023 ) Cite this article
5505 Accesses
2 Citations
310 Altmetric
Metrics details
- Astrobiology
- Biochemistry
Searching for evidence of life on Mars is a major impetus for exploration. A new study published in Nature Communications finds that current Mars mission instruments lack the essential sensitivity to identify life traces in Chilean desert samples that strongly resemble the martian area currently under study by NASA’s Perseverance rover.
Almost half a century ago the NASA Viking landers searched for evidence of life in Mars soils by attempting to detect active metabolism and measuring organic compounds by heating to vaporize them for detection via Gas Chromatography Mass Spectrometry (GC-MS). When no organic compounds were detected even at levels of parts per billion 1 , this provided a puzzle. Since organic compounds are continuously delivered to Mars by meteorites, comets, and interplanetary dust particles they should be ubiquitous and comprise up to 2% of Mars soil 2 . But where are they? This curious lack of organics argued both against a biological interpretation for results of the metabolism experiments, and for the presence of strong soil oxidants that actively destroy these compounds 3 . However, decades later the Viking GC-MS results were explained by the presence of high levels of perchlorate salts in the Martian soil 4 that oxidized organics during pyrolysis in the Viking GC-MS instrument 5 .
While Viking lander results seemed unpromising for surface life in the cold, dry martian conditions, data from Viking orbiters and later missions showed that Mars had surface liquid water early in its history (3 to 4 billion years ago) 6 where life may have thrived. This led later NASA rover missions to seek evidence of ancient habitable environments hosting organic remnants of life. The SAM ( Sample Analysis on Mars ) instrument on the Curiosity rover detected organics in ancient lakebed deposits 7 , 8 , but they are found at low levels (parts per billion) and appear to be highly altered. The Perseverance rover, now exploring an ancient illuvial fan river delta, has detected aromatic organic compounds using fluorescence spectroscopy 9 . But in these cases, the simple organics detected could have been delivered to Mars from space so proving a biological origin is impossible.
The paper by Azua-Bustos et al. 10 in Nature Communications describes analysis of samples collected from “Red Stone”, an alluvial fan river delta more than 100 million years old in the Atacama Desert of Chile, Earth’s oldest and driest desert. Red Stone has similar geology to the delta area currently being studied by NASA’s Perseverance rover 11 (Fig. 1 ). The Red Stone location (near the ocean) frequently experiences fogs that provide water for sparse but active microbial life that was detected by DNA extraction and gene sequencing, microscopy, and growth of a few microbial strains cultured from the samples. Most microbes identified consist of “microbial dark matter” i.e., genetic information is from organisms that have not yet been described.
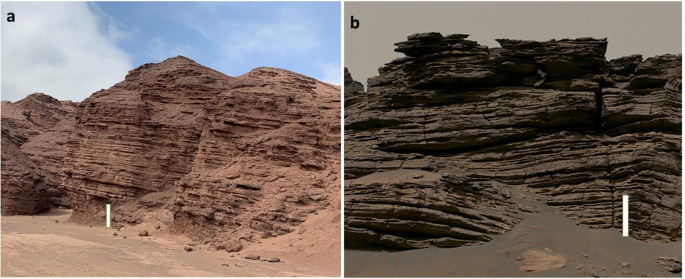
a Red Stone deposit from panorama image courtesy of Armando Azua-Bustos. b A section of the panoramic composite image [image PIA24921_MAIN-20k.jpg] of the Jezero delta captured by the MastCam-Z camera on the Perseverance Rover in June 2022 15 (Courtesy NASA/JPL-Caltech/ASU/MSSS). The height of both white scale bars are ~2 m.
Samples from Red Stone were also analyzed by instruments that simulate those used on Mars, but even finding organics in these samples was challenging. A GC-MS instrument comparable to but ten times more sensitive than Curiosity rover’s SAM instrument was barely able to detect specific biogenic organic compounds at the limit of detection, suggesting that SAM could not have detected them. There was more success with organic detection after sample extraction with a powerful solvent that was also implemented on the SAM instrument. Indeed, the SAM instrument detected organics in both sedimentary mudstones and dune sands on Mars by using solvent extraction and derivatization (a chemical treatment to make organic compounds easier to detect) 8 .
Red Stone samples were analyzed with a testbed of the MOMA (Mars Organic Molecular Analysis) instrument 12 planned for the European ExoMars rover payload. No organics were detected when flash pyrolysis was used, but a few organic molecules were identified in evaporite samples when they were first extracted with a solvent and derivatized.
Red Stone samples were also analyzed with the SOLID-LDChip (Signs of Life Detector-Life Detector Chip) 13 , an instrument based on the technology of immunoassay that is commonly used in biomedicine. SOLID was designed for life detection on Mars but no scheduled missions plan to use it. Interestingly, the LDChip detected evidence of cyanobacteria that were not seen in the modern microbiota so Azua-Bustos et al. 10 posit these biosignatures were deposited along with the delta sediments at least 100 million years ago.
This Red Stone sample analysis 10 shows how critical it is to test instruments designed for life detection on other planets by using samples from relevant Earth analogs prior to selecting them for flight missions. If the biosignatures can’t be detected in Earth samples, where both current and ancient life is clearly documented, we should not expect these instruments to be capable of detecting evidence of life from Mars’ early history.
Azua-Bustos et al. 10 argue that detection of ancient life signatures will require sample analysis in sophisticated terrestrial laboratories. This same view has led to the current plans for searching for life: the Perseverance rover is collecting samples that are to be retrieved and brought to Earth by a future mission 14 . But any biological activity in these samples presumably took place billions of years ago, and only a few small samples can be brought to Earth for study. It remains to be seen if unambiguous signatures of life can be found in those limited samples. We must be cautious about interpreting absence of strong evidence of life as evidence of its absence!
Biemann, K. & Lavoi, J. M. Jr Some final conclusions and supporting experiments related to the search for organic compounds on the surface of Mars. J. Geophys. Res. 84 , 8385–8390 (1979).
Article CAS ADS Google Scholar
Flynn, G. J. & McKay, D. S. An assessment of the meteoritic contribution to the Martian soil. J. Geophys. Res. 95 , 14,497–14,509 (1990).
Article ADS Google Scholar
Klein, H. P. The Viking biological experiments on Mars. Icarus 34 , 666–674 (1978).
Hecht, M. H. et al. Detection of perchlorate and the soluble chemistry of martian soil: findings from the Phoenix Mars Lander. Science 325 , 64–67 (2009).
Article CAS ADS PubMed Google Scholar
Navarro‐González, R. et al. Reanalysis of the Viking results suggests perchlorate and organics at midlatitudes on Mars. J. Geophys. Res. 115 , E12010 (2010).
Carr, M. H. & Head, J. W. III Geologic history of Mars. Earth Planet. Sci. Lett. 294 , 185–203 (2010).
Eigenbrode, J. L. et al. Organic matter preserved in 3-billion-year-old mudstones at Gale crater, Mars. Science 360 , 1096–1101 (2018).
Millan, M. et al. Sedimentary organics in Glen Torridon, Gale Crater, Mars: Results from the SAM instrument suite and supporting laboratory analyses. J. Geophys. Res. Planets 127 , e2021JE007107 (2022).
Scheller, E. L. et al. Aqueous alteration processes in Jezero Crater Mars- implications for organic geochemistry. Science 378 , 1105–1110 (2022).
Azua-Bustos, A. et al. Dark microbiome and extremely low organics in an Atacama fossil river delta unveil the limits of life detection on Mars. Nat. Commun. this issue . https://doi.org/10.1038/s41467-023-36172-1 (2023).
Farley, K. A. et al. Aqueously altered igneous rocks sampled on the floor of Jezero Crater. Science 377 , 1512 (2022).
Article Google Scholar
Goesmann, F. et al. The Mars Organic Molecule Analyzer (MOMA) instrument: characterization of organic material in martian sediments. Astrobiology 17 , 655–685 (2017).
Article CAS ADS PubMed PubMed Central Google Scholar
Parro, V. et al. SOLID3, a multiplex antibody microarray-based optical sensor instrument for in situ life detection in planetary exploration. Astrobiology 11 , 15–28 (2011).
Haltigen, T. et al. Rationale and Proposed Design for a Mars Sample Return (MSR) Science Program. Astrobiology 22 , S27–S55 (2022).
A small segment of a panorama comprised of Perseverance Rover MastCam-Z images (image PIA24921_Main-20k.jpg) taken June 2022. The full panoramic image is available for download at https://mars.nasa.gov/resources/26978/detailed-panorama-of-mars-jezero-crater-delta/ .
Download references
Author information
Authors and affiliations.
NASA Ames Research Center, Space Science Division, MS 245-3, Moffett Field, California, CA, 94035, USA
Carol R. Stoker
You can also search for this author in PubMed Google Scholar
Contributions
CRS is the sole author.
Corresponding author
Correspondence to Carol R. Stoker .
Ethics declarations
Competing interests.
The author declares no competing interests.
Additional information
Publisher’s note Springer Nature remains neutral with regard to jurisdictional claims in published maps and institutional affiliations.
Rights and permissions
Open Access This article is licensed under a Creative Commons Attribution 4.0 International License, which permits use, sharing, adaptation, distribution and reproduction in any medium or format, as long as you give appropriate credit to the original author(s) and the source, provide a link to the Creative Commons license, and indicate if changes were made. The images or other third party material in this article are included in the article’s Creative Commons license, unless indicated otherwise in a credit line to the material. If material is not included in the article’s Creative Commons license and your intended use is not permitted by statutory regulation or exceeds the permitted use, you will need to obtain permission directly from the copyright holder. To view a copy of this license, visit http://creativecommons.org/licenses/by/4.0/ .
Reprints and permissions
About this article
Cite this article.
Stoker, C.R. Life on Mars, can we detect it?. Nat Commun 14 , 807 (2023). https://doi.org/10.1038/s41467-023-36176-x
Download citation
Received : 14 December 2022
Accepted : 09 January 2023
Published : 21 February 2023
DOI : https://doi.org/10.1038/s41467-023-36176-x
Share this article
Anyone you share the following link with will be able to read this content:
Sorry, a shareable link is not currently available for this article.
Provided by the Springer Nature SharedIt content-sharing initiative
Quick links
- Explore articles by subject
- Guide to authors
- Editorial policies
Sign up for the Nature Briefing newsletter — what matters in science, free to your inbox daily.

- Frontiers in Astronomy and Space Sciences
- Astrobiology
- Research Topics
Advances in Mars Research and Exploration
Total Downloads
Total Views and Downloads
About this Research Topic
The pursuit of finding habitable conditions or life outside our planet has always been fascinating. In terms of habitability, Mars is the most Earth‐like planet within our solar system as it displays the highest Earth similarity index of 0.7 based on physical determinants such as radius, mass, and temperature ...
Keywords : Mars, remote sensing, geomorphology, astrobiology, geology
Important Note : All contributions to this Research Topic must be within the scope of the section and journal to which they are submitted, as defined in their mission statements. Frontiers reserves the right to guide an out-of-scope manuscript to a more suitable section or journal at any stage of peer review.
Topic Editors
Topic coordinators, recent articles, submission deadlines.
Submission closed.
Participating Journals
Total views.
- Demographics
No records found
total views article views downloads topic views
Top countries
Top referring sites, about frontiers research topics.
With their unique mixes of varied contributions from Original Research to Review Articles, Research Topics unify the most influential researchers, the latest key findings and historical advances in a hot research area! Find out more on how to host your own Frontiers Research Topic or contribute to one as an author.
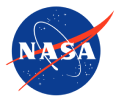
Mars Science Laboratory: Curiosity Rover
Curiosity's scientific tools found chemical and mineral evidence of past habitable environments on Mars. It continues to explore the rock record from a time when Mars could have been home to microbial life.
Landing at Gale Crater, Mars Science Laboratory is assessing whether Mars ever had an environment capable of supporting microbial life. Determining past habitability on Mars gives NASA and the scientific community a better understanding of whether life could have existed on the Red Planet and, if it could have existed, an idea of where to look for it in the future.
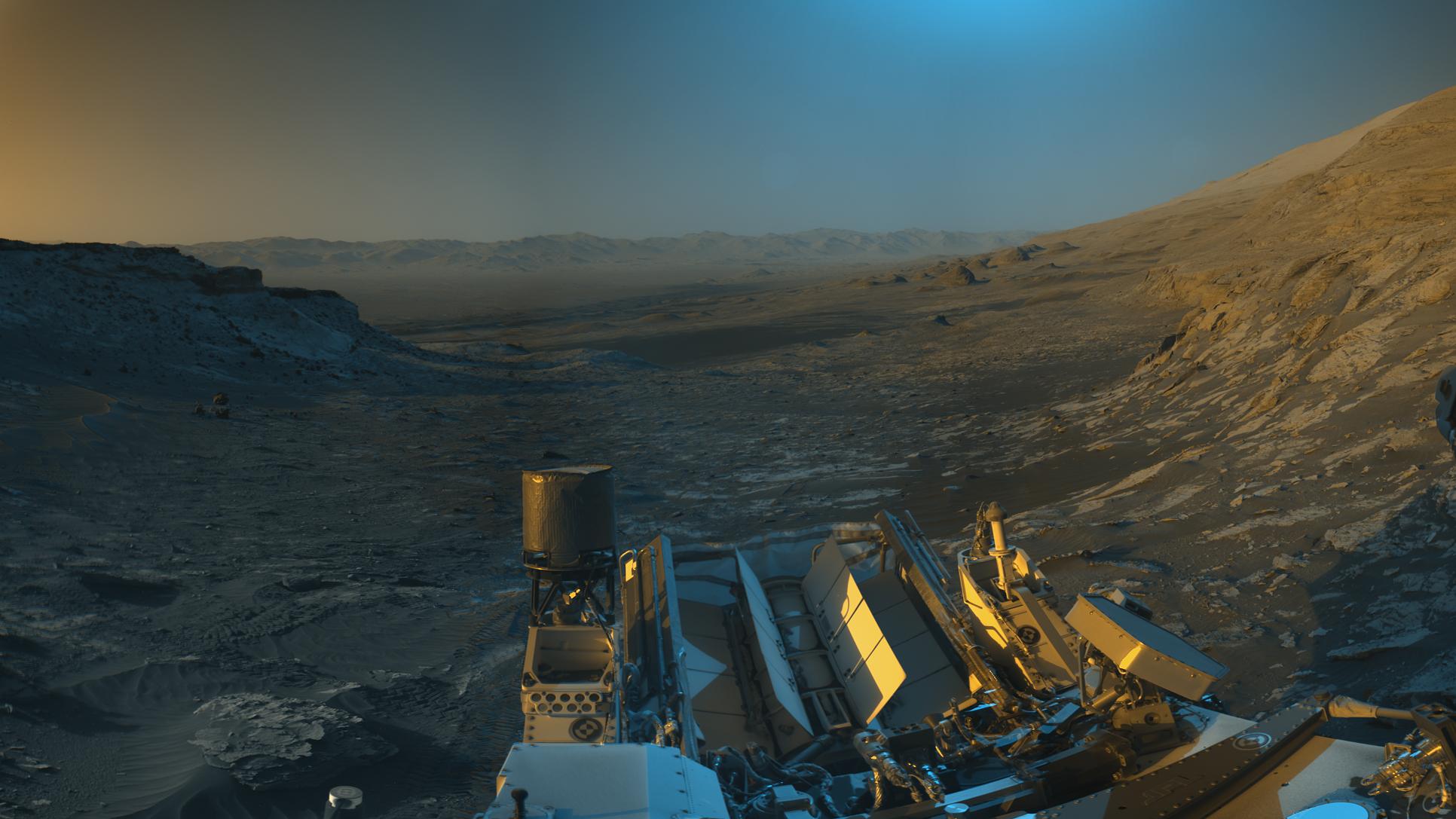
To contribute to the four Mars exploration science goals and meet its specific goal of determining Mars' habitability, Curiosity has the following science objectives:
Biological objectives
Geological and geochemical objectives, planetary process objectives, surface radiation objective.
1. Determine the nature and inventory of organic carbon compounds 2. Inventory the chemical building blocks of life (carbon, hydrogen, nitrogen, oxygen, phosphorous, and sulfur) 3. Identify features that may represent the effects of biological processes
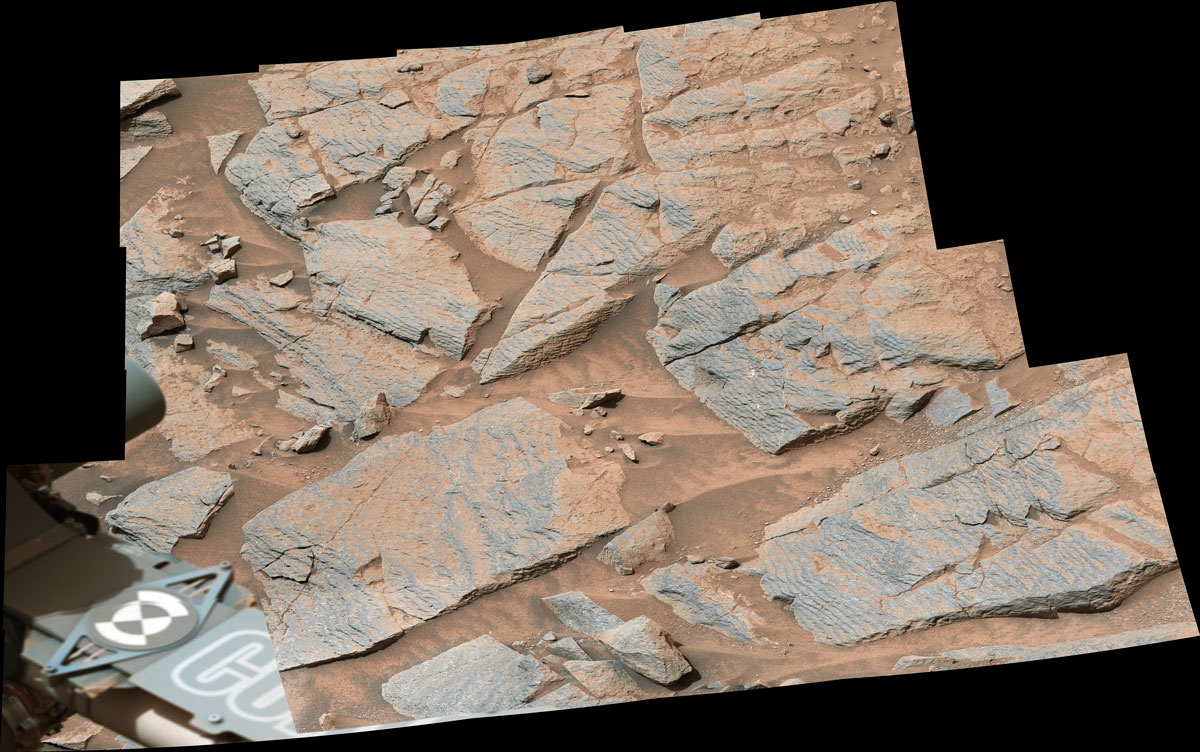
1. Investigate the chemical, isotopic, and mineralogical composition of the Martian surface and near-surface geological materials 2. Interpret the processes that have formed and modified rocks and soils
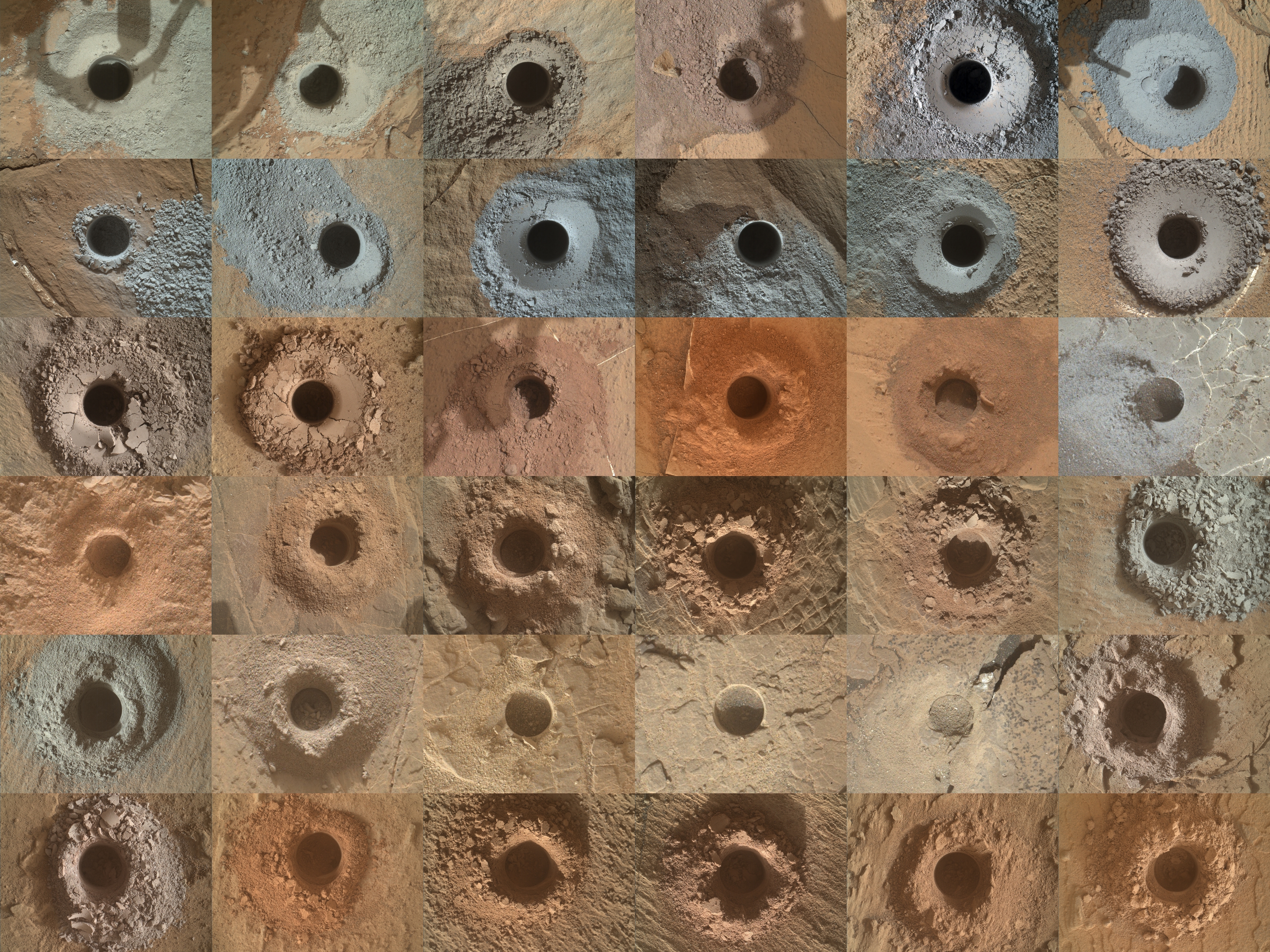
1. Assess long-timescale (i.e., 4-billion-year) atmospheric evolution processes 2. Determine present state, distribution, and cycling of water and carbon dioxide
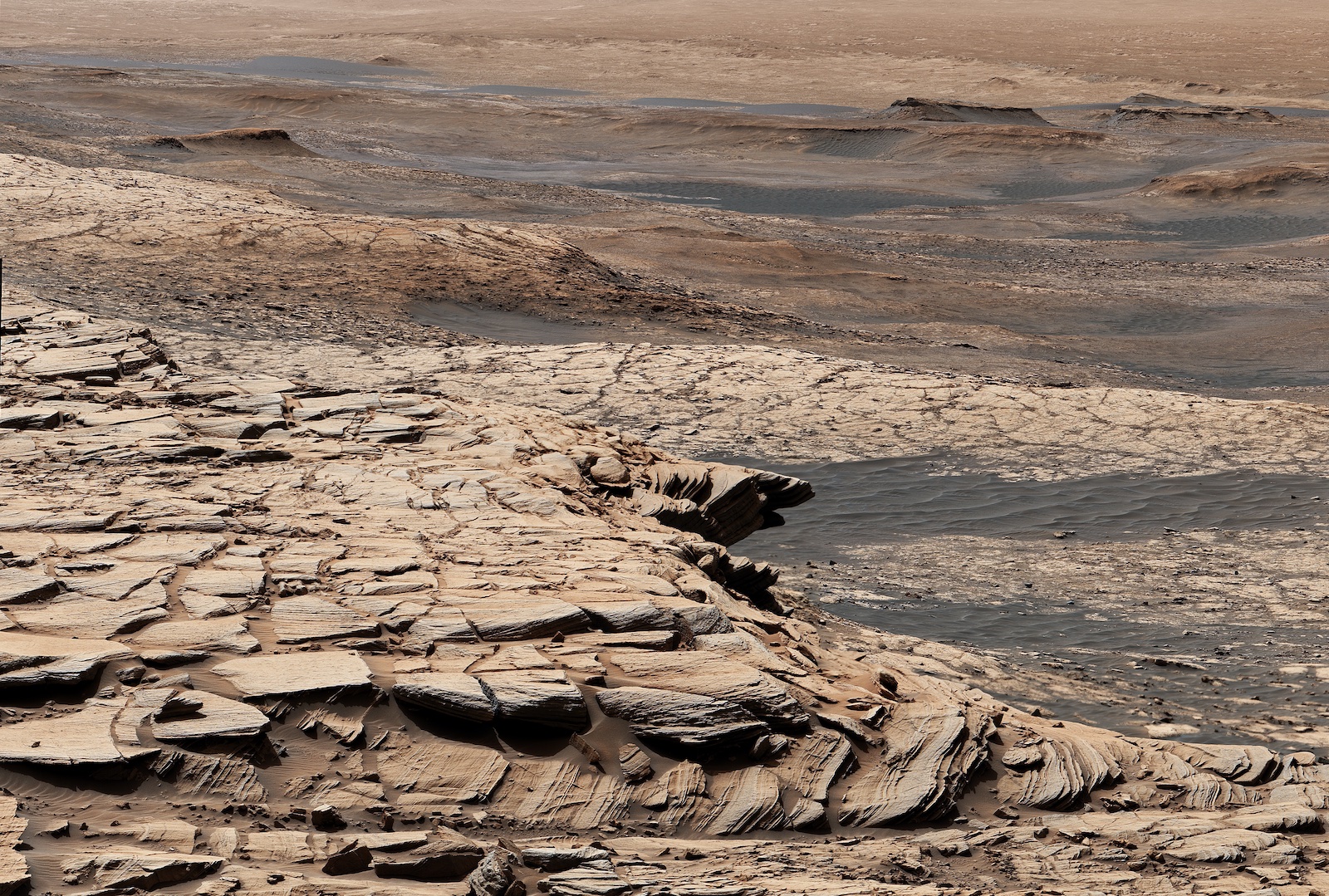
Characterize the broad spectrum of surface radiation, including galactic cosmic radiation, solar proton events, and secondary neutrons
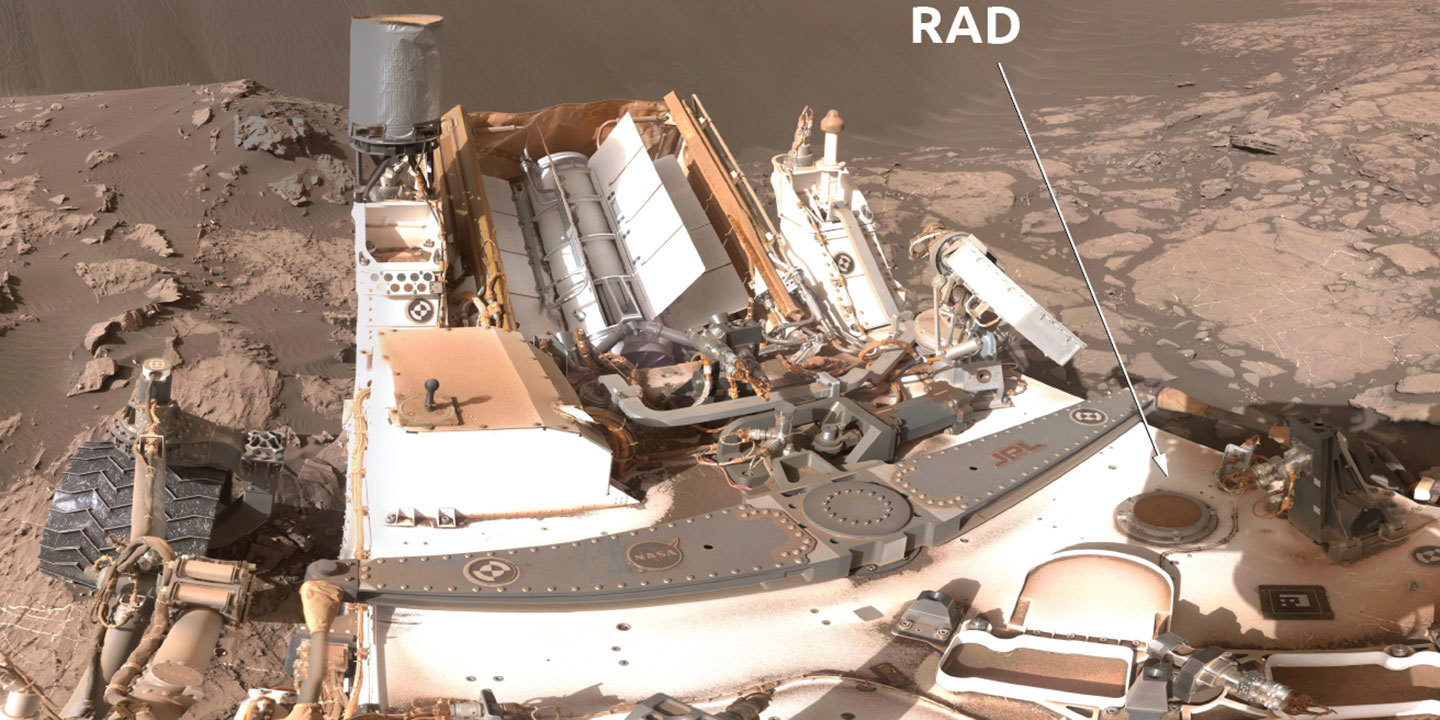
Science Highlights
With over a decade of exploration, Curiosity has unveiled the keys to some of science's most unanswered questions about Mars. Did Mars ever have the right environmental conditions to support small life forms called microbes? Early in its mission, Curiosity's scientific tools found chemical and mineral evidence of past habitable environments on Mars. It continues to explore the rock record from a time when Mars could have been home to microbial life.
Science Instruments
From cameras to environmental and atmospheric sensors, the Curiosity rover has a suite of state-of-the-art science instruments to achieve its goals.
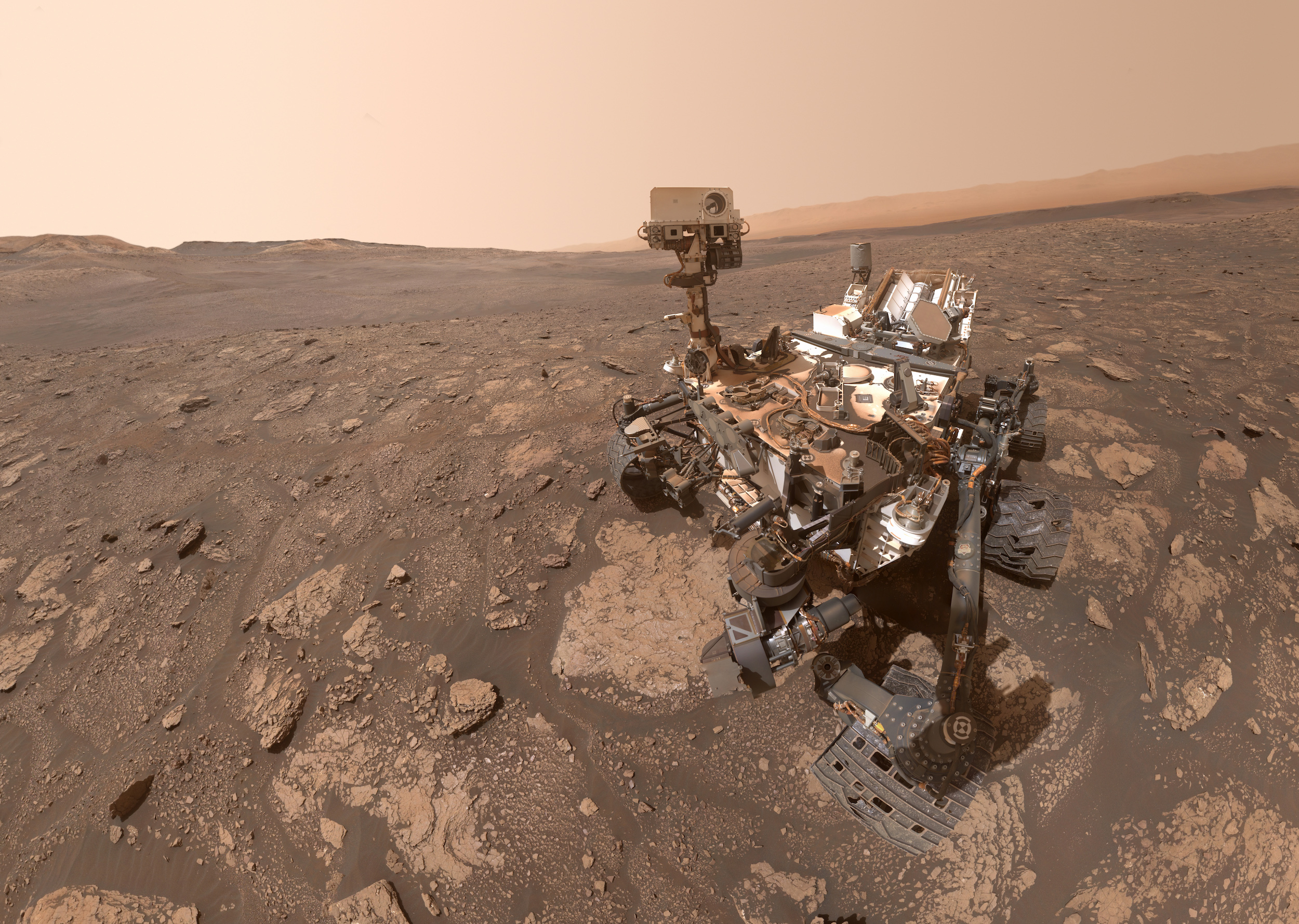
Discover More Topics From NASA
James Webb Space Telescope
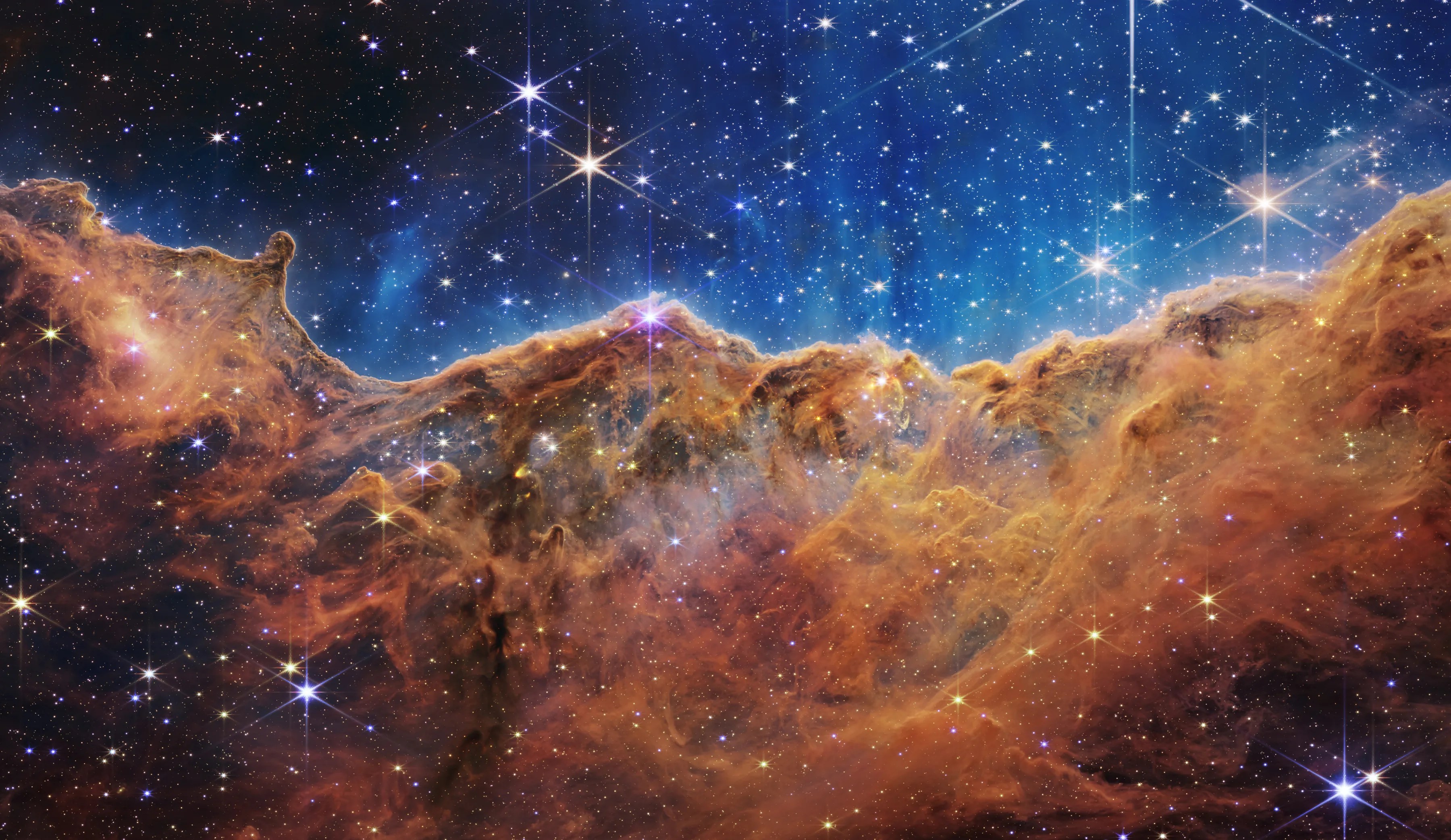
Perseverance Rover
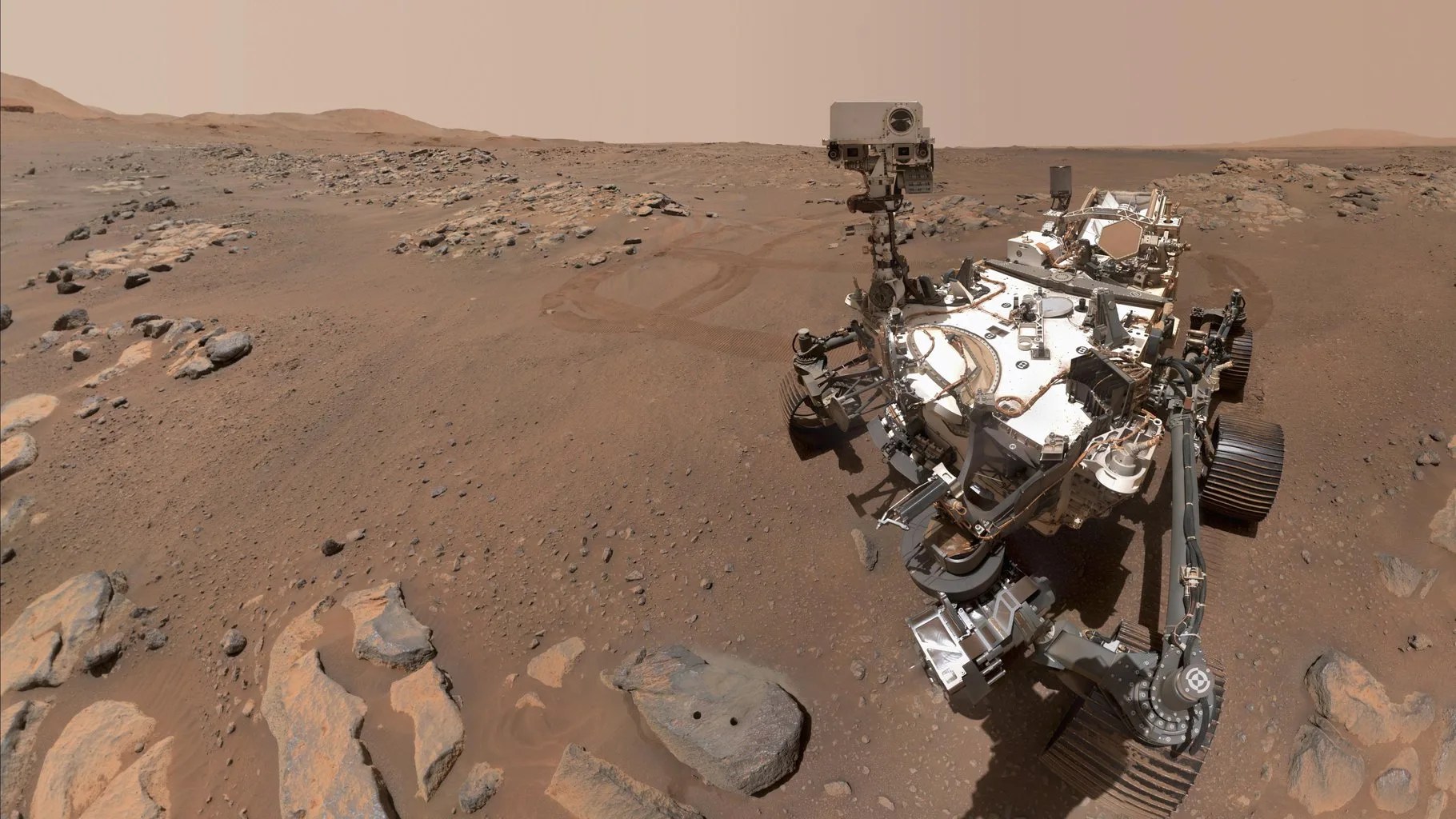
Parker Solar Probe
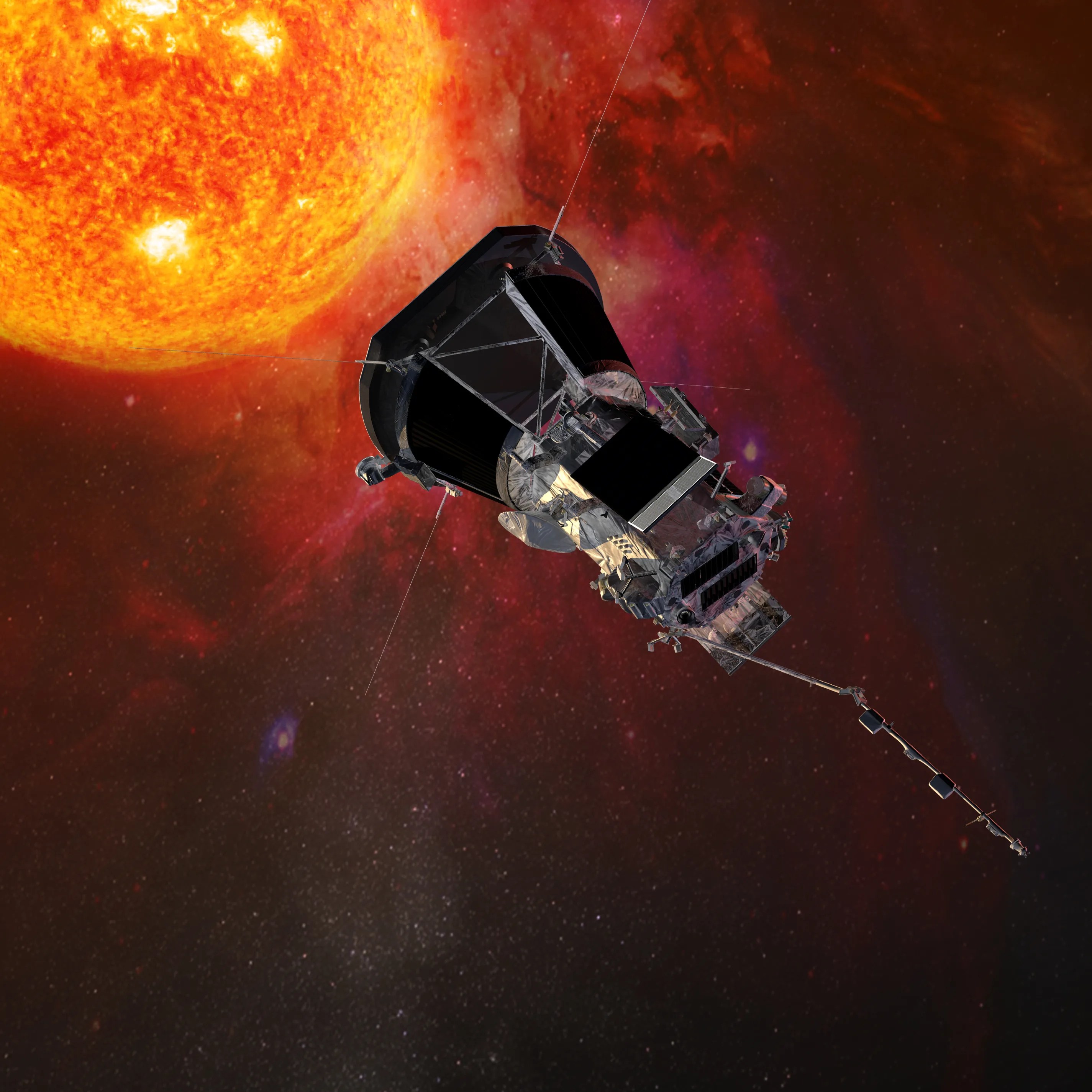
Here’s How AI Is Changing NASA’s Mars Rover Science
Your browser cannot play the provided video file(s).
In this time-lapse video of a test conducted at JPL in June 2023, an engineering model of the Planetary Instrument for X-ray Lithochemistry (PIXL) instrument aboard NASA’s Perseverance Mars rover places itself against a rock to collect data. Credit: NASA/JPL-Caltech Full Image Details
Artificial intelligence is helping scientists to identify minerals within rocks studied by the Perseverance rover.
Some scientists dream of exploring planets with “smart” spacecraft that know exactly what data to look for, where to find it, and how to analyze it. Although making that dream a reality will take time, advances made with NASA’s Perseverance Mars rover offer promising steps in that direction.
For almost three years, the rover mission has been testing a form of artificial intelligence that seeks out minerals in the Red Planet’s rocks. This marks the first time AI has been used on Mars to make autonomous decisions based on real-time analysis of rock composition.
The software supports PIXL (Planetary Instrument for X-ray Lithochemistry), a spectrometer developed by NASA’s Jet Propulsion Laboratory in Southern California. By mapping the chemical composition of minerals across a rock’s surface, PIXL allows scientists to determine whether the rock formed in conditions that could have been supportive of microbial life in Mars’ ancient past.
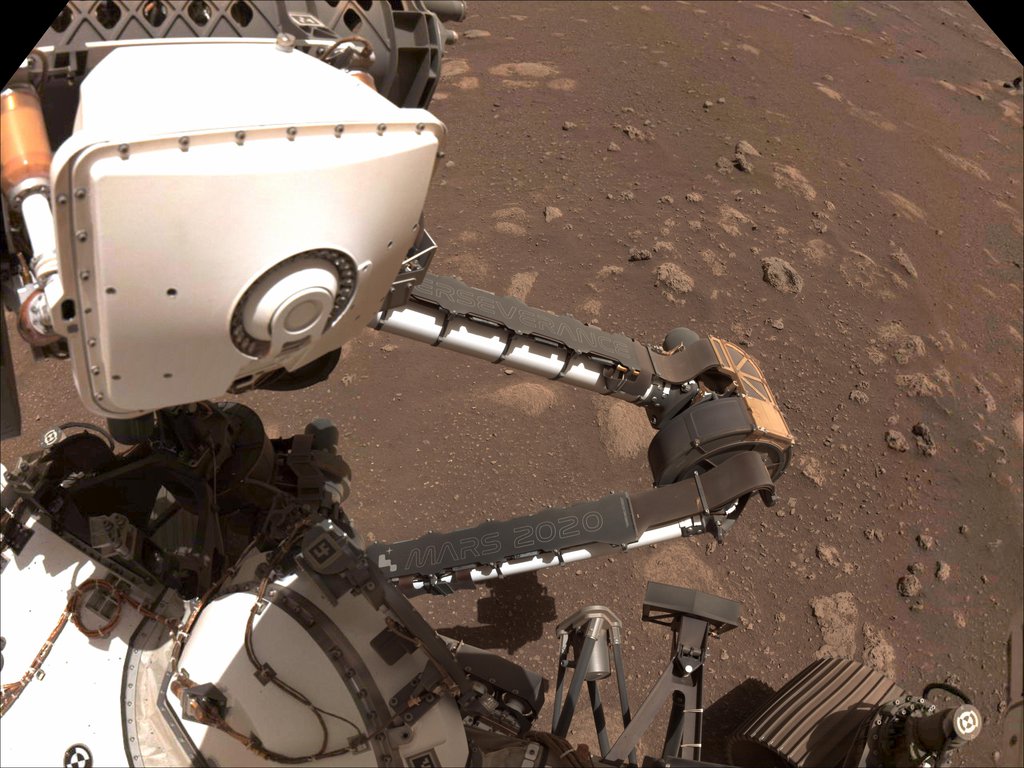
PIXL, the white instrument at top left, is one of several science tools located on the end of the robotic arm aboard NASA’s Perseverance rover. The Mars rover’s left navcam took the images that make up this composite on March 2, 2021.
Called “adaptive sampling,” the software autonomously positions the instrument close to a rock target, then looks at PIXL’s scans of the target to find minerals worth examining more deeply. It’s all done in real time, without the rover talking to mission controllers back on Earth.
“We use PIXL’s AI to home in on key science,” said the instrument’s principal investigator, Abigail Allwood of JPL. “Without it, you’d see a hint of something interesting in the data and then need to rescan the rock to study it more. This lets PIXL reach a conclusion without humans examining the data.”
Data from Perseverance’s instruments, including PIXL, helps scientists determine when to drill a core of rock and seal it in a titanium metal tube so that it, along with other high-priority samples , could be brought to Earth for further study as part of NASA’s Mars Sample Return campaign .
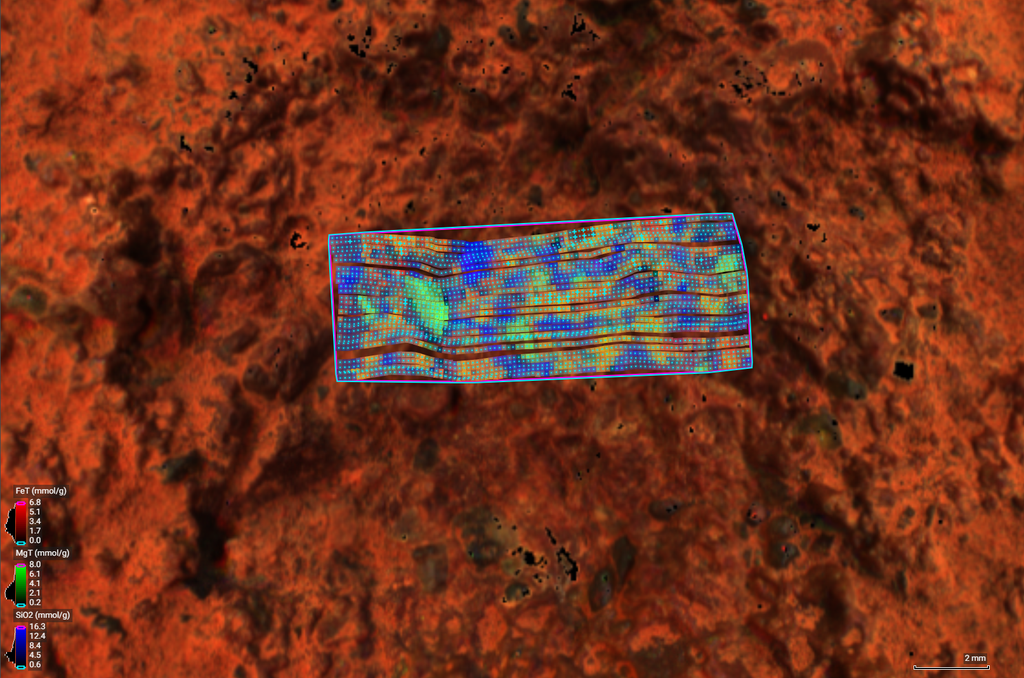
This image of a rock target nicknamed “Thunderbolt Peak” was created by NASA’s Perseverance Mars rover using PIXL, which determines the mineral composition of rocks by zapping them with X-rays. Each blue dot in the image represents a spot where an X-ray hit.
Adaptive sampling is not the only application of AI on Mars. About 2,300 miles (3,700 kilometers) from Perseverance is NASA’s Curiosity, which pioneered a form of AI that allows the rover to autonomously zap rocks with a laser based on their shape and color. Studying the gas that burns off after each laser zap reveals a rock’s chemical composition. Perseverance features this same ability, as well as a more advanced form of AI that enables it to navigate without specific direction from Earth. Both rovers still rely on dozens of engineers and scientists to plan each day’s set of hundreds of individual commands, but these digital smarts help both missions get more done in less time.
“The idea behind PIXL’s adaptive sampling is to help scientists find the needle within a haystack of data, freeing up time and energy for them to focus on other things,” said Peter Lawson, who led the implementation of adaptive sampling before retiring from JPL. “Ultimately, it helps us gather the best science more quickly.”
Using AI to Position PIXL
AI assists PIXL in two ways. First, it positions the instrument just right once the instrument is in the vicinity of a rock target. Located at the end of Perseverance’s robotic arm, the spectrometer sits on six tiny robotic legs, called a hexapod. PIXL’s camera repeatedly checks the distance between the instrument and a rock target to aid with positioning.
Never Miss a Discovery
Temperature swings on Mars are large enough that Perseverance’s arm will expand or contract a microscopic amount, which can throw off PIXL’s aim. The hexapod automatically adjusts the instrument to get it exceptionally close without coming into contact with the rock.
“We have to make adjustments on the scale of micrometers to get the accuracy we need,” Allwood said. “It gets close enough to the rock to raise the hairs on the back of an engineer’s neck.”
Making a Mineral Map
Once PIXL is in position, another AI system gets the chance to shine. PIXL scans a postage-stamp-size area of a rock, firing an X-ray beam thousands of times to create a grid of microscopic dots. Each dot reveals information about the chemical composition of the minerals present.
Minerals are crucial to answering key questions about Mars. Depending on the rock, scientists might be on the hunt for carbonates, which hide clues to how water may have formed the rock, or they may be looking for phosphates, which could have provided nutrients for microbes, if any were present in the Martian past.
There’s no way for scientists to know ahead of time which of the hundreds of X-ray zaps will turn up a particular mineral, but when the instrument finds certain minerals, it can automatically stop to gather more data — an action called a “long dwell.” As the system improves through machine learning, the list of minerals on which PIXL can focus with a long dwell is growing.
“PIXL is kind of a Swiss army knife in that it can be configured depending on what the scientists are looking for at a given time,” said JPL’s David Thompson, who helped develop the software. “Mars is a great place to test out AI since we have regular communications each day, giving us a chance to make tweaks along the way.”
When future missions travel deeper into the solar system, they’ll be out of contact longer than missions currently are on Mars. That’s why there is strong interest in developing more autonomy for missions as they rove and conduct science for the benefit of humanity.
More About the Mission
A key objective for Perseverance’s mission on Mars is astrobiology , including the search for signs of ancient microbial life. The rover will characterize the planet’s geology and past climate, pave the way for human exploration of the Red Planet, and be the first mission to collect and cache Martian rock and regolith (broken rock and dust).
Subsequent NASA missions, in cooperation with ESA (European Space Agency), would send spacecraft to Mars to collect these sealed samples from the surface and return them to Earth for in-depth analysis.
The Mars 2020 Perseverance mission is part of NASA’s Moon to Mars exploration approach, which includes Artemis missions to the Moon that will help prepare for human exploration of the Red Planet.
JPL, which is managed for NASA by Caltech in Pasadena, California, built and manages operations of the Perseverance rover.
For more about Perseverance:
mars.nasa.gov/mars2020/
News Media Contact
Andrew Good
Jet Propulsion Laboratory, Pasadena, Calif.
818-393-2433
Karen Fox / Alana Johnson
NASA Headquarters, Washington
202-358-1600 / 202-358-1501
[email protected] / [email protected]
Every print subscription comes with full digital access
Science News
Nasa’s perseverance rover finds its first possible hint of ancient life on mars.
The discovery builds the case for bringing pieces of Mars back to Earth for future study

Perseverance examined this Mars rock on July 21. The leopard spot–like features speckling the clay-colored part of the rock resemble structures in Earth rocks that are associated with life.
MSSS/JPL-Caltech/NASA
Share this:
By Lisa Grossman
July 25, 2024 at 7:52 pm
NASA’s Perseverance rover has bagged its first hint of ancient microbes on Mars.
“We’re not able to say that this is a sign of life,” says Perseverance deputy project scientist Katie Stack Morgan of NASA’s Jet Propulsion Lab in Pasadena, Calif. “But this is the most compelling sample we’ve found yet.”
The rover drilled up the sample on July 21 from a reddish rock, dubbed Cheyava Falls after a feature at the Grand Canyon. It is the first piece of Mars that Perseverance has examined that contains organic molecules, the building blocks of life, project scientist Ken Farley of Caltech reported July 25 at the 10th International Conference on Mars in Pasadena.
This isn’t the first sign of organics on Mars — the Curiosity rover detected organic molecules in a region called Gale Crater in 2014 (SN: 12/16/14) . But scientists have struggled to identify organics since Perseverance landed in an ancient dried-up lake called Jezero Crater in 2021 , says Stack Morgan ( SN: 2/17/21 ).
Adding to the excitement, the reddish rock is speckled with little white spots with black rims. “They look like a tricolored leopard spot,” Stack Morgan says.
Perseverance examined the spots with instruments that can identify their chemical contents and found that the rims contain iron phosphate molecules. On Earth, rings with similar texture and chemistry are associated with ancient microbial life . The chemical reactions that create the rings can be an energy source for microbes.
“They don’t require life, of course, and that’s an important caveat,” Stack Morgan says. “But based on our experience with similar things on Earth, there is a possibility that life could have been involved, and these could have a biological origin.”

The rock has other confusing features that muddy the picture of how it formed, Stack Morgan says. It is shot through with white veins of calcium sulfate. These veins are filled with millimeter-sized crystals of olivine, a mineral that forms from magma. The inclusion of both the spots and these volcanic features in the same rock is “a little bit mysterious,” Stack Morgan says, as they point to different origins. Figuring out how the rock formed could help tell how likely it is to have had the right conditions and temperatures to host biology.
Planetary scientist Paul Byrne thinks we should be circumspect about the finding.
“Could this truly be a biosignature? Yes. And if it is, then it really is the kind of society-altering discovery that the discovery of truly extraterrestrial life would be,” says Byrne, of Washington University in St. Louis. But it’s also possible that the spots came from something other than life, “in which case all this is is an interesting example of water-rock chemistry.”
The only way to find out for sure is to bring the rock home. A big part of Perseverance’s mission is to collect samples from interesting rocks for a future spacecraft to return to Earth, where they can be studied in more sophisticated laboratories than a rover can carry on its back. Perseverance has thrown everything it has at this rock already, Stack Morgan says.
But funding uncertainty has recently put the program, known as Mars Sample Return, on hold (SN: 5/8/24).
“With this sample, the rationale for MSR is strengthened even more, and should I hope motivate NASA to commit to pulling off this project sooner rather than later,” Byrne says.
Stack Morgan says the rover team is carrying on despite the budget uncertainty.
“We have a mission to carry out, and a job to do: collecting compelling samples,” Stack Morgan says. “It can only be our hope that the samples that we collect are compelling enough to justify the cost of Mars Sample Return. I think with this exciting sample, that really hits that home.”
More Stories from Science News on Planetary Science

Moonquakes are much more common than thought, Apollo data suggest

A planet needs to start with a lot of water to become like Earth

Jupiter’s Great Red Spot may be less than 200 years old

Sulfur was key to the first water on Earth

Venus might be as volcanically active as Earth

A weaker magnetic field may have paved the way for marine life to go big

Pluto’s heart-shaped basin might not hide an ocean after all

Our picture of habitability on Europa, a top contender for hosting life, is changing
Subscribers, enter your e-mail address for full access to the Science News archives and digital editions.
Not a subscriber? Become one now .
Information
- Author Services
Initiatives
You are accessing a machine-readable page. In order to be human-readable, please install an RSS reader.
All articles published by MDPI are made immediately available worldwide under an open access license. No special permission is required to reuse all or part of the article published by MDPI, including figures and tables. For articles published under an open access Creative Common CC BY license, any part of the article may be reused without permission provided that the original article is clearly cited. For more information, please refer to https://www.mdpi.com/openaccess .
Feature papers represent the most advanced research with significant potential for high impact in the field. A Feature Paper should be a substantial original Article that involves several techniques or approaches, provides an outlook for future research directions and describes possible research applications.
Feature papers are submitted upon individual invitation or recommendation by the scientific editors and must receive positive feedback from the reviewers.
Editor’s Choice articles are based on recommendations by the scientific editors of MDPI journals from around the world. Editors select a small number of articles recently published in the journal that they believe will be particularly interesting to readers, or important in the respective research area. The aim is to provide a snapshot of some of the most exciting work published in the various research areas of the journal.
Original Submission Date Received: .
- Active Journals
- Find a Journal
- Proceedings Series
- For Authors
- For Reviewers
- For Editors
- For Librarians
- For Publishers
- For Societies
- For Conference Organizers
- Open Access Policy
- Institutional Open Access Program
- Special Issues Guidelines
- Editorial Process
- Research and Publication Ethics
- Article Processing Charges
- Testimonials
- Preprints.org
- SciProfiles
- Encyclopedia
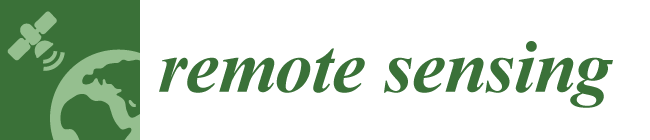
Article Menu

- Subscribe SciFeed
- Recommended Articles
- Google Scholar
- on Google Scholar
- Table of Contents
Find support for a specific problem in the support section of our website.
Please let us know what you think of our products and services.
Visit our dedicated information section to learn more about MDPI.
JSmol Viewer
Martian dust storms: reviews and perspective for the tianwen-3 mars sample return mission.

1. Introduction
2. martian dust storms, 2.1. basic properties of dust storms, 2.2. driving mechanisms of dust storms, 2.3. impacts of dust storms, 3. detection methods for dust storms, 3.1. historical views on dust storms, 3.2. orbiter multicolor imaging, 3.3. orbiter infrared spectroscopy, 3.4. landers/rovers monitoring, 4. dust storm monitoring and predicting for tianwen-3, 4.1. statistical predictions.
- The four zones are located in Utopia Planitia, Isidis Basin, Amazonis Planitia, and Chryse Planitia, respectively. Each zone covers a range of 18° in longitude and 15° in latitude, creating a 3 × 3 grid in the VDOD database;
- The VDOD data in the grid during MY 24–35 (12 MYs) are extracted from the database to form a subset for each region, resulting in 108 samples for each zone (=3 × 3 × 12);
- For each Martian day, the numbers of the six dust activity levels in the 108 samples are counted, and their probabilities are calculated;
- Since Martian dust storms are seasonal, we extend the date to MY 40 and 41 according to the orbit of Mars;
- Finally, the predicted results are presented in Figure 6 .
- Before the southern spring equinox ( L S < 180°), the dust activity remains weak, generally below the safety line (VDOD < 1.0), occasionally exceeding 1.0 but with very short duration;
- After the southern spring equinox ( L S > 180°), there is a significant increase in the probability of VDOD exceeding 1.0. The trend is particularly noticeable between L S of 200°~240° (i.e., 7 October to 11 December, 2029 in MY 40 and 25 August to 29 October, 2031 in MY 41), with the probability of VDOD exceeding 2.0 being greater than 30%. Dust activity generally peaks near L S of 210°~220°, and then begins to weaken and recover. During this period, it is important for operators to closely monitor the changing trend of dust activity;
- After the southern summer solstice ( L S > 270°), dust activity experiences a significant weakening but remains at an active level. As shown in Figure 6 a, just before L S = 270°, the probabilities for Level 2 and Level 3 are both about 50%. Subsequently, dust activity will recover to quiet conditions and begin another seasonal trend.
4.2. Dust Storm Monitoring for Tianwen-3
- Martian dust storm monitoring and early warning: Conducting large-field multicolor imaging of mid-low latitude dust storm activities on Mars to obtain information such as location, coverage and moving speed of dust storms on Mars.
- Spatial and temporal distribution of atmospheric ozone on Mars: Conducting large-field ultraviolet imaging of the column content of middle- and low-latitude ozone on Mars to obtain its spatial and temporal distributions. This will also improve the accuracy of dust storm identification in combination with water-ice cloud identification in visible light channels.
5. Conclusions
Author contributions, data availability statement, acknowledgments, conflicts of interest.
- Connerney, J.E.P.; Acuña, M.H.; Wasilewski, P.J.; Ness, N.F.; Rème, H.; Mazelle, C.; Vignes, D.; Lin, R.P.; Mitchell, D.L.; Cloutier, P.A. Magnetic lineations in the ancient crust of Mars. Science 1999 , 284 , 794–798. [ Google Scholar ] [ CrossRef ] [ PubMed ]
- Jakosky, B.M. Atmospheric loss to space and the history of water on Mars. Ann. Rev. Earth Planet. Sci. 2021 , 49 , 71–93. [ Google Scholar ] [ CrossRef ]
- Chaffin, M.S.; Kass, D.M.; Aoki, S.; Fedorova, A.A.; Deighan, J.; Connour, K.; Heavens, N.G.; Kleinböhl, A.; Jain, S.K.; Chaufray, J.-Y.; et al. Martian water loss to space enhanced by regional dust storms. Nat. Astronom. 2021 , 5 , 1036–1042. [ Google Scholar ] [ CrossRef ]
- Dubinin, E.; Fraenz, M.; Pätzold, M.; McFadden, J.; Halekas, J.S.; DiBraccio, G.A.; Connerney, J.E.P.; Eparvier, F.; Brain, D.; Jakosky, B.M.; et al. The effect of solar wind variations on the escape of oxygen ions from Mars through different channels: MAVEN observations. J. Geophys. Res. Space Phys. 2017 , 122 , 11285–11301. [ Google Scholar ] [ CrossRef ]
- Heavens, N.G.; Kleinböhl, A.; Chaffin, M.S.; Halekas, J.S.; Kass, D.M.; Hayne, P.O.; McCleese, D.J.; Piqueux, S.; Shirley, J.H.; Schofield, J.T. Hydrogen escape from Mars enhanced by deep convection in dust storms. Nat. Astronom. 2018 , 2 , 126–132. [ Google Scholar ] [ CrossRef ]
- Lillis, R.J.; Brain, D.A.; Bougher, S.W.; Leblanc, F.; Luhmann, J.G.; Jakosky, B.M.; Modolo, R.; Fox, J.; Deighan, J.; Fang, X.; et al. Characterizing atmospheric escape from Mars today and through time, with MAVEN. Space Sci. Rev. 2015 , 195 , 357–422. [ Google Scholar ] [ CrossRef ]
- Lundin, R.; Barabash, S.; Andersson, H.; Holmström, M.; Grigoriev, A.; Yamauchi, M.; Sauvaud, J.-A.; Fedorov, A.; Dudnik, E.; Thocaven, J.J.; et al. Solar wind-induced atmospheric erosion at Mars: First results from ASPERA-3 on Mars Express. Science 2004 , 305 , 1933–1936. [ Google Scholar ] [ CrossRef ]
- Ramstad, R.; Barabash, S.; Futaana, Y.; Nilsson, H.; Wang, X.-D.; Holmström, M. The Martian atmospheric ion escape rate dependence on solar wind and solar EUV conditions: 1. Seven years of Mars Express observations. J. Geophys. Res. Planets 2015 , 120 , 1298–1309. [ Google Scholar ] [ CrossRef ]
- Wei, Y.; Fraenz, M.; Dubinin, E.; Woch, J.; Lühr, H.; Wan, W.; Zong, Q.-G.; Zhang, T.L.; Pu, Z.Y.; Fu, S.Y.; et al. Enhanced atmospheric oxygen outflow on Earth and Mars driven by a corotating interaction region. J. Geophys. Res. 2012 , 117 , A03208. [ Google Scholar ] [ CrossRef ]
- Barlow, N. Mars: An Introduction to Its Interior, Surface and Atmosphere ; Cambridge University Press: New York, NY, USA, 2008; pp. 163–186. [ Google Scholar ]
- Leovy, C. Weather and climate on Mars. Nature 2001 , 412 , 245–249. [ Google Scholar ] [ CrossRef ]
- Shirley, J.H. Solar system dynamics and global-scale dust storms on Mars. Icarus 2015 , 251 , 128–144. [ Google Scholar ] [ CrossRef ]
- Keiffer, H.H.; Jakosky, B.M.; Snyder, C.W.; Matthews, M.S. Mars ; Univ. Ariz. Press: Tucson, AZ, USA, 1992. [ Google Scholar ]
- Haberle, R.M.; Clancy, R.T.; Forget, F.; Smith, M.D.; Zurek, R.W. The Atmosphere and Climate of Mars ; Cambridge University Press: New York, NY, USA, 2007. [ Google Scholar ]
- Encrenaz, T. The atmosphere of Mars as constrained by remote sensing. Space Sci. Rev. 2001 , 96 , 411–424. [ Google Scholar ] [ CrossRef ]
- Smith, M.D. Spacecraft observations of Martian atmosphere. Ann. Rev. Earth Planet. Sci. 2008 , 36 , 191–219. [ Google Scholar ] [ CrossRef ]
- Schmidt, F.; Way, M.J.; Costard, F.; Bouley, S.; Séjourné, A.; Aleinov, I. Circumpolar ocean stability on Mars 3 Gy ago. Proc. Natl. Acad. Sci. USA 2022 , 119 , e2112930118. [ Google Scholar ] [ CrossRef ] [ PubMed ]
- Wei, Y.; He, F.; Fan, K.; Rong, Z.; Wang, Y. Preliminary predictions of the dust storm activity at the landing site of China’s Zhurong Mars rover in 2022. Chin. Sci. Bull. 2022 , 67 , 1938–1944. (In Chinese) [ Google Scholar ] [ CrossRef ]
- Wang, Y.; Wei, Y.; Fan, K.; He, F.; Rong, Z.; Zhou, X.; Tan, N. The impact of dust storms on Mars surface rovers: Review and prospect. Chin. Sci. Bull. 2023 , 68 , 368–379. (In Chinese) [ Google Scholar ] [ CrossRef ]
- Rong, Z.; Wei, Y.; He, F.; Gao, J.; Fan, K.; Wang, Y.; Cao, L.; Yan, L.; Ren, Z.; Zhou, X.; et al. The orbit schemes to monitor Martian dust storms: Benefits to China’s future mars missions. Chin. Sci. Bull. 2023 , 68 , 716–728. (In Chinese) [ Google Scholar ] [ CrossRef ]
- James, P.B. Martian local dust storms. In Recent Advances in Planetary Meteorology ; Hunt, G., Ed.; Cambridge University Press: New York, NY, USA, 1985; pp. 85–100. [ Google Scholar ]
- Martin, L.J.; Zurek, R.W. An analysis of the history of dust activity on Mars. J. Geophys. Res. 1993 , 98 , 3221–3246. [ Google Scholar ] [ CrossRef ]
- Zurek, R.W.; Martin, L.J. Interannual variability of planet-encircling dust storms on Mars. J. Geophys. Res. 1993 , 98 , 3247–3259. [ Google Scholar ] [ CrossRef ]
- Fernández, W. Martian dust storms: A review. Earth Moon Planet. 1997 , 77 , 19–46. [ Google Scholar ] [ CrossRef ]
- Kahre, M.A.; Murphy, J.R.; Newman, C.E.; Wilson, R.J.; Cantor, B.A.; Lemmon, M.T.; Wolff, M.J. The Mars Dust Cycle. In The Atmosphere and Climate of Mars ; Haberle, R.M., Clancy, R.T., Forget, F., Smith, M.D., Zurek, R.W., Eds.; Cambridge University Press: New York, NY, USA, 2007; pp. 295–337. [ Google Scholar ]
- Malin, M.C.; Cantor, B.A. MRO MARCI Weather Report for the week of 27 December 2021–2 January 2022. Malin Space Science Systems Captioned Image Release, 2022, MSSS-603. Available online: https://www.msss.com/msss_images/2022/01/05/ (accessed on 9 March 2024).
- Malin, M.C.; Cantor, B.A.; Britton, A.W. MRO MARCI Weather Report for the week of 4 June 2018–10 June 2018, Malin Space Science Systems Captioned Image Release, 2018, MSSS-534. Available online: https://www.msss.com/msss_images/2018/06/13/ (accessed on 9 March 2024).
- Cantor, B.A.; James, P.B.; Caplinger, M.; Wolff, M.J. Martian dust storms: 1999 Mars Orbiter Camera observations. J. Geophys. Res. 2001 , 106 , 23653–23688. [ Google Scholar ] [ CrossRef ]
- Wang, H.; Richardson, M.I. The origin, evolution, and trajectory of large dust storms on Mars during Mars Year 24–30 (1999–2011). Icarus 2015 , 251 , 112–127. [ Google Scholar ] [ CrossRef ]
- Kulowski, L.; Wang, H.; Toigo, A.D. The seasonal and spatial distribution of textured dust storms observed by Mars Global Surveyor Mars Orbiter Camera. Adv. Space Res. 2017 , 59 , 715–721. [ Google Scholar ] [ CrossRef ]
- Guzewich, S.D.; Fedorova, A.A.; Kahre, M.A.; Toigo, A.D. Studies of the 2018/Mars Year 34 planet-encircling dust storm. J. Geophys. Res. Planets 2020 , 125 , e2020JE006700. [ Google Scholar ] [ CrossRef ]
- Wang, H.; Saidel, M.; Richardson, M.I.; Toigo, A.D.; Battalio, J.M. Martian dust storm distribution and annual cycle from Mars daily global map observations. Icarus 2023 , 394 , 115416. [ Google Scholar ] [ CrossRef ]
- Sánchez-Lavega, A.; del Río-Gaztelurrutia, T.; Hernández-Bernal, J.; Delcroix, M. The onset and growth of the 2018 Martian Global Dust Storm. Geophys. Res. Lett. 2019 , 46 , 6101–6108. [ Google Scholar ] [ CrossRef ]
- Kass, D.M.; Kleinböhl, A.; McCleese, D.J.; Schofield, J.T.; Smith, M.D. Interannual similarity in the Martian atmosphere during the dust storm season. Geophys. Res. Lett. 2016 , 43 , 6111–6118. [ Google Scholar ] [ CrossRef ]
- Montabone, L.; Forget, F.; Millour, E.; Wilson, R.J.; Lewis, S.R.; Cantor, B.; Kass, D.; Kleinböhl, A.; Lemmon, M.T.; Smith, M.D.; et al. Eight-year climatology of dust optical depth on Mars. Icarus 2015 , 251 , 65–95. [ Google Scholar ] [ CrossRef ]
- Montabone, L.; Spiga, A.; Kass, D.M.; Kleinböhl, A.; Forget, F.; Millour, E. Martian year 34 column dust climatology from Mars Climate Sounder observations: Reconstructed maps and model simulations. J. Geophys. Res. Planets 2020 , 125 , e2019JE006111. [ Google Scholar ] [ CrossRef ]
- Lemmon, M.T.; Guzewich, S.D.; McConnochie, T.; de Vicente-Retortillo, A.; Martínez, G.; Smith, M.D.; Bell, J.F., III; Wellington, D.; Jacob, S. Large dust aerosol sizes seen during the 2018 Martian global dust event by the Curiosity rover. Geophys. Res. Lett. 2019 , 46 , 9448–9456. [ Google Scholar ] [ CrossRef ]
- Chen-Chen, H.; Pérez-Hoyos, S.; Sánchez-Lavega, A. Dust particle size, shape and optical depth during the 2018/MY34 Martian global dust storm retrieved by MSL Curiosity rover Navigation Cameras. Icarus 2021 , 354 , 114021. [ Google Scholar ] [ CrossRef ]
- Clancy, R.T.; Wolff, M.J.; Christensen, P.R. Mars aerosol studies with the MGS TES emission phase function observations: Optical depths, particle sizes, and ice cloud types versus latitude and solar longitude. J. Geophys. Res. 2003 , 108 , 5098. [ Google Scholar ] [ CrossRef ]
- Wolff, M.J.; Clancy, R.T. Constraints on the size of Martian aerosols from thermal emission spectrometer observations. J. Geophys. Res. 2003 , 108 , 5097. [ Google Scholar ] [ CrossRef ]
- Vicente-Retortillo, Á.; Martínez, G.; Rennó, N.; Lemmon, M.T.; de la Torre Juarez, M. Determination of dust aerosol particle size at Gale crater using REMS UVS and Mastcam measurements. Geophys. Res. Lett. 2017 , 44 , 3502–3508. [ Google Scholar ] [ CrossRef ]
- Fedorova, A.A.; Montmessin, F.; Rodin, A.V.; Korablev, O.I.; Maattanen, A.; Maltagliati, L.; Bertaux, J.-L. Evidence for a bimodal size distribution for the suspended aerosol particles on Mars. Icarus 2014 , 231 , 239–260. [ Google Scholar ] [ CrossRef ]
- Bertaux, J.-L.; Korablev, O.; Perrier, S.; Quémerais, E.; Montmessin, F.; Leblanc, F.; Lebonnois, S.; Rannou, P.; Lefèvre, F.; Forget, F.; et al. SPICAM on Mars Express: Observing modes and overview of UV spectrometer data and scientific results. J. Geophys. Res. 2006 , 111 , E10S90. [ Google Scholar ] [ CrossRef ]
- Guzewich, S.D.; Tiogo, A.D.; Richardson, M.I.; Newman, C.E.; Talaat, E.R.; Waugh, D.W.; McConnochie, T.H. The impact of a realistic vertical dust distribution on the simulation of the Martian General Circulation. J. Geophys. Res. Planet. 2013 , 118 , 980–993. [ Google Scholar ] [ CrossRef ]
- Medvedev, A.S.; Yiğit, E.; Kuroda, T.; Hartogh, P. General circulation modeling of the Martian upper atmosphere during global dust storms. J. Geophys. Res. Planet. 2013 , 118 , 2234–2246. [ Google Scholar ] [ CrossRef ]
- Wang, Y.; Chow, K.-C.; Xiao, J.; Wang, C.-F. Effect of dust particle size on the climate of Mars. Planet. Space Sci. 2021 , 208 , 105346. [ Google Scholar ] [ CrossRef ]
- Hanel, R.; Conrath, B.; Hovis, W.; Kunde, V.; Lowman, P.; Maguire, W.; Pearl, J.; Pirraglia, J.; Prabhakara, C.; Schlachman, B.; et al. Investigation of the Martian environment by infrared spectroscopy on Mariner 9. Icarus 1972 , 17 , 423–442. [ Google Scholar ] [ CrossRef ]
- Leovy, C.B.; Zurek, R.W.; Pollack, J.B. Mechanisms for Mars dust storms. J. Atmos. Sci. 1973 , 30 , 749–762. [ Google Scholar ] [ CrossRef ]
- Gierasch, P.J.; Goody, R.M. A model of a Martian great dust storm. J. Atmos. Sci. 1973 , 30 , 169–179. [ Google Scholar ] [ CrossRef ]
- Leovy, C.B.; Briggs, G.A.; Young, A.T.; Smith, B.A.; Pollack, J.B.; Shipley, E.N.; Wildey, R.L. The Martian atmosphere: Mariner 9 television experiment progress report. Icarus 1972 , 17 , 373–393. [ Google Scholar ] [ CrossRef ]
- Cross, C.A. The heat balance of the Martian polar caps. Icarus 1971 , 15 , 110–114. [ Google Scholar ] [ CrossRef ]
- Ryan, J.A.; Sharman, R.D.; Lucich, R.D. Local Mars dust storm generation mechanism. Geophys. Res. Lett. 1981 , 8 , 899–901. [ Google Scholar ] [ CrossRef ]
- Ryan, J.A.; Lucich, R.D. Possible dust devils, vortices on Mars. J. Geophys. Res. 1983 , 88 , 11005–11011. [ Google Scholar ] [ CrossRef ]
- Hess, S.L. Martian winds and dust clouds. Planet. Space Sci. 1973 , 21 , 1549–1557. [ Google Scholar ] [ CrossRef ]
- Newman, C.E.; Lewis, S.R.; Read, S.R.; Forget, F. Modeling the Martian dust cycle, 1, Representations of dust transport processes. J. Geophys. Res. 2002 , 107 , 5123. [ Google Scholar ] [ CrossRef ]
- Newman, C.E.; Lewis, S.R.; Read, S.R.; Forget, F. Modeling the Martian dust cycle, 2, Multiannual radiatively active dust transport simulations. J. Geophys. Res. 2002 , 107 , 5124. [ Google Scholar ] [ CrossRef ]
- Basu, S.; Wilson, J.; Richardson, M.; Ingersoll, A. Simulation of spontaneous and variable global dust storms with the GFDL Mars GCM. J. Geophys. Res. 2006 , 111 , E09004. [ Google Scholar ] [ CrossRef ]
- Kahre, M.A.; Murphy, J.R.; Haberle, R.M. Modeling the Martian dust cycle and surface dust reservoirs with the NASA Ames general circulation model. J. Geophys. Res. 2006 , 11 , E06008. [ Google Scholar ] [ CrossRef ]
- Xiao, J.; Chow, K.C.; Chan, K. Dynamical processes of dust lifting in the northern mid-latitude region of Mars during the dust storm season. Icarus 2019 , 317 , 94–103. [ Google Scholar ] [ CrossRef ]
- Forget, F.; Hourdin, F.; Fournier, R.; Hourdin, C.; Talagrand, O.; Collins, M.; Lewis, S.R.; Read, P.L.; Huot, J.P. Improved general circulation model for the Martian atmosphere from the surface to above 80 km. J. Geophys. Res. 1999 , 104 , 24155–24175. [ Google Scholar ] [ CrossRef ]
- Petrosyan, A.; Galperin, B.; Larsen, S.E.; Lewis, S.R.; Määttänen, A.; Read, P.L.; Renno, N.; Rogberg, L.P.H.T.; Savijärvi, H.; Siili, T.; et al. The Martian atmospheric boundary layer. Rev. Geophys. 2011 , 49 , RG3005. [ Google Scholar ] [ CrossRef ]
- Shirley, J.H.; Mischna, M.A. Orbit-spin coupling and the interannual variability of global-scale dust storm occurrence on Mars. Planet. Space Sci. 2017 , 139 , 37–50. [ Google Scholar ] [ CrossRef ]
- Mischna, M.A.; Shirley, J.H. Numerical modeling of orbit-spin coupling accelerations in a Mars general circulation model: Implications for global dust storm activity. Planet. Space Sci. 2017 , 141 , 45–72. [ Google Scholar ] [ CrossRef ]
- Newman, C.E.; Lee, C.; Mischna, M.A.; Richardson, M.I.; Shirley, J.H. An initial assessment of the impact of postulated orbit-spin coupling on Mars dust storm variability in fully interactive dust simulations. Icarus 2019 , 317 , 649–668. [ Google Scholar ] [ CrossRef ]
- Shirley, J.H.; McKim, R.J.; Battalio, J.M.; Kass, D.M. Orbit-Spin Coupling and the Triggering of the Martian Planet-Encircling Dust Storm of 2018. J. Geophys. Res. Planets 2020 , 125 , e2019JE006077. [ Google Scholar ] [ CrossRef ]
- Bertrand, T.; Wilson, R.J.; Kahre, M.A.; Urata, R.; Kling, A. Simulation of the 2018 global dust storm on Mars using the NASA Ames Mars GCM: A multitracer approach. J. Geophys. Res. Planets 2020 , 125 , e2019JE006122. [ Google Scholar ] [ CrossRef ]
- Wu, Z.; Li, T.; Heavens, N.G.; Newman, C.E.; Richardson, M.I.; Yang, C.; Li, J.; Cui, J. Earth-like thermal and dynamical coupling processes in the Martian climate system. Earth Sci. Rev. 2022 , 229 , 104023. [ Google Scholar ] [ CrossRef ]
- Zhou, X.; Wei, Y.; Wu, Z.; Ren, Z.; Tan, N.; Fan, S.; He, F.; Rong, Z.; Yan, L.; Wang, Y.; et al. Martian whole atmosphere model and dust activities: Review and prospect. Chin. Sci. Bull. 2024 , 69 , 1058–1067. (In Chinese) [ Google Scholar ] [ CrossRef ]
- Fenton, L.K.; Geissler, P.E.; Haberle, R.M. Global warming and climate forcing by recent albedo changes on Mars. Nature 2007 , 446 , 646–649. [ Google Scholar ] [ CrossRef ]
- Kass, D.M.; Schofield, J.T.; Kleinböhl, A.; McCleese, D.J.; Heavens, N.G.; Shirley, J.H.; Steele, L.J. Mars Climate Sounder observation of Mars’ 2018 global dust storm. Geophys. Res. Lett. 2020 , 47 , e2019GL083931. [ Google Scholar ] [ CrossRef ]
- Guzewich, S.D.; Lemmon, M.; Smith, C.L.; Martínez, G.; de Vicente-Retortillo, Á.; Newman, C.E.; Baker, M.; Campbell, C.; Cooper, B.; Gómez-Elvira, J.; et al. Mars Science Laboratory observations of the 2018/Mars year 34 global dust storm. Geophys. Res. Lett. 2019 , 46 , 71–79. [ Google Scholar ] [ CrossRef ]
- Haberle, R.M.; Pollack, J.B.; Barnes, J.R.; Zurek, R.W.; Leovy, C.B.; Murphy, J.R.; Lee, H.; Schaeffer, J. Mars atmospheric dynamics as simulated by the NASA Ames General Circulation Model: 1. The zonal-mean circulation. J. Geophys. Res. 1993 , 98 , 3093–3123. [ Google Scholar ] [ CrossRef ]
- Wu, Z.; Li, T.; Zhang, X.; Li, J.; Cui, J. Dust tides and rapid meridional motions in the Martian atmosphere during major dust storms. Nat. Commun. 2020 , 11 , 614. [ Google Scholar ] [ CrossRef ] [ PubMed ]
- Zhao, Y.; Zhong, L.; Yuan, R.; Zhao, C.; Li, R.; Wang, Y.; Lian, Y.; Richardson, M. Simulation of Martian dust effects on polar CO 2 ice caps and atmospheric circulation using the MarsWRF model. J. Geophys. Res. Planet. 2021 , 126 , e2021JE006937. [ Google Scholar ] [ CrossRef ]
- Melnik, O.; Parrot, M. Electrostatic discharge in Martian dust storms. J. Geophys. Res. 1998 , 103 , 29107–29117. [ Google Scholar ] [ CrossRef ]
- Farrell, W.M.; Kaiser, M.L.; Desch, M.D.; Houser, J.G.; Cummer, S.A.; Wilt, D.M.; Landis, G.A. Detecting electrical activity from Martian dust storms. J. Geophys. Res. 1999 , 104 , 3795–3801. [ Google Scholar ] [ CrossRef ]
- Krauss, C.E.; Horanyi, M.; Robertson, S. Experimental evidence for electrostatic discharging of dust near the surface of Mars. New J. Phys. 2003 , 5 , 70. [ Google Scholar ] [ CrossRef ]
- Merrison, J.; Jensen, J.; Kinch, K.; Mugford, R.; Nørnberg, P. The electrical properties of Mars analogue dust. Planet Space Sci. 2004 , 52 , 279–290. [ Google Scholar ] [ CrossRef ]
- Michael, M.; Tripathi, S.N. Effect of charging of aerosols in the lower atmosphere of Mars during the dust storm of 2001. Planet Space Sci. 2008 , 56 , 1696–1702. [ Google Scholar ] [ CrossRef ]
- Haider, S.A.; Sheel, V.; Smith, M.D.; Maguire, W.C.; Molina-Cuberos, G.J. Effect of dust storms on the D region of the Martian ionosphere: Atmospheric electricity. J. Geophys. Res. Space Phys. 2010 , 115 , A12336. [ Google Scholar ] [ CrossRef ]
- McDunn, T.L.; Bougher, S.W.; Murphy, J.; Smith, M.D.; Forget, F.; Bertaux, J.-L.; Montmessin, F. Simulating the density and thermal structure of the middle atmosphere (80–130 km) of Mars using the MGCM–MTGCM: A comparison with MEX/SPICAM observations. Icarus 2010 , 206 , 5–17. [ Google Scholar ] [ CrossRef ]
- Chaufray, J.Y.; Chaffin, M.S.; Deighan, J.; Jain, S.; Schneider, N.; Mayyasi, M.; Jakosky, B. Effect of the 2018 Martian global dust storm on the CO2 density in the lower nightside thermosphere observed from MAVEN/IUVS Lyman-alpha absorption. Geophys. Res. Lett. 2020 , 47 , e2019GL082889. [ Google Scholar ] [ CrossRef ]
- Fang, X.; Ma, Y.; Lee, Y.; Bougher, S.; Liu, G.; Benna, M.; Mahaffy, P.; Montabone, L.; Pawlowski, D.; Dong, C.; et al. Mars dust storm effects in the ionosphere and magnetosphere and implications for atmospheric carbon loss. J. Geophys. Res. Space Phys. 2020 , 125 , e2019JA026838. [ Google Scholar ] [ CrossRef ]
- Niu, D.D.; Cui, J.; Wu, S.Q.; Gu, H.; Cao, Y.-T.; Wu, Z.-P.; Wu, X.-S.; Zhong, J.-H.; Wu, M.-Y.; Wei, Y.; et al. Species-dependent response of the Martian ionosphere to the 2018 global dust event. J. Geophys. Res. Planets 2021 , 126 , e2020JE006679. [ Google Scholar ] [ CrossRef ]
- Mukundan, V.; Thampi, S.V.; Bhardwaj, A.; Fang, X. Impact of the 2018 Mars global dust storm on the ionospheric peak: A study using a photochemical model. J. Geophys. Res. Planets 2021 , 126 , e2021JE006823. [ Google Scholar ] [ CrossRef ]
- Stone, S.W.; Yelle, R.V.; Benna, M.; Lo, D.Y.; Elrod, M.K.; Mahaffy, P.R. Hydrogen escape from Mars is driven by seasonal and dust storm transport of water. Science 2020 , 370 , 824–831. [ Google Scholar ] [ CrossRef ]
- Clancy, R.T.; Nair, H. Annual (perihelion-aphelion) cycles in the photochemical behavior of the global Mars atmosphere. J. Geophys. Res. Planets 1996 , 101 , 12785–12790. [ Google Scholar ] [ CrossRef ]
- Clancy, R.T.; Wolff, M.J.; Lefèvre, F.; Cantor, B.A.; Malin, M.C.; Smith, M.D. Daily global mapping of Mars ozone column abundances with MARCI UV band imaging. Icarus 2016 , 266 , 112–133. [ Google Scholar ] [ CrossRef ]
- Hvidberg, C.S.; Fishbaugh, K.E.; Winstrup, M.; Svensson, A.; Byrne, S.; Herkenhoff, K.E. Reading the climate record of the Martian polar layered deposits. Icarus 2012 , 221 , 405–419. [ Google Scholar ] [ CrossRef ]
- Colaprete, A.; Barnes, J.; Haberle, R.; Hollingsworth, J.L.; Kieffer, H.H.; Titus, T.N. Albedo of the south pole on Mars determined by topographic forcing of atmosphere dynamics. Nature 2005 , 435 , 184–188. [ Google Scholar ] [ CrossRef ] [ PubMed ]
- Golombek, M.P.; Warner, N.H.; Ganti, V.; Lamb, M.P.; Parker, T.J.; Fergason, R.L.; Sullivan, R. Small crater modification on Meridiani Planum and implications for erosion rates and climate change on Mars. J. Geophys. Res. Planets 2014 , 119 , 2522–2547. [ Google Scholar ] [ CrossRef ]
- Farley, K.A.; Malespin, C.; Mahaffy, P.; Grotzinger, J.P.; Vasconcelos, P.M.; Milliken, R.E.; Malin, M.; Edgett, K.S.; Pavlov, A.A.; Hurowitz, J.A.; et al. In Situ Radiometric and Exposure Age Dating of the Martian Surface. Science 2013 , 343 , 1247166. [ Google Scholar ] [ CrossRef ] [ PubMed ]
- Vasavada, A.R.; Chen, A.; Barnes, J.R.; Burkhart, P.D.; Cantor, B.A.; Dwyer-Cianciolo, A.M.; Fergason, R.L.; Hinson, D.P.; Justh, H.L.; Kass, D.M.; et al. Assessment of environments for mars science laboratory entry, descent, and surface operations. Space Sci. Rev. 2012 , 170 , 793–835. [ Google Scholar ] [ CrossRef ]
- Team, R. Characterization of the Martian Surface Deposits by the Mars Pathfinder Rover, Sojourner. Science 1997 , 278 , 1765–1767. [ Google Scholar ] [ CrossRef ]
- Rieder, R.T.; Economou, T.E.; Wänke, H.; Turkevich, A.; Crisp, J.; Brückner, J.; Dreibus, G.; McSween, H.Y., Jr. The Chemical Composition of Martian Soil and Rocks Returned by the Mobile Alpha Proton X-ray Spectrometer: Preliminary Results from the X-ray Mode. Science 1998 , 278 , 1771–1774. [ Google Scholar ] [ CrossRef ]
- Lorenz, R.D.; Martínez, G.M.; Spiga, A.; Vicente-Retortillo, A.; Newman, C.E.; Murdoch, N.; Forget, F.; Millour, E.; Pierron, T. Lander and rover histories of dust accumulation on and removal from solar arrays on Mars. Planet. Space Sci. 2021 , 207 , 105337. [ Google Scholar ] [ CrossRef ]
- Landis, G.A.; Jenkins, P.P. Measurement of the settling rate of atmospheric dust on Mars by the MAE instrument on Mars Pathfinder. J. Geophys. Res. 2000 , 105 , 1855–1857. [ Google Scholar ] [ CrossRef ]
- Crisp, D.; Pathare, A.; Ewell, R.C. The performance of gallium arsenide/germanium solar cells at the Martian surface. Acta Astronaut. 2004 , 54 , 83–101. [ Google Scholar ] [ CrossRef ]
- Staab, M.S.; Herman, J.A.; Reich, K.; Reich, K.; Sridhar, V.; Nelson, R.W. MER Opportunity dust-storm recovery operations and implications for future Mars surface missions. In Proceedings of the 2020 IEEE Aerospace Conference, Big Sky, MT, USA, 7–14 March 2020. [ Google Scholar ]
- Yen, A.; Gellert, R.; Schröder, C.; Morris, R.V.; Bell, J.F., III; Knudson, A.T.; Clark, B.C.; Ming, D.M.; Crisp, J.A.; Arvidson, R.E.; et al. An integrated view of the chemistry and mineralogy of Martian soils. Nature 2005 , 436 , 49–54. [ Google Scholar ] [ CrossRef ] [ PubMed ]
- Slipher, E.C. Mars—The Photographic Story ; Northland Press: Flagstaff, AZ, USA, 1962. [ Google Scholar ]
- Horn, D.; McAfee, J.M.; Winer, A.M.; Herr, K.C.; Pimentel, G.C. The composition of the Martian atmosphere: Minor constituents. Icarus 1972 , 16 , 543–556. [ Google Scholar ] [ CrossRef ]
- Cimino, G.; Clavin, W.M. Calibration and analysis of Mariner 7 infrared spectra. Bull. Am. Astron. Soc. 1996 , 28 , 1068. [ Google Scholar ]
- Hord, C.W.; Barth, C.A.; Stewart, A.I.; Lane, A.L. Mariner 9 ultraviolet spectrometer experiment: Photometry and topography of Mars. Icarus 1972 , 17 , 433–456. [ Google Scholar ] [ CrossRef ]
- Anderson, E.; Loevy, C. Mariner 9 television limb observations of dust and ice hazes on Mars. J. Atmos. Sci. 1978 , 35 , 723. [ Google Scholar ] [ CrossRef ]
- Martin, T.Z.; Richardson, M.I. New dust opacity mapping from Viking infrared thermal mapper data. J. Geophys. Res. 1993 , 98 , 10941–10949. [ Google Scholar ] [ CrossRef ]
- Biggs, G.A.; Baum, W.A.; Barnes, J. Viking Orbiter imaging observations of dust in the martian atmosphere. J. Geophys. Res. 1979 , 84 , 2795. [ Google Scholar ] [ CrossRef ]
- Malin, M.C.; Danielson, G.E.; Ravine, M.A.; Soulanille, T.A. Design and development of the Mars Observer Camera. Int. J. Imaging Syst. Technol. 1991 , 3 , 76–91. [ Google Scholar ] [ CrossRef ]
- Malin, M.C.; Danielson, G.E.; Ingersoll, A.P.; Masursky, H.; Veverka, J.; Ravine, M.A.; Soulanille, T.A. Mars Observer Camera. J. Geophys. Res. 1992 , 97 , 7699–7718. [ Google Scholar ] [ CrossRef ]
- Christensen, P.R.; Bandfield, J.L.; Hamilton, V.E.; Ruff, S.W.; Kieffer, H.H.; Titus, T.N.; Malin, M.C.; Morris, R.V.; Lane, M.D.; Clark, R.L.; et al. Mars Global Surveyor Thermal Emission Spectrometer experiment: Investigation description and surface science results. J. Geophys. Res. 2001 , 106 , 23823–23871. [ Google Scholar ] [ CrossRef ]
- Tomasko, M.G.; Doose, L.R.; Lemmon, M.; Smith, P.H.; Wegryn, E. Properties of dust in Martian atmosphere from the Imager on Mars Pathfinder. J. Geophys. Res. 1999 , 104 , 8987–9007. [ Google Scholar ] [ CrossRef ]
- Smith, P.H.; Lemmon, M. Opacity of the Martian atmosphere measured by the Imager for Mars Pathfinder. J. Geophys. Res. 1999 , 104 , 8975–8985. [ Google Scholar ] [ CrossRef ]
- Christensen, P.R.; Jakosky, B.M.; Kieffer, H.H.; Malin, M.C.; McSween, H.Y., Jr.; Nealson, K.; Mehall, G.L.; Silverman, S.H.; Ferry, S.; Caplinger, M.; et al. The Thermal Emission Imaging System (THEMIS) for the Mars 2001 Odyssey mission. Space Sci. Rev. 2004 , 110 , 85–130. [ Google Scholar ] [ CrossRef ]
- Määttänen, A.; Listowski, C.; Montmessin, F.; Maltagliati, L.; Reberac, A.; Joly, L.; Bertaux, L.J. A complete climatology of the aerosol vertical distribution on Mars from MEx/SPICAM UV solar occultations. Icarus 2013 , 223 , 892–941. [ Google Scholar ] [ CrossRef ]
- Bibring, J.-P.; Soufflot, A.; Berthé, M.; Langevin, Y.; Gondet, B.; Drossart, P.; Bouyé, M.; Combes, M.; Puget, P.; Semery, A.; et al. OMEGA: Observatoire pour la Mineralogie, l’Eau, les Glaces et l’Activité. Eur. Space Agency Spec. Pub. 2004 , 1240 , 37. [ Google Scholar ]
- Gwinner, K.; Jaumann, R.; Hauber, E.; Hoffmann, H.; Heipke, C.; Oberst, J.; Neukum, G.; Ansan, V.; Bostelmann, J.; Dumke, A.; et al. The High Resolution Stereo Camera (HRSC) of Mars Express and its approach to science analysis and mapping for Mars and its satellites. Planet. Space Sci. 2016 , 126 , 93–138. [ Google Scholar ] [ CrossRef ]
- Lemmon, M.T.; Wolff, M.J.; Bell, J.F., III; Smith, M.D.; Cantor, B.A.; Smith, P.H. Dust aerosol, clouds, and the atmospheric optical depth record over 5 Mars years of the Mars Exploration Rover mission. Icarus 2015 , 251 , 96–111. [ Google Scholar ] [ CrossRef ]
- Bell, J.F., III; Squyres, S.W.; Herkenhoff, K.E.; Maki, J.N.; Arneson, H.M.; Brown, D.; Collins, S.A.; Dingizian, A.; Elliot, S.T.; Hagerott, E.C.; et al. Mars Exploration Rover Athena Panoramic Camera (Pancam) investigation. J. Geophys. Res. Planets 2003 , 108 , 8063. [ Google Scholar ] [ CrossRef ]
- Chistensen, P.R.; Mehall, G.L.; Silverman, S.H.; Anwar, S.; Cannon, G.; Gorelick, N.; Kheen, R.; Tourville, T.; Bates, D.; Ferry, S.; et al. Miniature Thermal Emission Spectrometer for the Mars Exploration Rovers. J. Geophys. Res. Planets 2003 , 108 , 8064. [ Google Scholar ]
- Bell, J.F., III; Wolff, M.J.; Malin, M.C.; Calvin, W.M.; Cantor, B.A.; Caplinger, M.A.; Clancy, R.T.; Edgett, K.S.; Edwards, L.J.; Fahle, J.; et al. Mars Reconnaissance Orbiter Mars Color Imager (MARCI): Instrument description, calibration, and performance. J. Geophys. Res. Planets 2009 , 114 , E08S92. [ Google Scholar ] [ CrossRef ]
- Murchie, S.; Arvidson, R.; Bedini, P.; Beisser, K.; Bibring, J.-P.; Bishop, J.; Boldt, J.; Cavender, P.; Choo, T.; Clancy, R.T.; et al. Compact Reconnaissance Imaging Spectrometer for Mars (CRISM) on Mars Reconnaissance Orbiter (MRO). J. Geophys. Res. Planets 2007 , 112 , E05S03. [ Google Scholar ] [ CrossRef ]
- McCleese, D.J.; Schofield, J.T.; Taylor, F.W.; Calcutt, S.B.; Foote, M.C.; Kass, D.M.; Leovy, C.B.; Paige, D.A.; Read, P.L.; Zurek, R.W. Mars Climate Sounder: An investigation of thermal and water vapor structure, dust and condensate distributions in the atmosphere, and energy balance of the polar regions. J. Geophys. Res. Planets 2007 , 112 , E05S06. [ Google Scholar ] [ CrossRef ]
- Smith, P.H.; Tamppari, L.; Arvidson, R.E.; Bass, D.; Blaney, D.; Boynton, W.; Carswell, A.; Catling, D.; Clark, B.; Duck, T.; et al. Introduction to special section on the Phoenix Mission: Landing Site Characterization Experiments, Mission Overviews, and Expected Science. J. Geophys. Res. Planets 2008 , 113 , E00A18. [ Google Scholar ] [ CrossRef ]
- Grotzinger, J.P.; Crisp, J.; Vasavada, A.R.; Anderson, R.C.; Baker, C.J.; Barry, R.; Blake, D.F.; Conrad, P.; Edgett, K.S.; Ferdowski, B.; et al. Mars Science Laboratory mission and science investigation. Space Sci. Rev. 2012 , 170 , 5–56. [ Google Scholar ] [ CrossRef ]
- Vandaele, A.C.; Neefs, E.; Drummond, R.; Thomas, I.R.; Daerden, F.; Lopez-Moreno, J.-J.; Rodriguez, J.; Patel, M.R.; Bellucci, G.; Allen, M.; et al. Science objectives and performances of NOMAD, a spectrometer suite for the ExoMars TGO mission. Planet. Space Sci. 2015 , 119 , 233–249. [ Google Scholar ] [ CrossRef ]
- Thomas, N.; Cremonese, G.; Ziethe, R.; Gerber, M.; Brändli, M.; Bruno, G.; Erismann, M.; Gambicorti, L.; Gerber, T.; Ghose, K.; et al. The Colour and Stereo Surface Imaging System (CaSSIS) for the ExoMars Trace Gas Orbiter. Space Sci. Rev. 2017 , 212 , 1897–1944. [ Google Scholar ] [ CrossRef ]
- Korablev, O.; Montmessin, F.; Trokhimovskiy, A.; Fedorova, A.A.; Shakun, A.V.; Grigoriev, A.V.; Moshkin, B.E.; Ignatiev, N.I.; Forget, F.; Lefèvre, F.; et al. The Atmospheric Chemistry Suite (ACS) of Three Spectrometers for the ExoMars 2016 Trace Gas Orbiter. Space Sci. Rev. 2018 , 214 , 7. [ Google Scholar ] [ CrossRef ]
- Maki, J.N.; Golombek, M.; Deen, R.; Abarca, H.; Sorice, C.; Goodsall, T.; Schwochert, M.; Lemmon, M.; Trebi-Ollennu, A.; Banerdt, W.B. The Color Cameras on the InSight Lander. Space Sci. Rev. 2018 , 214 , 105. [ Google Scholar ] [ CrossRef ]
- Banfield, D.; Rodriguez-Manfredi, J.A.; Russell, C.T.; Rowe, K.M.; Leneman, D.; Lai, H.R.; Cruce, P.R.; Means, J.D.; Johnson, C.L.; Mittelholz, A.; et al. Insight Auxiliary Payload Sensor Suite (APSS). Space Sci. Rev. 2019 , 215 , 4. [ Google Scholar ] [ CrossRef ]
- Bell, J.F., III; Maki, J.N.; Mehall, G.L.; Ravine, M.A.; Caplinger, M.A.; Bailey, Z.J.; Brylow, S.; Schaffner, J.A.; Kinch, K.M.; Madsen, M.B.; et al. The Mars 2020 Perseverance Rover Mast Camera Zoom (Mastcam-Z) Multispectral, Stereoscopic Imaging Investigation. Space Sci. Rev. 2021 , 217 , 24. [ Google Scholar ] [ CrossRef ]
- Maurice, S.; Wiens, R.C.; Bernardi, P.; Caïs, P.; Robinson, S.; Nelson, T.; Gasnault, O.; Reess, J.-M.; Deleuze, M.; Rull, F.; et al. The SuperCam Instrument Suite on the Mars 2020 Rover: Science Objectives and Mast-Unit Description. Space Sci. Rev. 2021 , 217 , 47. [ Google Scholar ] [ CrossRef ]
- Rodriguez-Manfredi, J.A.; de la Torre Juárez, M.; Alonso, A.; Apéstigue, V.; Arruego, I.; Atienza, T.; Banfield, D.; Boland, J.; Carrera, M.A.; Castañer, L.; et al. The Mars Environmental Dynamics Analyzer, MEDA. A Suite of Environmental Sensors for the Mars 2020 Mission. Space Sci. Rev. 2021 , 217 , 48. [ Google Scholar ] [ CrossRef ] [ PubMed ]
- Jones, A.R.; Wolff, M.; Alshamsi, M.; Osterloo, M.; Bay, P.; Brennan, N.; Bryant, K.; Castleman, Z.; Curtin, A.; DeVito, E.; et al. The Emirates Exploration Imager (EXI) Instrument on the Emirates Mars Mission (EMM) Hope Mission. Space Sci. Rev. 2021 , 217 , 81. [ Google Scholar ] [ CrossRef ]
- Meng, Q.; Wang, D.; Wang, X.; Li, W.; Yang, X.; Yan, D.; Li, Y.; Cao, Z.; Ji, Q.; Sun, T.; et al. High Resolution Imaging Camera (HiRiC) on China’s first Mars exploration Tianwen-1 mission. Space Sci. Rev. 2021 , 217 , 42. [ Google Scholar ] [ CrossRef ]
- Yu, G.B.; Liu, E.H.; Liu, G.L.; Zhou, L.; Zeng, J.Z.; Chen, Y.P.; Zhou, X.D.; Zhao, R.J.; Zhu, S.Y. Moderate Resolution Imaging Camera (MoRIC) of China’s First Mars Mission Tianwen-1. Earth Planet. Phys. 2020 , 4 , 364–370. [ Google Scholar ] [ CrossRef ]
- Yang, J.-F.; Liu, D.-W.; Xue, B.; Lyu, J.; Liu, J.-J.; Li, F.; Ren, X.; Ge, W.; Liu, B.; Ma, X.-L.; et al. Design and ground verification for Multispectral Camera on the Mars Tianwen-1 Rover. Space Sci. Rev. 2022 , 218 , 19. [ Google Scholar ] [ CrossRef ]
- Li, B.; Yue, Z.; Qu, S.; Yao, P.; Fu, X.; Ling, Z.; Chen, S. Spatio-Temporal Analysis of Dust Storm Activity in Chryse Planitia Using MGS-MOC Observations from Mars Years 24–28. Universe 2021 , 7 , 433. [ Google Scholar ] [ CrossRef ]
- Guha, B.K.; Gebhardt, C.; Young, R.M.B.; Wolff, M.J.; Montabone, L. Seasonal and diurnal variations of dust storms in Martian year 36 based on the EMM-EXI database. J. Geophys. Res. Planets 2024 , 129 , e2023JE008156. [ Google Scholar ] [ CrossRef ]
- Wang, H.; Ingersoll, A.P. Martian clouds observed by Mars Global Surveyor Mars Orbiter Camera. J. Geophys. Res. 2002 , 107 , 5078. [ Google Scholar ] [ CrossRef ]
- Battalio, M.; Wang, H. The Mars Dust Activity Database (MDAD): A comprehensive statistical study of dust storm sequences. Icarus 2021 , 354 , 114059. [ Google Scholar ] [ CrossRef ]
- Wu, B.; Dong, J.; Wang, Y.; Rao, W.; Sun, Z.Z.; Li, Z.; Tan, Z.; Chen, Z.; Wang, C.; Liu, W.C.; et al. Landing Site Selection and Characterization of Tianwen-1 (Zhurong Rover) on Mars. J. Geophys. Res. Planets 2022 , 127 , e2021JE007137. [ Google Scholar ] [ CrossRef ]
- Shirley, J.H.; McConnochie, T.H.; Kass, D.M.; Kleinböhl, A.; Schofield, J.T.; Heavens, N.G.; McCleese, D.J.; Benson, J.; Hinson, D.P.; Bandfield, J.L. Temperature and aerosol opacities of the Mars atmosphere at aphelion: Validation and inter-comparison of limb sounding profiles from MRO/MCS and MGS/TES. Icarus 2015 , 251 , 26–49. [ Google Scholar ] [ CrossRef ]
- Laseigneur, Y.; Vincendon, M. OMEGA/Mars Express: A new Martian atmospheric dust hunter. Icarus 2023 , 392 , 115366. [ Google Scholar ] [ CrossRef ]
- Smith, M.D.; Pearl, J.C.; Conrath, B.J.; Christensen, P.R. Mars Global Surveyor Thermal Emission Spectrometer (TES) observations of dust opacity during aerobraking and science phasing. J. Geophys. Res. 2000 , 105 , 9539–9552. [ Google Scholar ] [ CrossRef ]
- Smith, M.D.; Bandfield, J.L.; Christensen, P.R.; Richardson, M.I. Thermal Emission Imaging System (THEMIS) infrared observations of atmospheric dust and water ice cloud optical depth. J. Geophys. Res. 2003 , 108 , 5115. [ Google Scholar ] [ CrossRef ]
- Smith, M.D. Interannual variability in TES atmospheric observations of Mars during 1999-2003. Icarus 2004 , 167 , 148–165. [ Google Scholar ] [ CrossRef ]
- Kleinböhl, A.; Schofield, J.T.; Kass, D.M.; Abdou, W.A.; Backus, C.R.; Sen, B.; Shirley, J.H.; Lawson, W.G.; Richardson, M.I.; Taylor, F.W.; et al. Mars Climate Sounder limb profile retrieval of atmospheric temperature, pressure and dust and water ice opacity. J. Geophys. Res. 2009 , 114 , E10006. [ Google Scholar ] [ CrossRef ]
- Millour, E.; Forget, F.; Spiga, A.; Navarro, T.; Madeleine, J.B.; Montabone, L.; Pottier, A.; Lefevre, F.; Montmessin, F.; Chaufray, J.-Y.; et al. The Mars Climate Database (MCD version 5.2). In Proceedings of the European Planetary Science Congress 2015, Nantes, France, 27 September 2015. [ Google Scholar ]
- Kleinböhl, A.; Spiga, A.; Kass, D.M.; Shirley, J.H.; Millour, E.; Montabone, L.; Forget, F. Diurnal variations of dust during the 2018 global dust storm observed by the Mars Climate Sounder. J. Geophys. Res. Planets 2020 , 125 , e2019JE006115. [ Google Scholar ] [ CrossRef ]
- Vandaele, A.C.; Korablev, O.; Daerden, F.; Aoki, S.; Thomas, I.R.; Altieri, F.; López-Valverde, M.; Villanueva, G.; Liuzzi, G.; Smith, M.D.; et al. Martian dust storm impact on atmospheric H 2 O and D/H observed by ExoMars Trace Gas Orbiter. Nature 2019 , 568 , 521–525. [ Google Scholar ] [ CrossRef ]
- Information Office of the State Council of the People’s Republic of China. China’s Spaceflight in 2021 ; People’s Publishing House: Beijing, China, 2022. (In Chinese) [ Google Scholar ]
- Kleinböhl, A.; Schofield, J.T.; Abdou, W.A.; Irwin, P.G.J.; de Kok, R.J. A single-scattering approximation for infrared radiative transfer in limb geometry in the Martian atmosphere. J. Quant. Spectrosc. Radiat. Transfer. 2011 , 112 , 1568–1580. [ Google Scholar ] [ CrossRef ]
- He, F.; Wei, Y.; Rong, Z.; Reng, Z.; Yan, L.; Tan, N.; Wang, Y.; Fan, K.; Zhou, X.; Gao, J. Monitoring methods for Martian dust storms. Chin. Sci. Bull. 2023 , 68 , 2046–2057. (In Chinese) [ Google Scholar ] [ CrossRef ]
- Montabone, L.; Forget, F. On forecasting dust storms on Mars. In Proceedings of the 48th International Conference on Environmental Systems, Albuquerque, NM, USA, 8–12 July 2018. [ Google Scholar ]
- Fan, K.; Wei, Y. Prospection of multi-spacecraft planetary exploration missions in upcoming future. Chin. Sci. Bull. 2021 , 66 , 4350–4353. (In Chinese) [ Google Scholar ] [ CrossRef ]
Click here to enlarge figure
Mission | Type | Instruments | Years | References |
---|---|---|---|---|
Mariner 6 | Flyby | IR Spectrometer (IRS) | 1969 | [ , ] |
Mariner 7 | Flyby | IR Spectrometer (IRS) | 1969 | [ ] |
Mariner 9 | Orbiter | IR Spectrometer (IRS) UV Spectrometer (UVS) Television (TV) | 1971~1972 | [ , , ] |
Viking 1 | Orbiter Lander | IR Thermal Mapper (IRTM) Visual Imaging Subsystem (VIS) Cameras | 1976~1980 1976~1982 | [ , ] |
Viking 2 | Orbiter Lander | IR Thermal Mapper (IRTM) Visual Imaging Subsystem (VIS) Cameras | 1976~1978 1976~1980 | [ , ] |
Mars Global Surveyor (MGS) | Orbiter | Thermal Emission Spectrometer (TES) Mars Orbit Camera (MOC) | 1997~2006 | [ , , ] |
Mars Pathfinder | Lander Rover | Imager for Mars Pathfinder (IMP) Atmospheric Structure Instrument Navigation Camera (ASINC) Alpha Proton X-Ray Spectrometer (APXS) | 1997 1997 | [ , , ] |
Mars Odyssey | Orbiter | Thermal Emission Imaging System (THEMIS) | 2004~present | [ ] |
Mars Express | Orbiter | Spectroscopy for the Investigation of the Characteristics of the Atmosphere of Mars (SPICAM) Observatoire pour la Mineralogie, l’Eau, les Glaces et l’Activité (OMEGA) High Resolution Stereo Camera (HRSC) | 2003~present | [ , , , ] |
Mars Exploration Rovers: Spirit Opportunity | Rover Rover | Panoramic Cameras (Pancam) Miniature Thermal Emission Spectrometer (Mini-TES) Alpha Particle X-ray Spectrometer (APXS) | 2004~2009 2004~2022 | [ , , ] |
Mars Reconnaissance Orbiter (MRO) | Orbiter | Mars Color Imager (MARCI) Compact Reconnaissance Imaging Spectrometer for Mars (CRISM) Mars Climate Sounder (MCS) | 2006~present | [ , , ] |
Phoenix | Lander | Surface Stereo Imager (SSI) | 2008~2009 | [ ] |
Mars Science Laboratory (MSL) Curiosity | Rover | Rover Environmental Monitoring Station (REMS) Mast Camera (Mastcam) | 2012~present | [ , ] |
ExoMars 2016 Trace Gas Orbiter (TGO) | Orbiter | Nadir and Occultation for Mars Discovery (NOMAD) Color and Stereo Surface Imaging System (CaSSIS) Atmospheric Chemistry Suit (ACS) | 2016~present | [ , , ] |
Insight | Lander | Color Cameras Auxiliary Payload Sensor Suite | 2018~2022 | [ , ] |
Perseverance | Rover | Mastcam-Z SuperCam Mars Environmental Dynamics Analyzer (MEDA) | 2021~ | [ , , ] |
Hope | Orbiter | Emirates Exploration Imager (EXI) | 2021~ | [ ] |
Tianwen-1 | Orbiter | High-Resolution Imaging Camera (HiRiC) Medium Resolution Imaging Camera (MoRiC) Multispectral Camera (MSCam) | 2021~ | [ , , ] |
Levels | VDOD Values | Actions |
---|---|---|
Level 1 | <0.7 | No restrictions. |
Level 2 | 0.7 < VDOD < 1.0 | No restrictions but watch the VDOD closely. |
Level 3 | 1.0 < VDOD < 2.0 | Scientific activities can only be carried out within reasonable limits during the day, with no overnight activities, and the operation team searches for possible parking points for rovers and protective measures for landers. |
Level 4 | 2.0 < VDOD < 3.0 | Start driving to a parking point to safely ride out the dust storm, allowing ONLY essential activities (VDOD observation and battery control board history). |
Level 5 | 3.0 < VDOD < 3.5 | Final drive to parking point, minimal activities ONLY (VDOD observation and battery control board history), begin ultra-high frequency (UHF) overflights per a couple of days, and wait for VDOD to drop. |
Level 6 | VDOD > 3.5 | Minimal activities ONLY (VDOD observation and battery control board history), UHF overflights per couple of days, and wait for VDOD to drop. |
The statements, opinions and data contained in all publications are solely those of the individual author(s) and contributor(s) and not of MDPI and/or the editor(s). MDPI and/or the editor(s) disclaim responsibility for any injury to people or property resulting from any ideas, methods, instructions or products referred to in the content. |
Share and Cite
He, F.; Rong, Z.; Wu, Z.; Gao, J.; Fan, K.; Zhou, X.; Yan, L.; Wang, Y.; Wei, Y. Martian Dust Storms: Reviews and Perspective for the Tianwen-3 Mars Sample Return Mission. Remote Sens. 2024 , 16 , 2613. https://doi.org/10.3390/rs16142613
He F, Rong Z, Wu Z, Gao J, Fan K, Zhou X, Yan L, Wang Y, Wei Y. Martian Dust Storms: Reviews and Perspective for the Tianwen-3 Mars Sample Return Mission. Remote Sensing . 2024; 16(14):2613. https://doi.org/10.3390/rs16142613
He, Fei, Zhaojin Rong, Zhaopeng Wu, Jiawei Gao, Kai Fan, Xu Zhou, Limei Yan, Yuqi Wang, and Yong Wei. 2024. "Martian Dust Storms: Reviews and Perspective for the Tianwen-3 Mars Sample Return Mission" Remote Sensing 16, no. 14: 2613. https://doi.org/10.3390/rs16142613
Article Metrics
Article access statistics, further information, mdpi initiatives, follow mdpi.
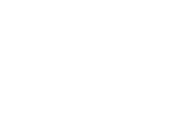
Subscribe to receive issue release notifications and newsletters from MDPI journals
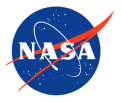
Suggested Searches
- Climate Change
- Expedition 64
- Mars perseverance
- SpaceX Crew-2
- International Space Station
- View All Topics A-Z
Humans in Space
Earth & climate, the solar system, the universe, aeronautics, learning resources, news & events.
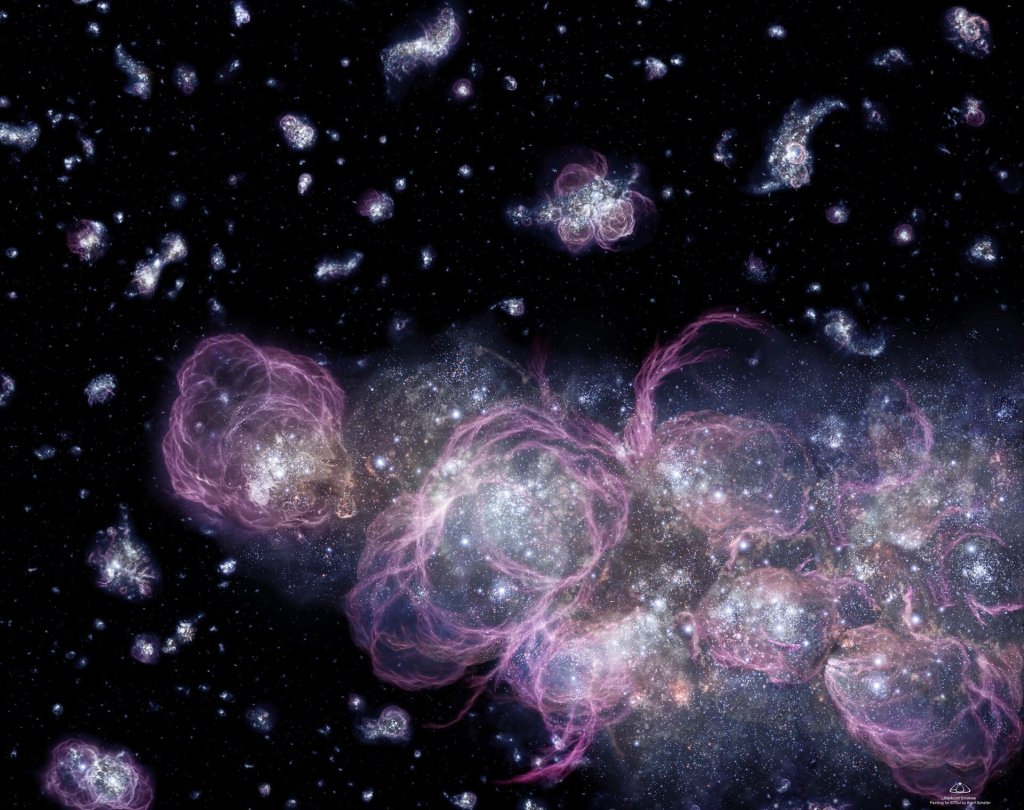
How NASA’s Roman Space Telescope Will Illuminate Cosmic Dawn
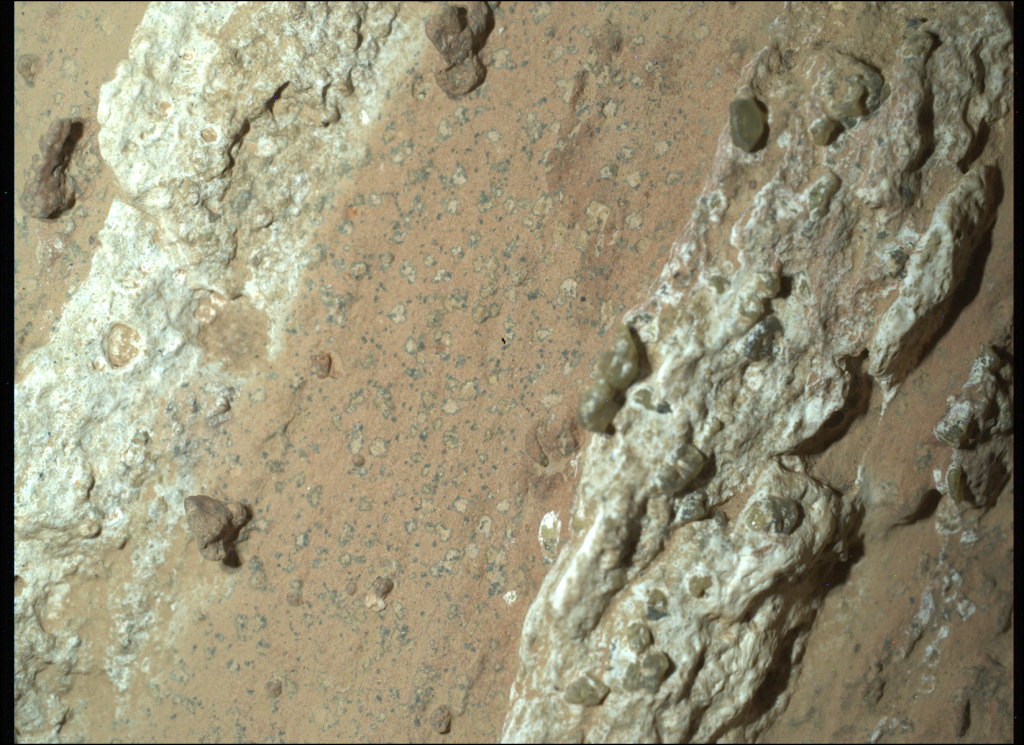
NASA’s Perseverance Rover Scientists Find Intriguing Mars Rock
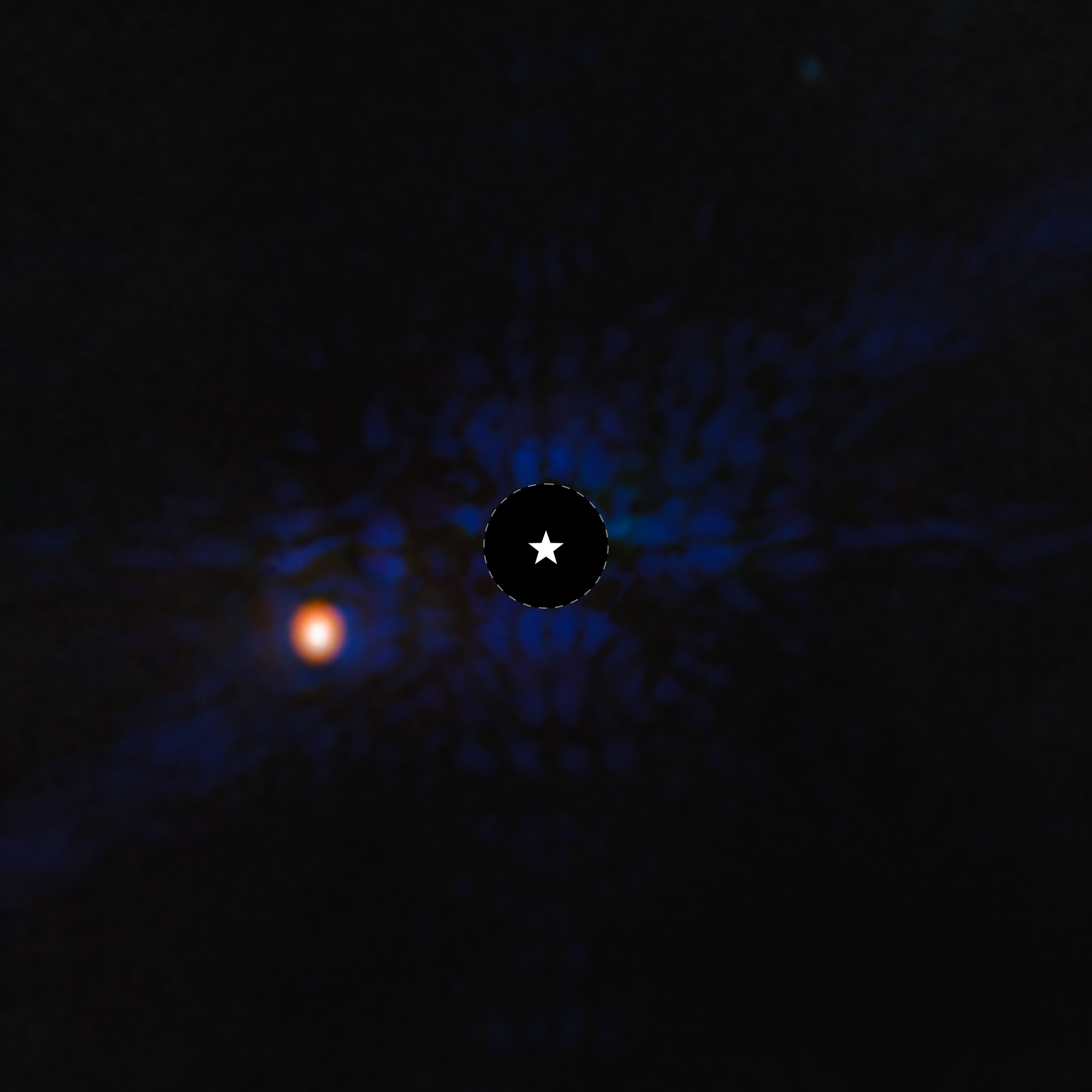
NASA’s Webb Images Cold Exoplanet 12 Light-Years Away
- Search All NASA Missions
- A to Z List of Missions
- Upcoming Launches and Landings
- Spaceships and Rockets
- Communicating with Missions
- James Webb Space Telescope
- Hubble Space Telescope
- Why Go to Space
- Commercial Space
- Destinations
Living in Space
- Explore Earth Science
- Earth, Our Planet
- Earth Science in Action
- Earth Multimedia
- Earth Science Researchers
- Pluto & Dwarf Planets
- Asteroids, Comets & Meteors
- The Kuiper Belt
- The Oort Cloud
- Skywatching
- The Search for Life in the Universe
- Black Holes
- The Big Bang
- Dark Energy & Dark Matter
- Earth Science
- Planetary Science
- Astrophysics & Space Science
- The Sun & Heliophysics
- Biological & Physical Sciences
- Lunar Science
- Citizen Science
- Astromaterials
- Aeronautics Research
- Human Space Travel Research
- Science in the Air
- NASA Aircraft
- Flight Innovation
- Supersonic Flight
- Air Traffic Solutions
- Green Aviation Tech
- Drones & You
- Technology Transfer & Spinoffs
- Space Travel Technology
- Technology Living in Space
- Manufacturing and Materials
- Science Instruments
- For Kids and Students
- For Educators
- For Colleges and Universities
- For Professionals
- Science for Everyone
- Requests for Exhibits, Artifacts, or Speakers
- STEM Engagement at NASA
- NASA's Impacts
- Centers and Facilities
- Directorates
- Organizations
- People of NASA
- Internships
- Our History
- Doing Business with NASA
- Get Involved
NASA en Español
- Aeronáutica
- Ciencias Terrestres
- Sistema Solar
- All NASA News
- Video Series on NASA+
- Newsletters
- Social Media
- Media Resources
- Upcoming Launches & Landings
- Virtual Events
- Sounds and Ringtones
- Interactives
- STEM Multimedia
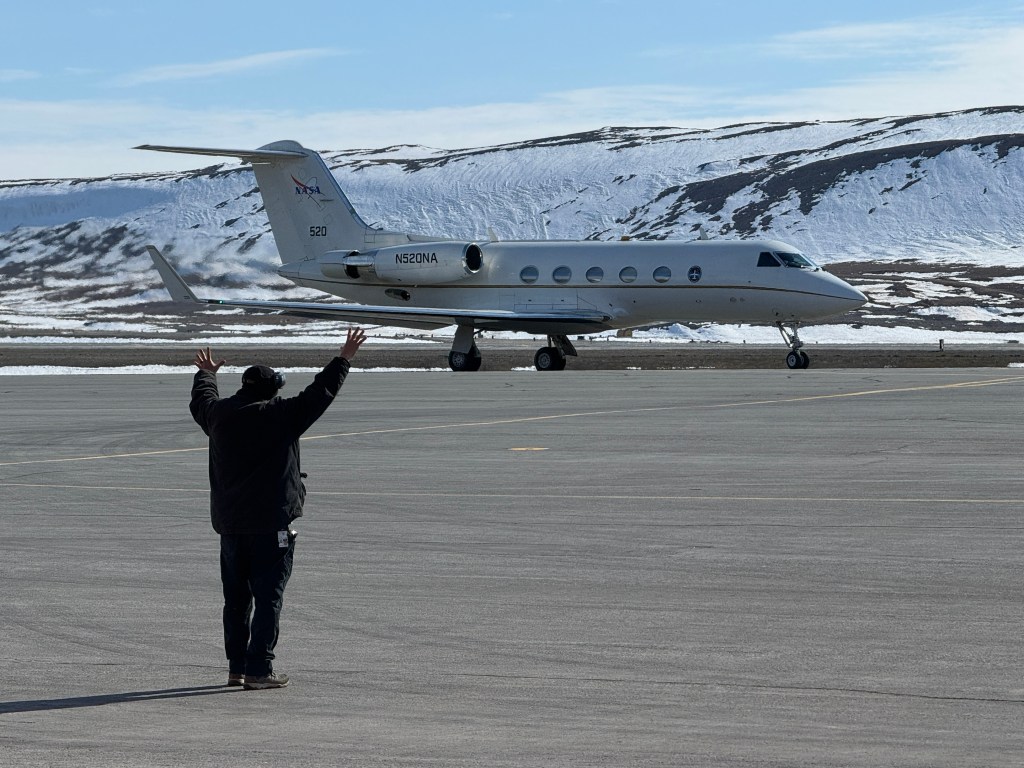
NASA Returns to Arctic Studying Summer Sea Ice Melt
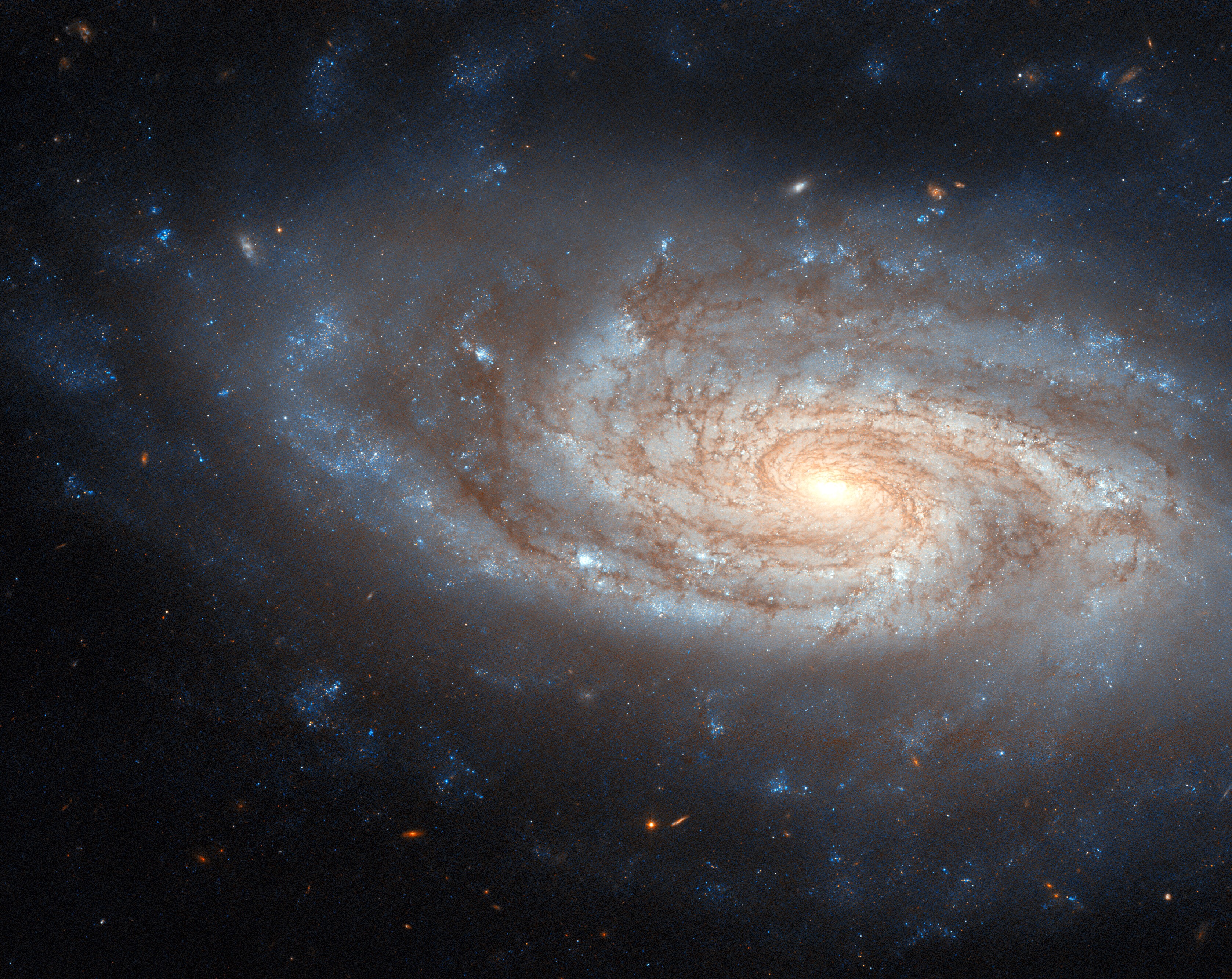
Hubble Images a Classic Spiral
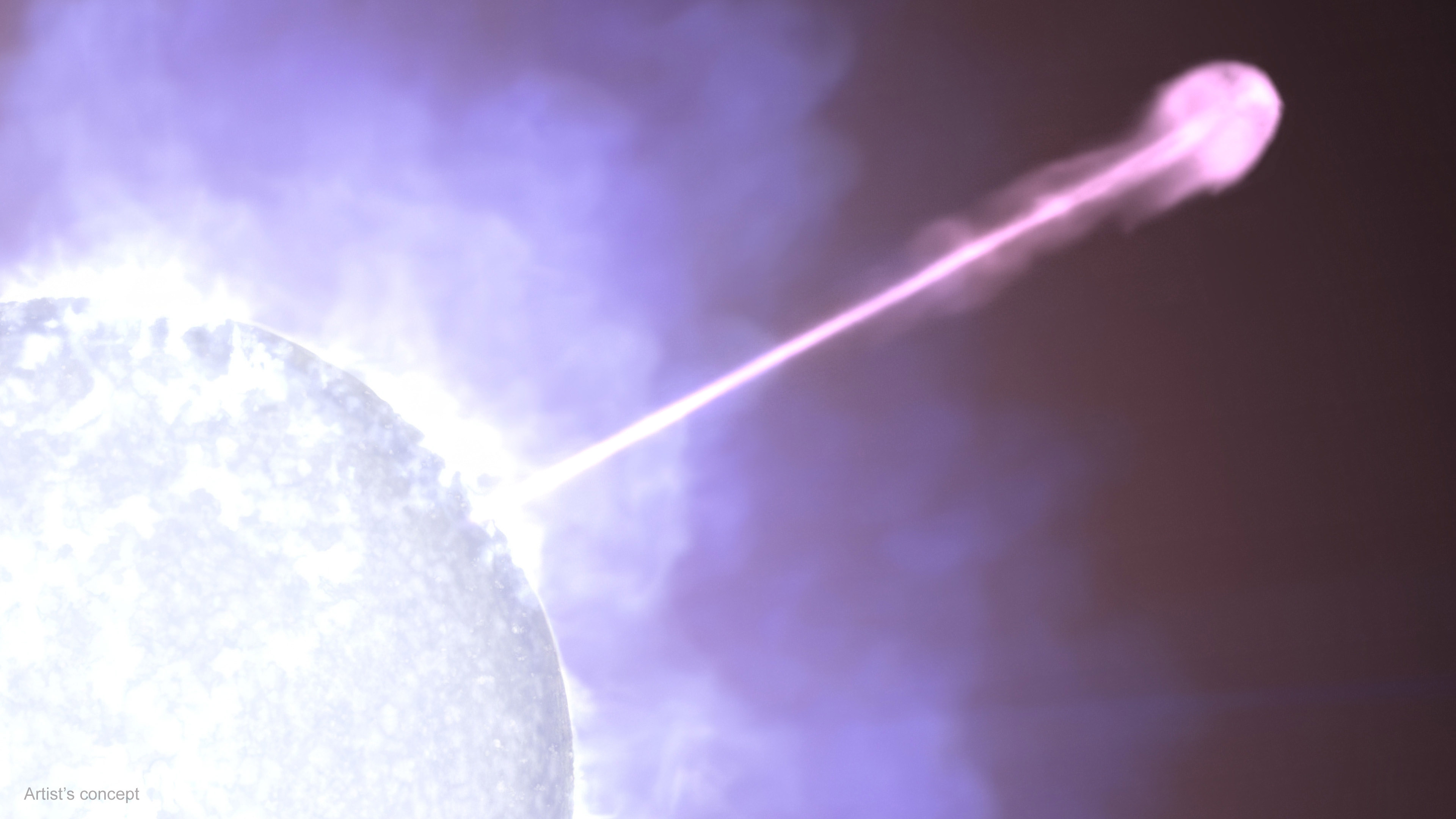
NASA’s Fermi Finds New Feature in Brightest Gamma-Ray Burst Yet Seen

NASA Supports Burst Test for Orbital Reef Commercial Space Station
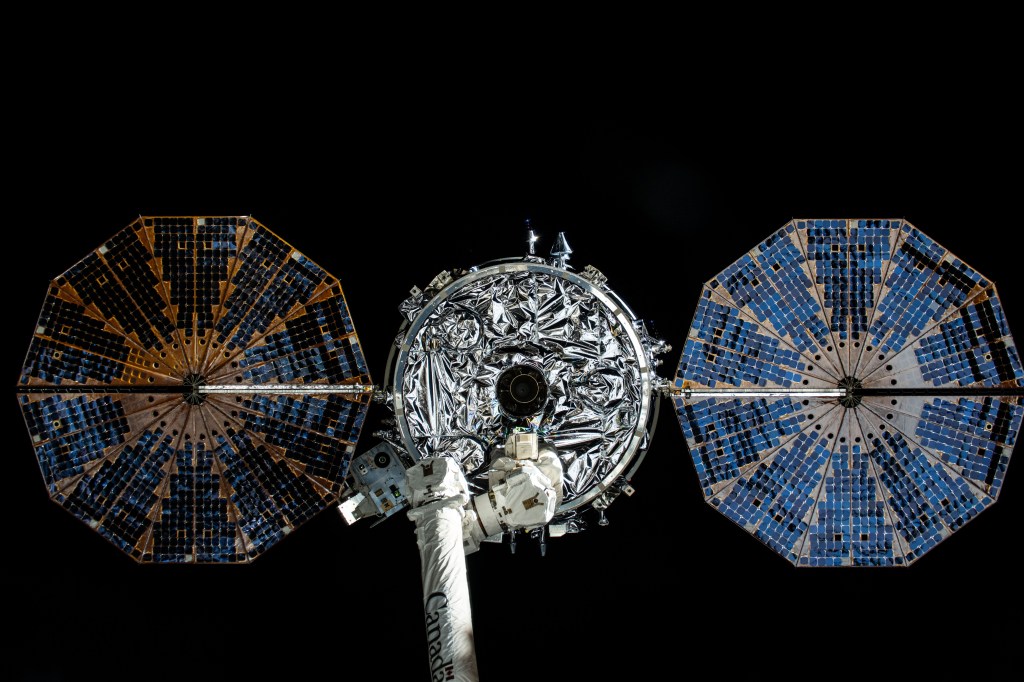
NASA’s 21st Northrop Grumman Mission Launches Scientific Studies to Station

Eileen Collins Broke Barriers as America’s First Female Space Shuttle Commander
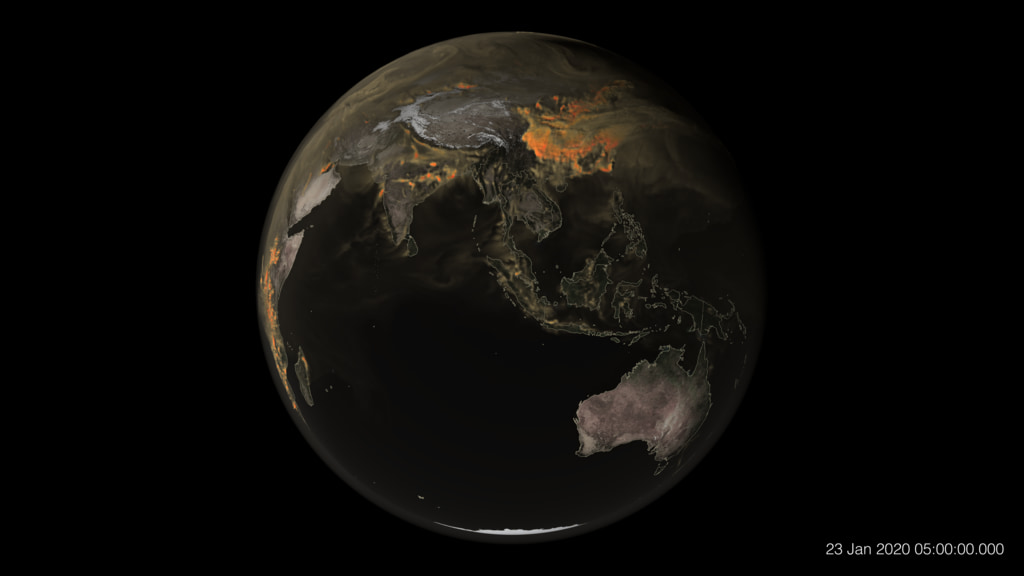
Watch Carbon Dioxide Move Through Earth’s Atmosphere

NASA-Funded Studies Explain How Climate Is Changing Earth’s Rotation
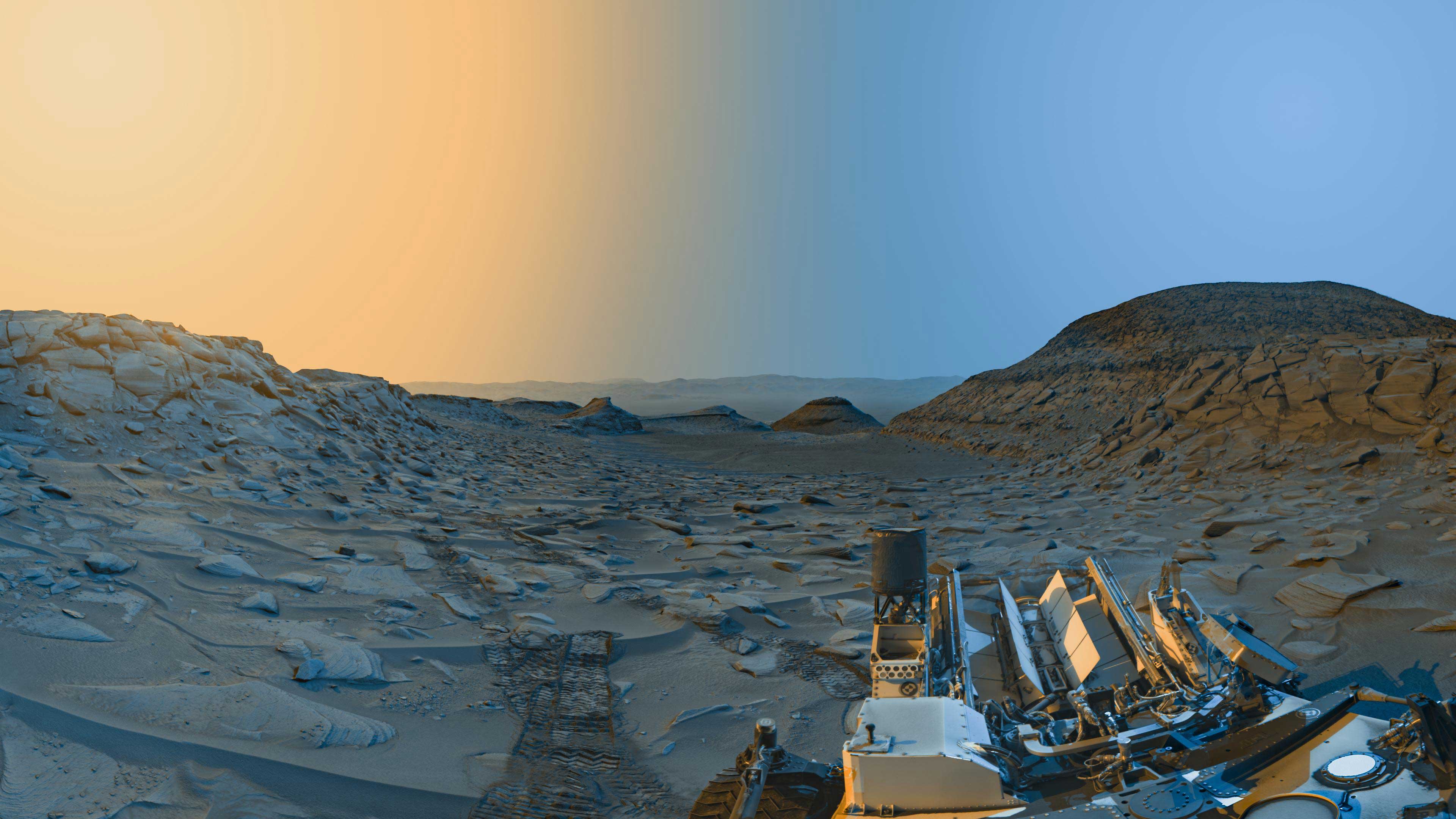
UPDATED: 10 Things for Mars 10
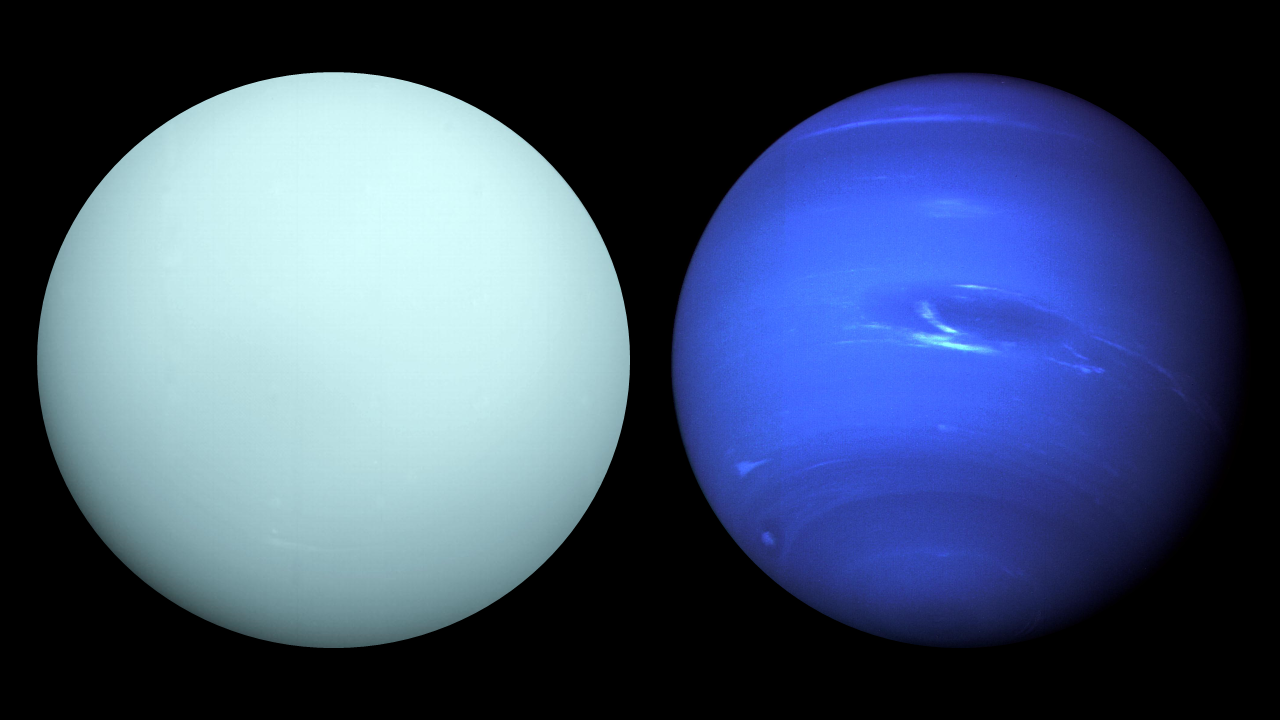
Ice Giant Resources
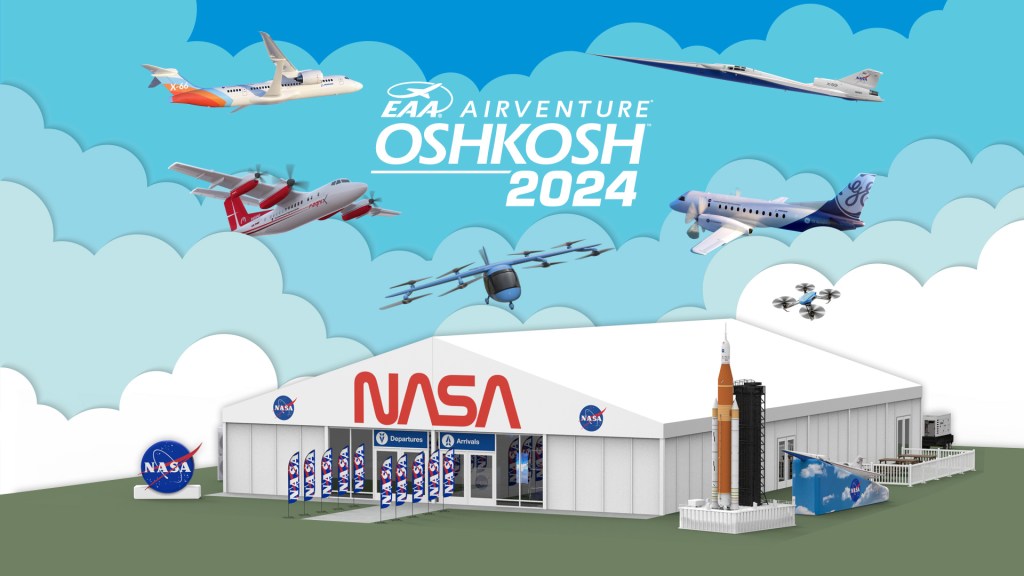
LIVE: NASA is with you from Oshkosh
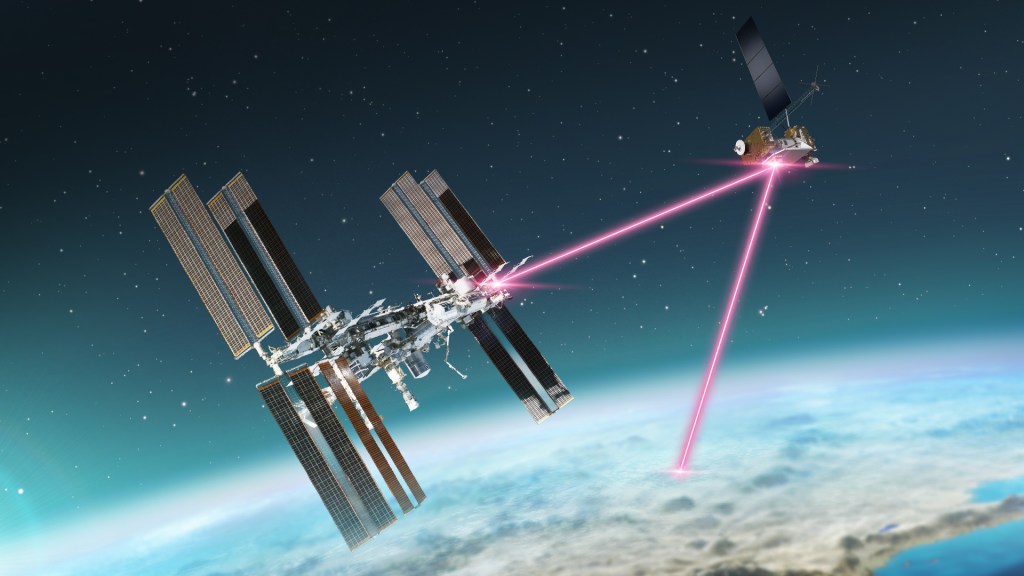
NASA Streams First 4K Video from Aircraft to Space Station, Back
Nasa to host panels, forums, and more at oshkosh 2024.
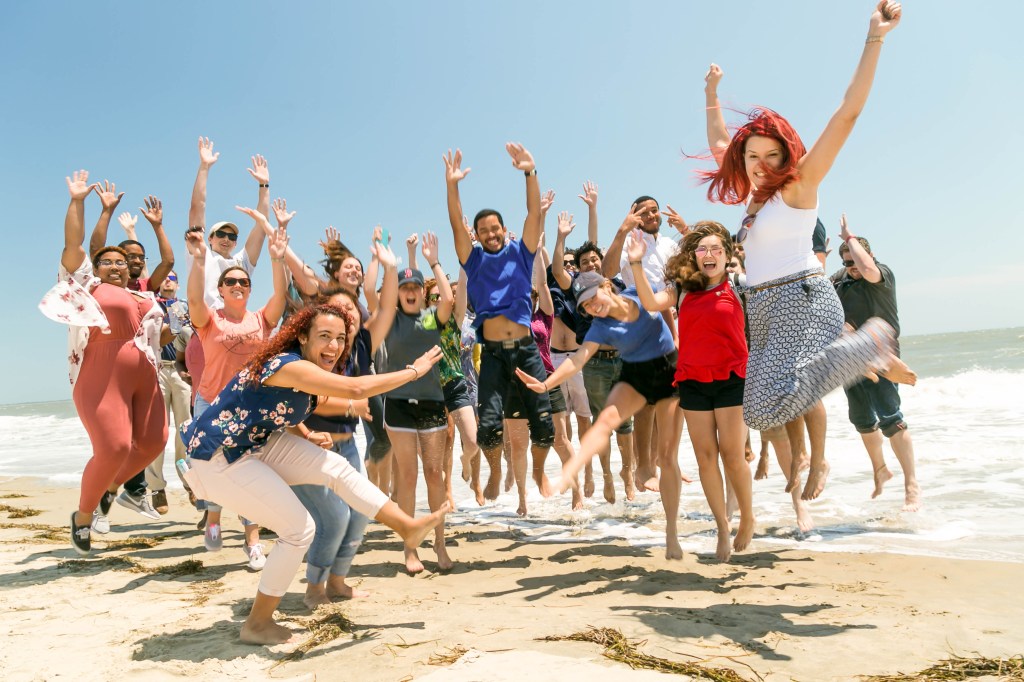
Former Space Communications, Navigation Interns Pioneer NASA’s Future
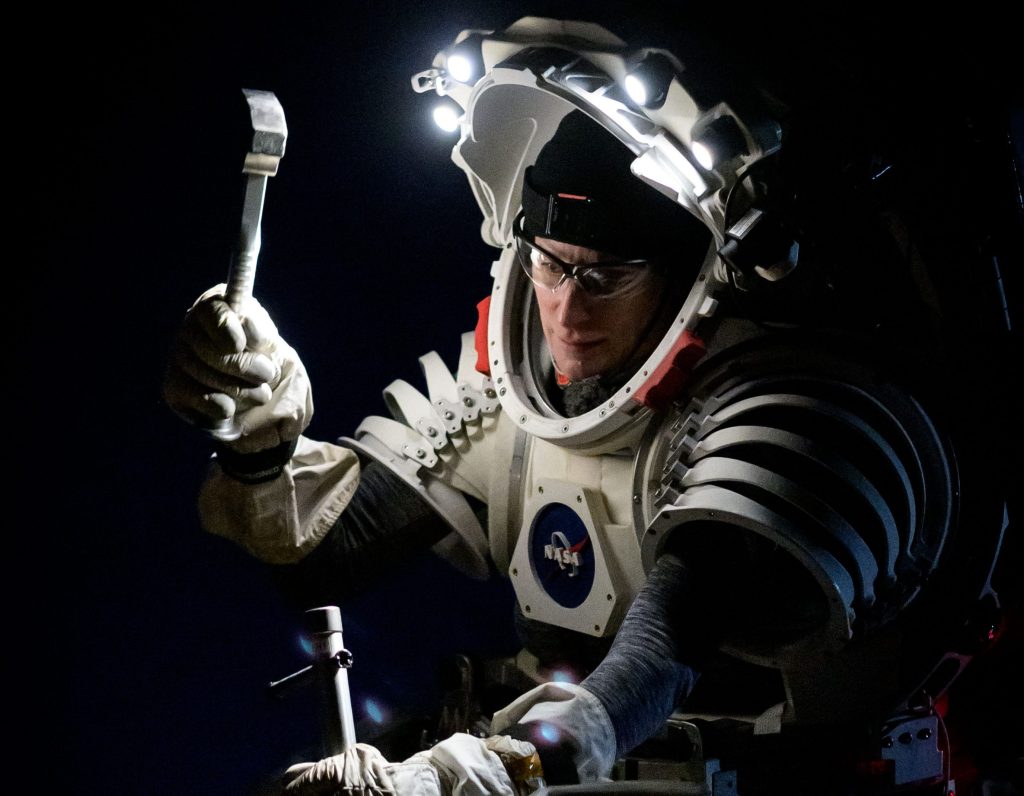
NASA Releases First Integrated Ranking of Civil Space Challenges
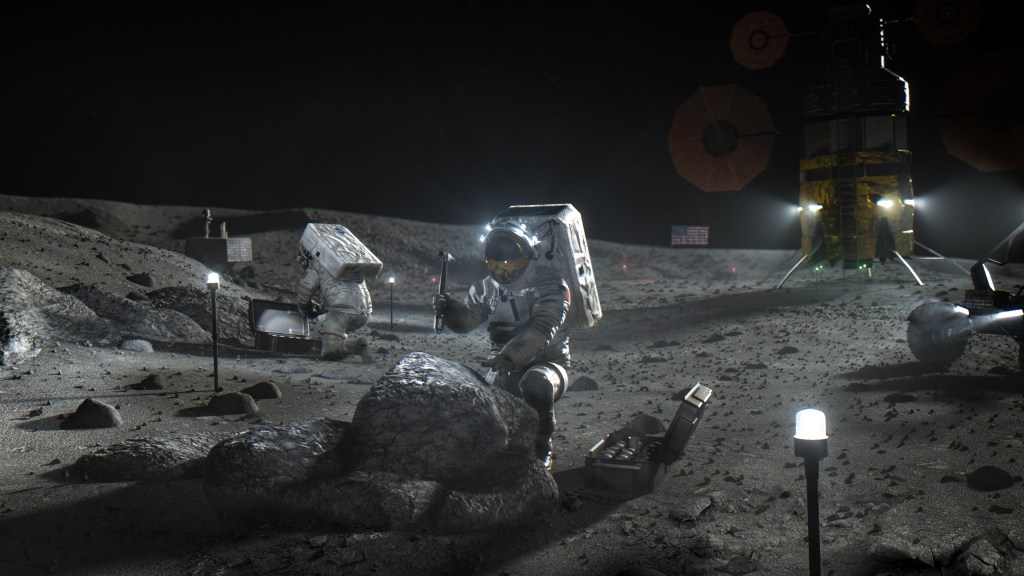
Ground Antenna Trio to Give NASA’s Artemis Campaign ‘LEGS’ to Stand On
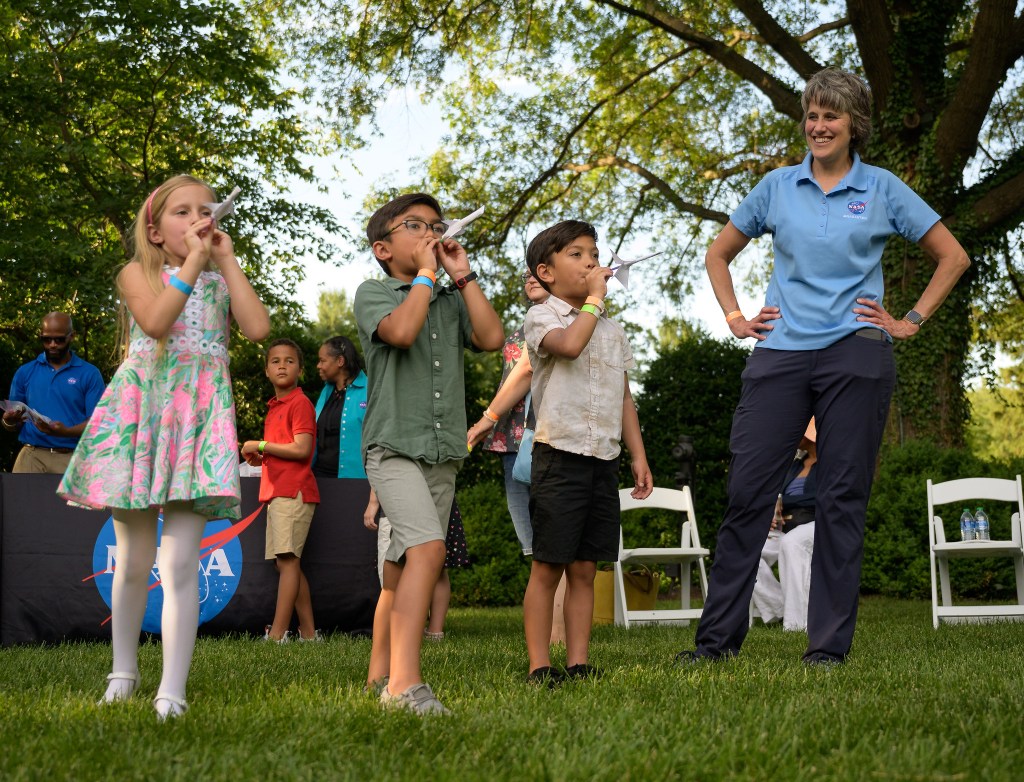
Slow Your Student’s ‘Summer Slide’ and Beat Boredom With NASA STEM
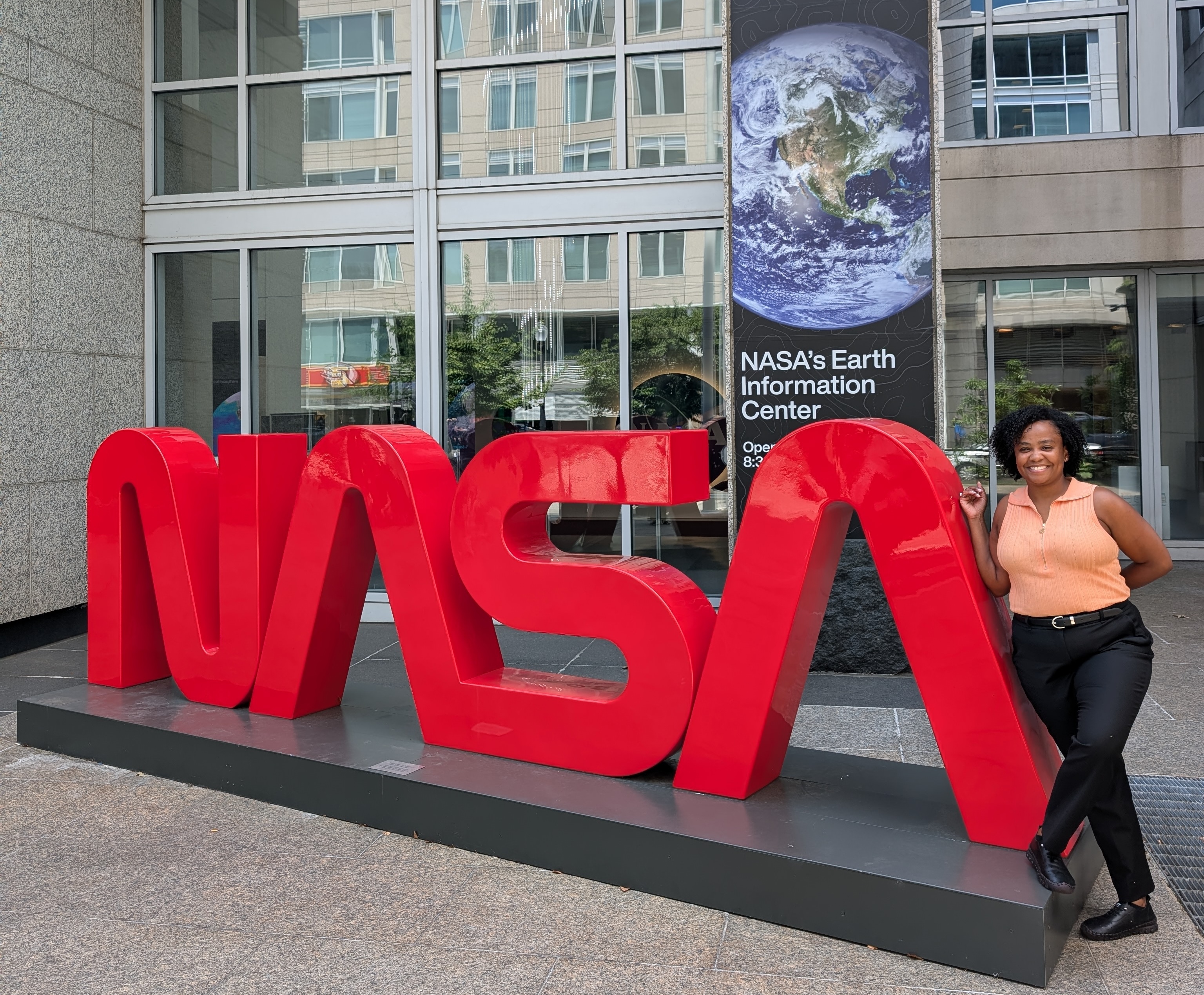
Meet NASA Interns Shaping Future of Open Science
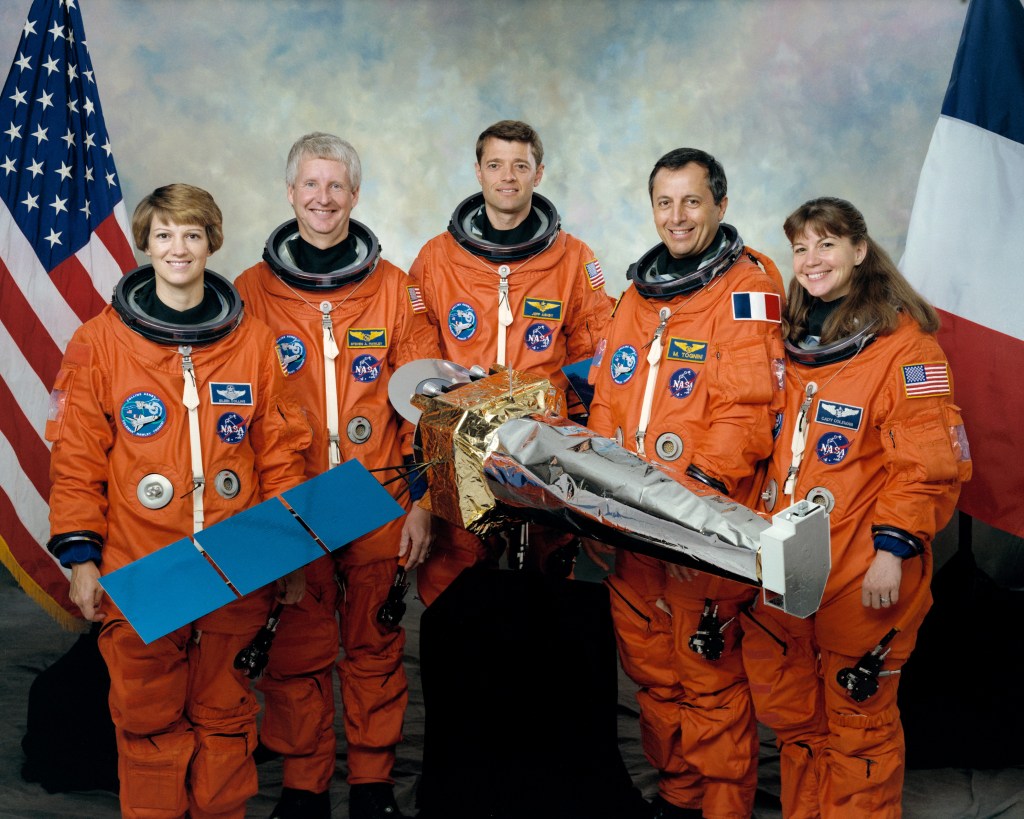
25 Years Ago: STS-93, Launch of the Chandra X-Ray Observatory
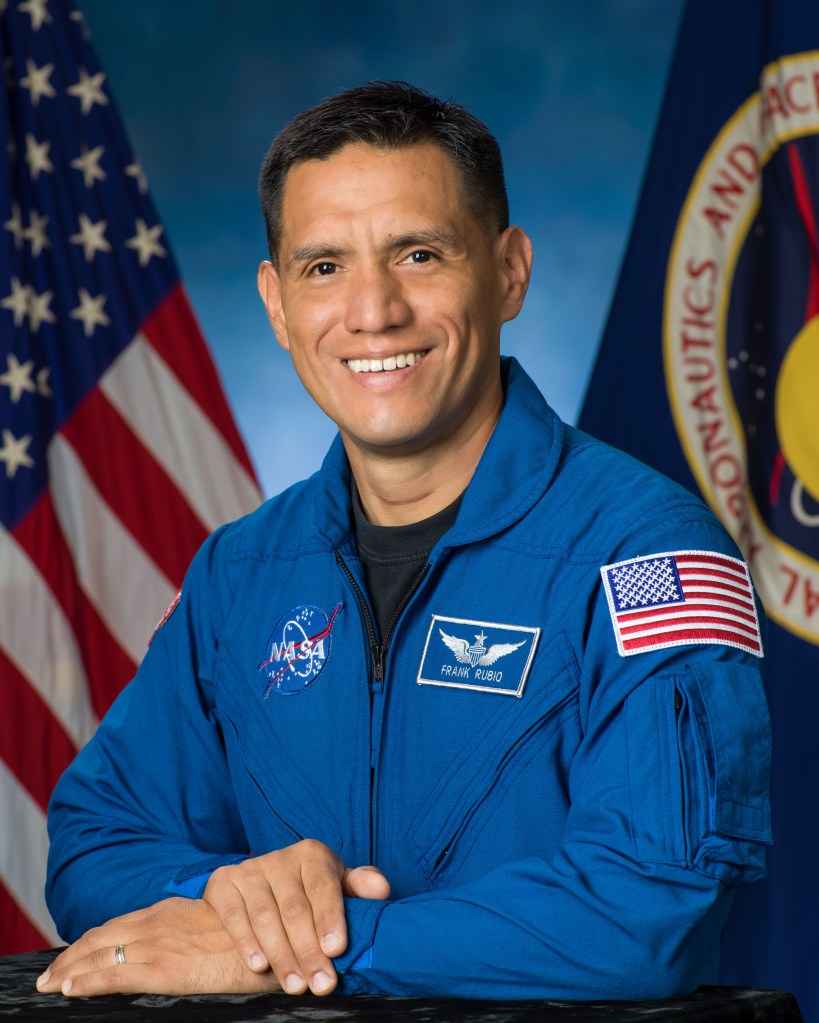
Astronauta de la NASA Frank Rubio
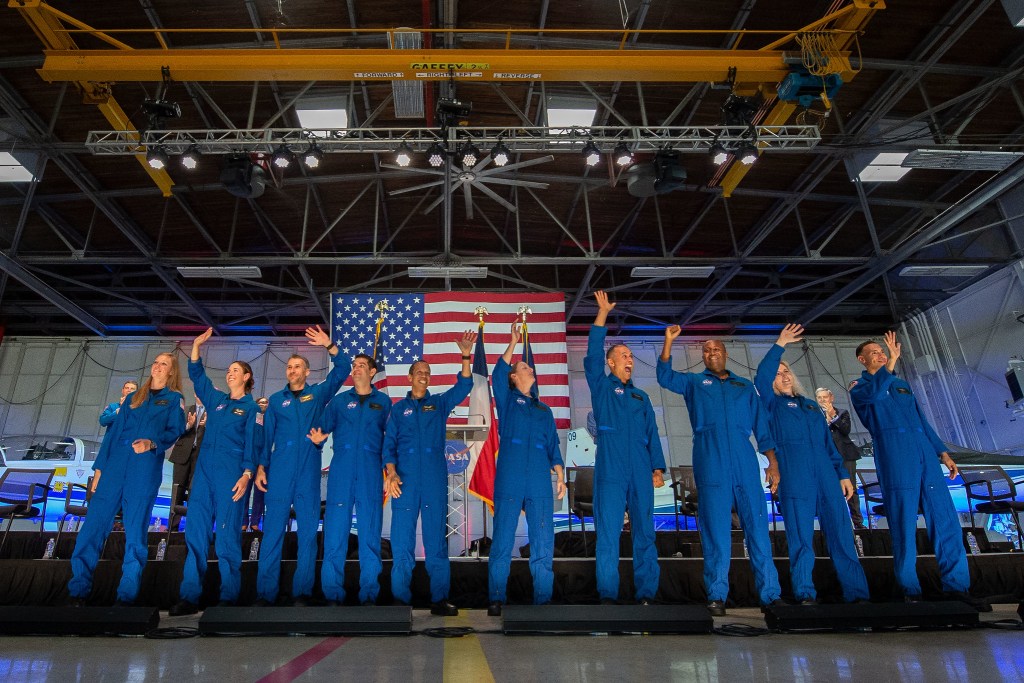
Diez maneras en que los estudiantes pueden prepararse para ser astronautas
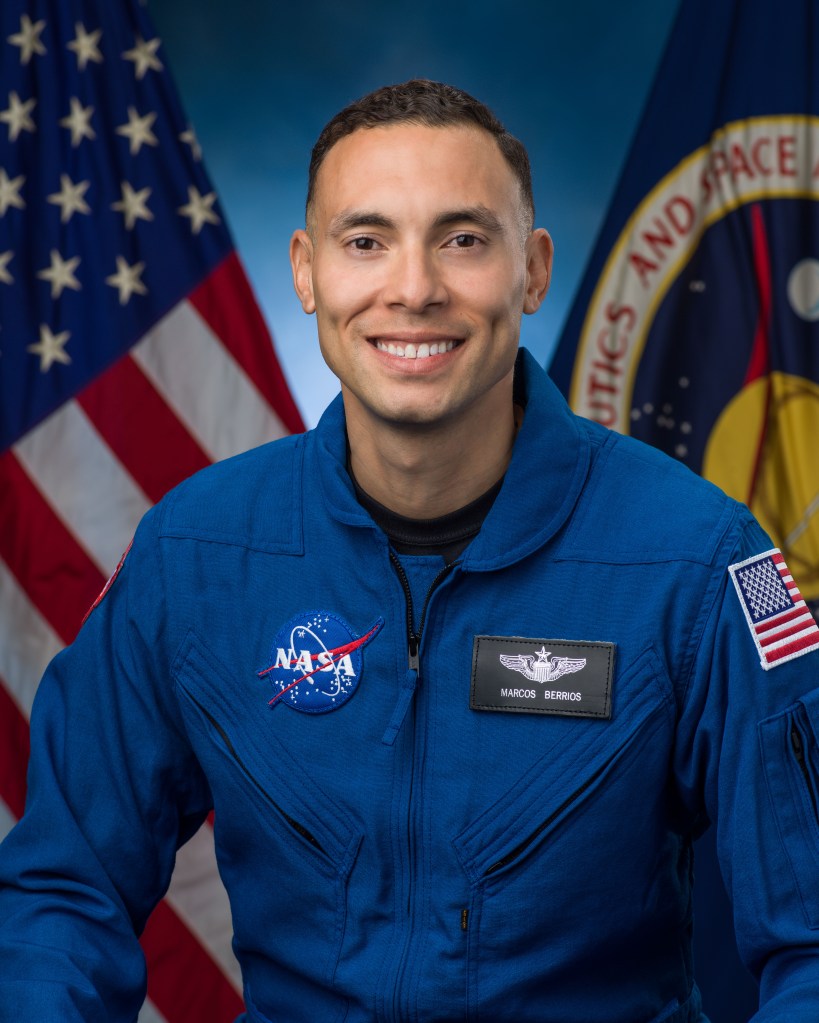
Astronauta de la NASA Marcos Berríos
Nasa research volunteers to begin next simulated mission to mars, hrp communications team, primary crew, alternate crew.

NASA selected a new team of four research volunteers to participate in a simulated mission to Mars within HERA ( Human Exploration Research Analog ) at the agency’s Johnson Space Center in Houston.
Erin Anderson, Sergii Iakymov, Brandon Kent, and Sarah Elizabeth McCandless will begin their simulated trek to Mars on Friday, Aug. 9. The volunteer crew members will stay inside the 650-square-foot habitat for 45 days, exiting Monday, Sept. 23 after a simulated “return” to Earth. Jason Staggs and Anderson Wilder will serve as alternate crew members.
The HERA missions offer scientific insights into how people react to the type of isolation, confinement, work and life demands, and remote conditions astronauts might experience during deep space missions.
The facility supports more frequent, shorter-duration simulations in the same building as CHAPEA ( Crew Health and Performance Analog ). This crew is the third group of volunteers to participate in a simulated Mars mission in HERA this year. The most recent crew completed its HERA mission on June 24. In total, there will be four analog missions in this series.
During this summer’s simulation, participants will perform a mix of science and operational tasks, including harvesting plants from a hydroponic garden, growing shrimp, deploying a small, cube-shaped satellite (CubeSat) to simulate gathering virtual data for analysis, “walking” on the surface of Mars using virtual reality goggles, and flying simulated drones on the simulated Mars surface. The team members also will encounter increasingly longer communication delays with Mission Control throughout their mission, culminating in five-minute lags as they “near” Mars. Astronauts traveling to Mars may experience communications delays of up to 20 minutes.
NASA’s Human Research Program will conduct 18 human health experiments during each of the 2024 HERA missions. Collectively, the studies explore how a Mars-like journey may affect the crew members’ mental and physical health. The work also will allow scientists to test certain procedures and equipment designed to keep astronauts safe and healthy on deep space missions.
Erin Anderson

Anderson earned a bachelor’s degree in Aerospace Engineering from the University of Illinois at Urbana-Champaign in 2013. After graduating, she worked as a structural engineer for Boeing on NASA’s SLS ( Space Launch System ) in Huntsville, Alabama. She moved to New Orleans to support the assembly of the first core stage of the SLS at NASA’s Michoud Assembly Facility . Anderson received a master’s degree in Aeronautical Engineering from Purdue University in West Lafayette, Indiana, in 2020. She started her current job in 2021, continuing her research on carbon fiber composites.
In her free time, Anderson enjoys playing rugby, doting on her dog, Sesame, and learning how to ride paddleboard at local beaches.
Sergii Iakymov

Iakymov received a bachelor’s degree in Aviation and Cosmonautics and a master’s in Aircraft Control Systems from Kyiv Polytechnic Institute in Ukraine. His graduate research focused on the motion of satellites equipped with pitch flywheels and magnetic coils.
Iakymov was born in Germany, raised in Ukraine, and currently splits his time between southern Utah and Chino Hills, California. His hobbies include traveling, running, hiking, scuba diving, photography, and reading.
Brandon Kent
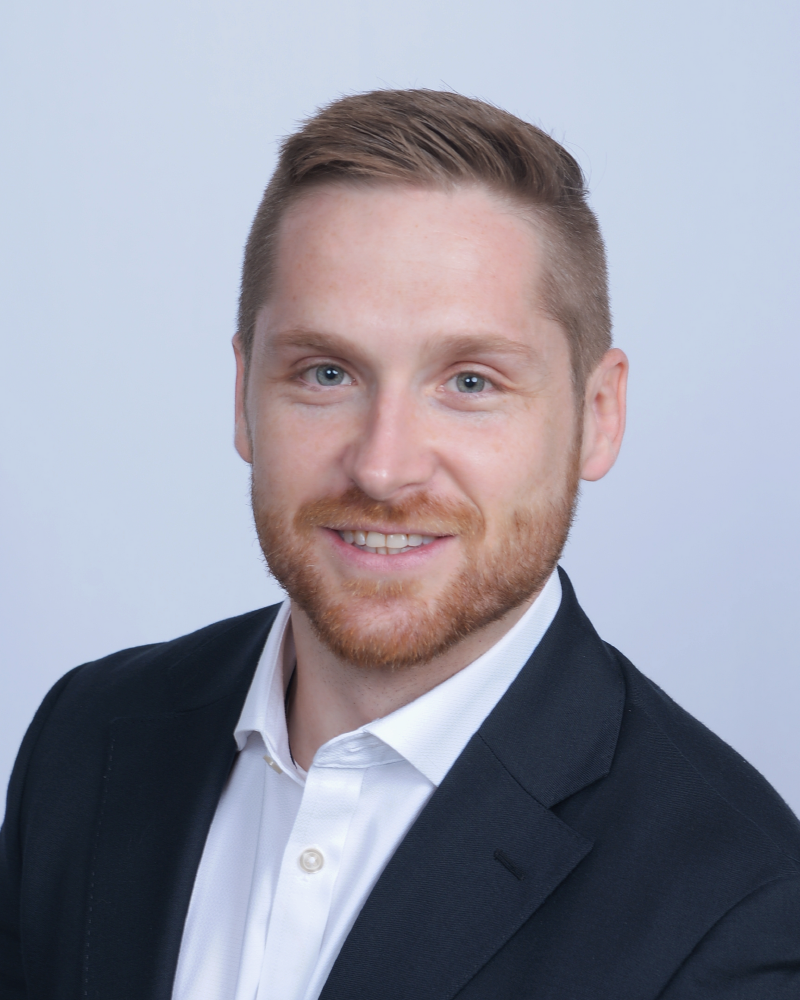
Kent received a bachelor’s degrees in Biochemistry and Biology from North Carolina State University in Raleigh. He earned his doctorate in Biomedicine from Mount Sinai School of Medicine in New York City, where his work primarily focused on how genetic factors regulate early embryonic development and cancer development.
Following graduate school, Kent moved into scientific and medical communications consulting in oncology, primarily focusing on clinical trial data disclosures, scientific exchange, and medical education initiatives.
Kent and his wife have two daughters. In his spare time, he enjoys spending time with his daughters, flying private aircraft, hiking, staying physically fit, and reading. He lives in Kinnelon, New Jersey.
Sarah Elizabeth McCandless

McCandless received a bachelor’s in Aerospace Engineering from the University of Kansas in Lawrence, and a master’s in Aerospace Engineering from the University of Texas at Austin, focused on orbital mechanics.
McCandless is originally from Fairway, Kansas, and remains an avid fan of sports teams from her alma mater and hometown. She is active in STEM (science, technology, engineering, and mathematics) outreach and education and enjoys camping, running, traveling with friends and family, and piloting Cessna 172s. She lives in Pasadena, California.
Jason Staggs

Staggs supported scientific research expeditions with the National Science Foundation at McMurdo Station in Antarctica. He also previously served as a space engineer and medical officer while working as an analog astronaut in the Hawaii Space Exploration Analog and Simulation (HI-SEAS) atop the Mauna Loa volcano.
Staggs received his bachelor’s degree in Information Assurance and Forensics at Oklahoma State University and master’s and doctorate degrees in Computer Science from the University of Tulsa. During his postdoctoral studies at Idaho National Laboratory, Idaho Falls, he investigated electric vehicle charging station vulnerabilities.
In his spare time, Staggs enjoys hiking, building radio systems, communicating with ham radio operators in remote locations, and volunteering as a solar system ambassador for NASA’s Jet Propulsion Laboratory — sharing his passion for astronomy, oceanography, and space exploration with his community.
Anderson Wilder
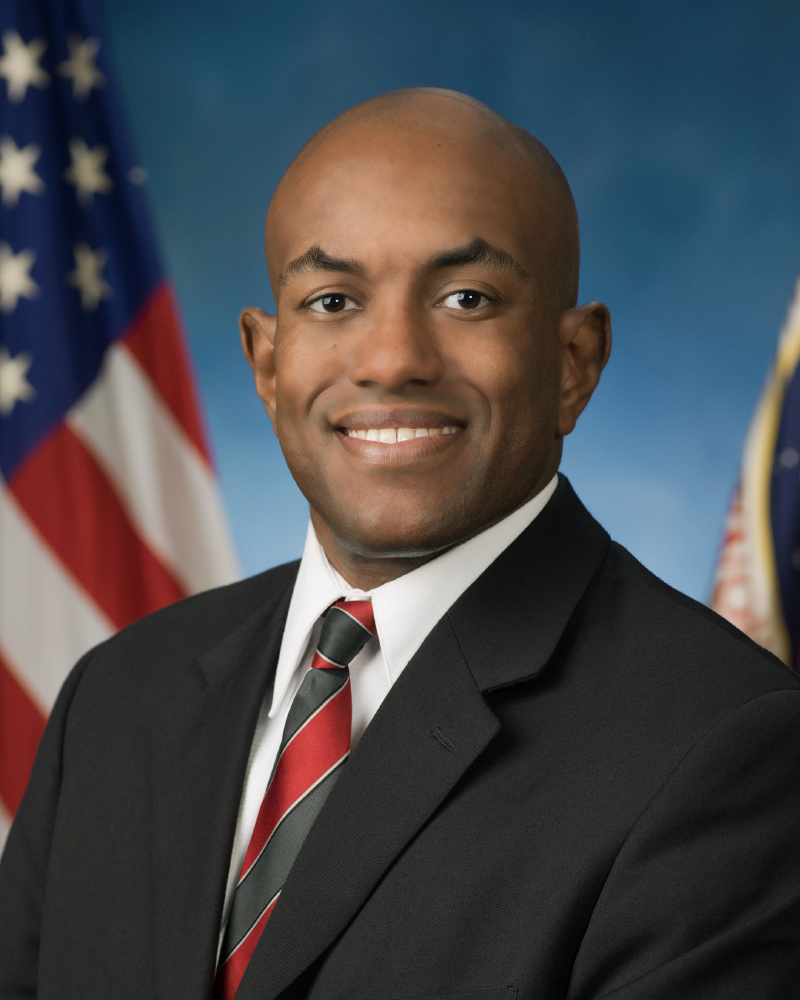
Wilder previously served as an executive officer and engineer for an analog mission at the Mars Desert Research Station in Utah. There, he performed studies related to crew social dynamics, plant growth, and geology.
Wilder received bachelor’s degrees in Linguistics and Psychology from Ohio State University in Columbus. He also received a master’s degree in Space Studies from International Space University in Strasbourg, France, and is completing a second master’s in Cognitive Experimental Psychology from Cleveland State University in Ohio.
Outside of school, Wilder works as a parabolic flight coach, teaching people how to experience reduced-gravity environments. He also enjoys chess, reading, video games, skydiving, and scuba diving. On a recent dive, he explored a submerged section of the Great Wall of China.
NASA’s Human Research Program
NASA’s Human Research Program (HRP) pursues the best methods and technologies to support safe, productive human space travel. Through science conducted in laboratories, ground-based analogs, and the International Space Station, HRP scrutinizes how spaceflight affects human bodies and behaviors. Such research drives HRP’s quest to innovate ways to keep astronauts healthy and mission-ready as space travel expands to the Moon, Mars, and beyond.
Explore More

Discover More Topics From NASA
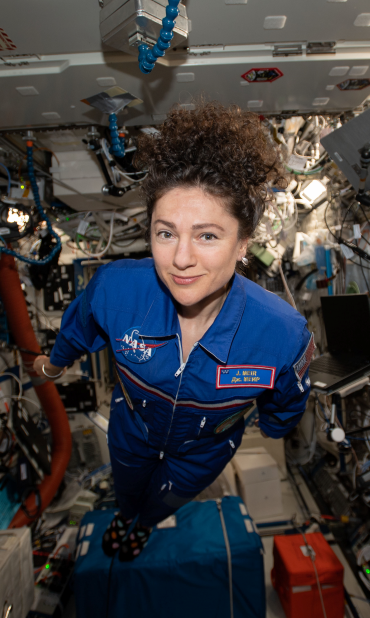
Human Research Program
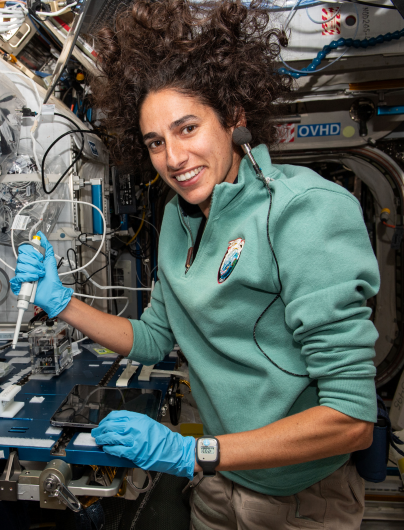
Space Station Research and Technology
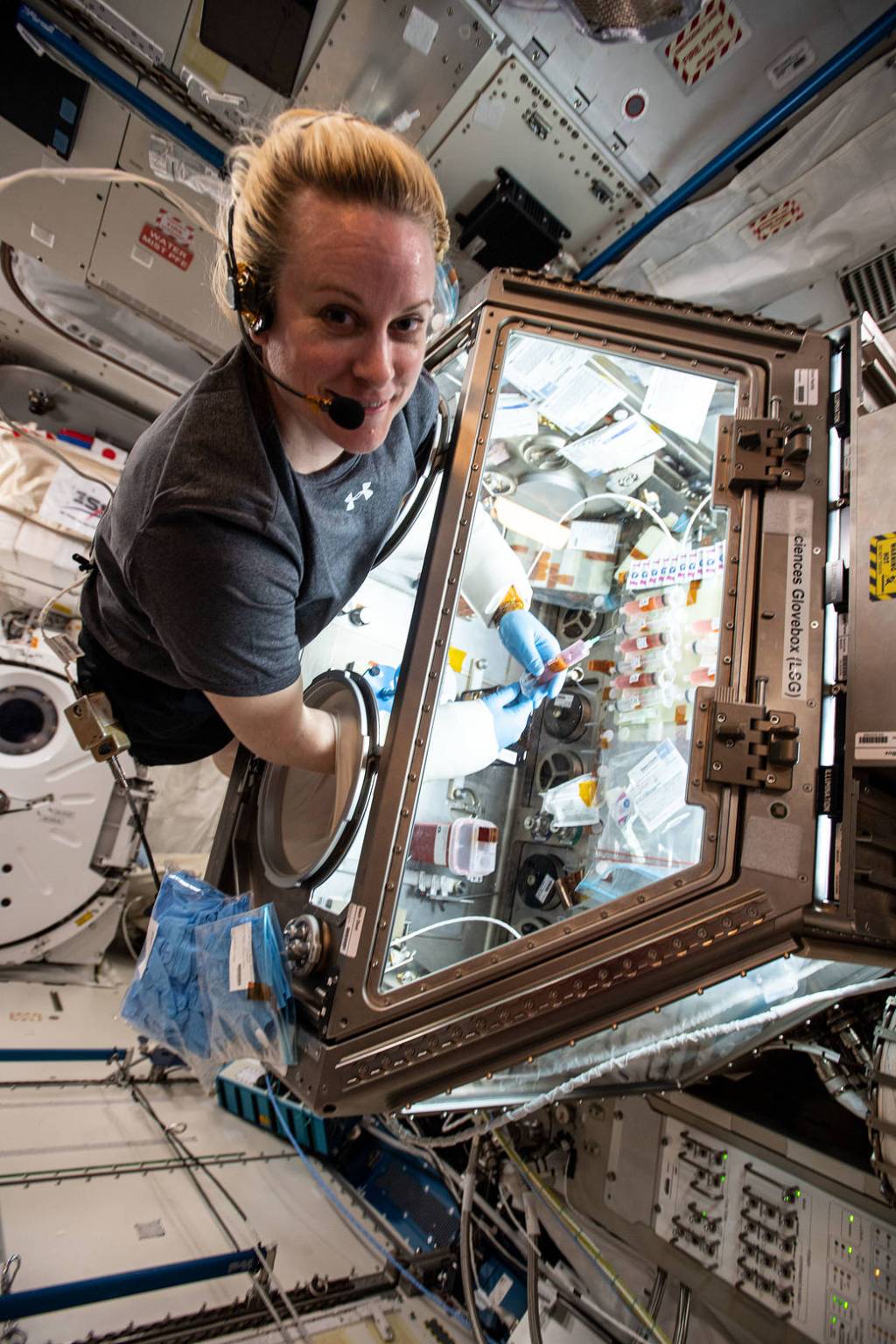
Articles on Life on Mars
Displaying 1 - 20 of 43 articles.
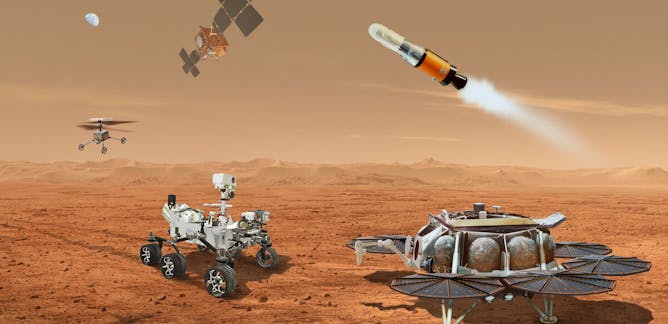
The Mars Sample Return mission has a shaky future, and NASA is calling on private companies for backup
Chris Impey , University of Arizona
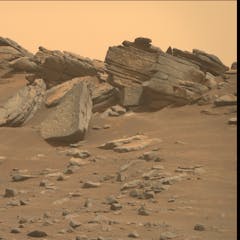
NASA’s search for life on Mars: a rocky road for its rovers, a long slog for scientists – and back on Earth, a battle of the budget
Amy J. Williams , University of Florida
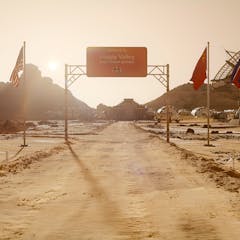
For All Mankind’s Happy Valley: why a Martian city could well extend below the surface
Elizabeth Stanway , University of Warwick
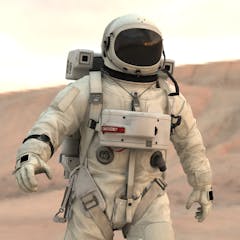
Could people breathe the air on Mars?
Phylindia Gant , University of Florida and Amy J. Williams , University of Florida
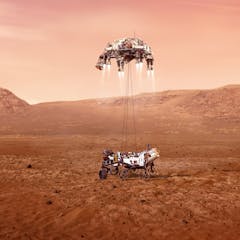
As the Perseverance rover lands on Mars, there’s a lot we already know about the red planet from meteorites found on Earth
James Scott , University of Otago
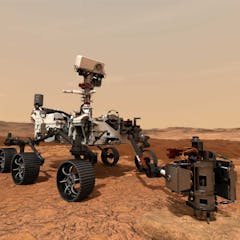
Perseverance Mars rover: how to prove whether there’s life on the red planet
Samantha Rolfe , University of Hertfordshire
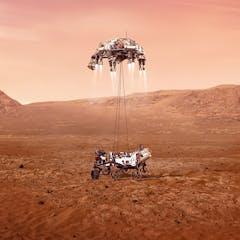
Mars: Perseverance rover set for nail-biting landing – here’s the rocket science
Andrew Coates , UCL
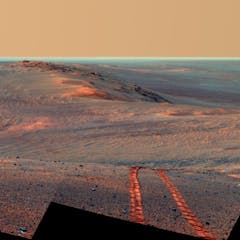
As new probes reach Mars, here’s what we know so far from trips to the red planet
Tanya Hill , Museums Victoria Research Institute
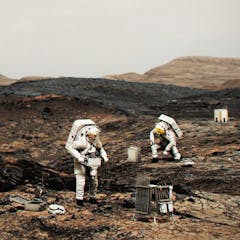
How to get people from Earth to Mars and safely back again
Chris James , The University of Queensland
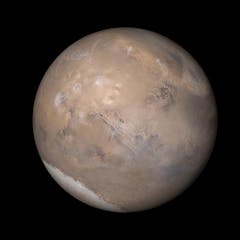
Mars: mounting evidence for subglacial lakes, but could they really host life?
David Rothery , The Open University
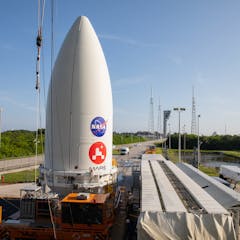
Perseverance: the Mars rover searching for ancient life, and the Aussie scientists who helped build it
David Flannery , Queensland University of Technology
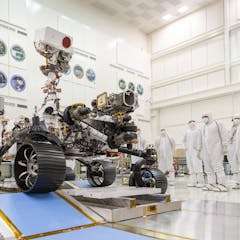

NASA’s big move to search for life on Mars – and to bring rocks home
Briony Horgan , Purdue University and Melissa Rice , Western Washington University
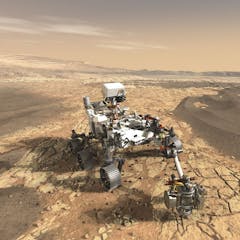
Meteorites from Mars contain clues about the red planet’s geology
Arya Udry , University of Nevada, Las Vegas
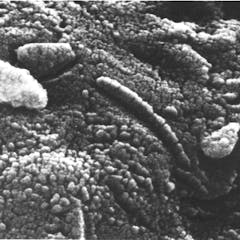
Spotting alien life – how ‘microfossils’ can fool scientists
Alexander Brasier , University of Aberdeen
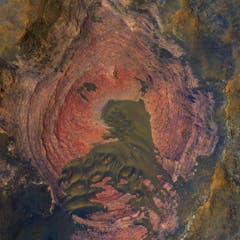
Tiny specks in space could be the key to finding martian life
Andrew Tomkins , Monash University
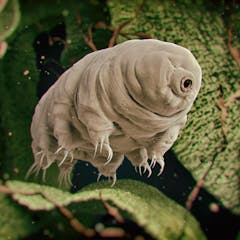
Tardigrades: we’re now polluting the moon with near indestructible little creatures
Monica Grady , The Open University
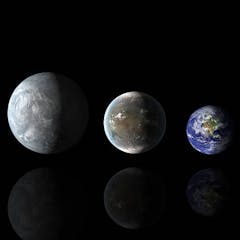
Why the idea of alien life now seems inevitable and possibly imminent
Cathal D. O'Connell , The University of Melbourne
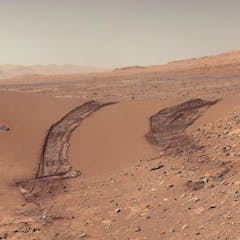
Our long fascination with the journey to Mars
Paulo de Souza , CSIRO
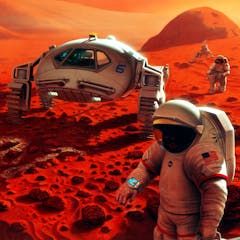
Colonizing Mars means contaminating Mars – and never knowing for sure if it had its own native life
David Weintraub , Vanderbilt University
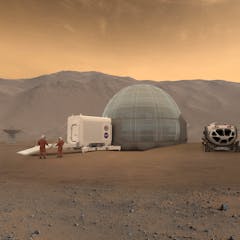
Sorry Elon Musk, but it’s now clear that colonising Mars is unlikely – and a bad idea
Related topics.
- Astrobiology
- Extraterrestrial life
- Mars exploration
- Perseverance Rover
- Space exploration
Top contributors
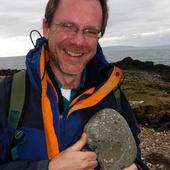
Professor in Earth Sciences, Nanyang Technological University, Singapore, and Emeritus Professor of Mineral Physics, University of Cambridge
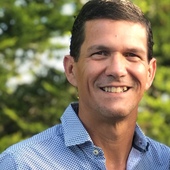
Professor, Griffith University
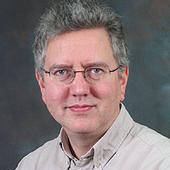
Professor of Physics, Deputy Director (Solar System) at the Mullard Space Science Laboratory, UCL
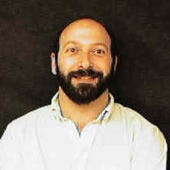
Professor of Astronomy, Vanderbilt University
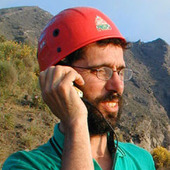
Professor of Planetary Geosciences, The Open University
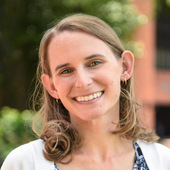
Assistant Professor of Geology, University of Florida
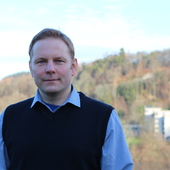
Senior Lecturer in Environmental Science and Planetary Exploration, University of Stirling
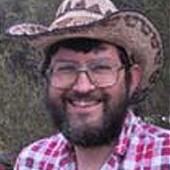
Associate member of the Australian Centre for Astrobiology, UNSW Sydney
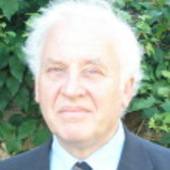
Senior Lecturer in Chemistry, University of Birmingham
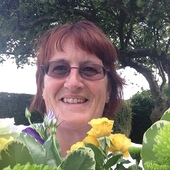
Professor of Planetary and Space Sciences, The Open University
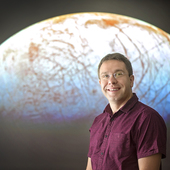
Professor (Astrophysics), University of Southern Queensland
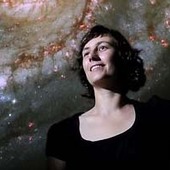
Honorary Fellow at University of Melbourne and Senior Curator (Astronomy), Museums Victoria Research Institute
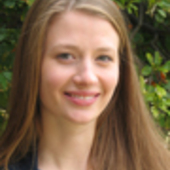
PhD Student in Geosciences, University of Arizona
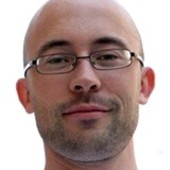
Director, Space Science and Technology Centre, Curtin University
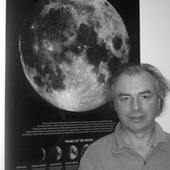
Professor of Planetary Science and Astrobiology, Birkbeck, University of London; Honorary Associate Professor, UCL
- X (Twitter)
- Unfollow topic Follow topic

An official website of the United States government
The .gov means it’s official. Federal government websites often end in .gov or .mil. Before sharing sensitive information, make sure you’re on a federal government site.
The site is secure. The https:// ensures that you are connecting to the official website and that any information you provide is encrypted and transmitted securely.
- Publications
- Account settings
Preview improvements coming to the PMC website in October 2024. Learn More or Try it out now .
- Advanced Search
- Journal List
- v.3(1); 2019 Jan
Mars Colonization: Beyond Getting There
Igor levchenko.
1 Plasma Sources and Applications Centre/Space Propulsion Centre, NIE, Nanyang Technological University, Singapore, 637616, Singapore
2 School of Chemistry, Physics and Mechanical Engineering, Queensland University of Technology, Brisbane, QLD, 4000, Australia
Stéphane Mazouffre
3 CNRS, ICARE, Electric Propulsion Team, 1c Avenue de la Recherche Scientifique, 45071, Orléans, France
Michael Keidar
4 Mechanical and Aerospace Engineering, George Washington University, Washington, DC, 20052, USA
Kateryna Bazaka
Colonization of Mars: As humans gradually overcome technological challenges of deep space missions, the possibility of exploration and colonization of extraterrestrial outposts is being seriously considered by space agencies and commercial entities alike. But should we do it just because we potentially can? Is such an undoubtedly risky adventure justified from the economic, legal, and ethical points of view? And even if it is, do we have a system of instruments necessary to effectively and fairly manage these aspects of colonization? In this essay, a rich diversity of current opinions on the pros and cons of Mars colonization voiced by space enthusiasts with backgrounds in space technology, economics, and materials science are examined.
1. Mars Colonization—Do We Need It?
Mars: Among other potential outposts, the Red Planet has always been shrouded by a veil of romanticism and mystery. Beyond an active target for space exploration, colonization of Mars has become a popular topic nowadays, fuelled by a potentially naive and somewhat questionable belief that this planet could at some point in time be terraformed to sustain human life. 1 Indeed, the Moon, while very close, is small, barren and devoid of atmosphere. Life on the Moon base would not differ from that in the lifeless desert, with no hope of ever finding water. Other neighboring planets, such as hot Venus and gas giants Jupiter and Saturn, are no more suitable for human habitation.
Mars, however, is a horse of a different color. With a mean radius of 0.53 of that of Earth,, i.e., a surface area nearly equal to the total area of dry land on our planet, and 0.38 of Earth's surface gravity ( Figure 1 ), Mars is thought to provide a potentially much more benevolent environment for the colonists from Earth compared to any other proximate planet. Moreover, promising results obtained by rovers and a low‐frequency radar installed on the Mars Express spacecraft have long sustained the belief that it might be possible to find undersurface and subglacial liquid water. 2 Furthermore, similar to Earth, Mars is expected to have substantial mineral resource at and under its surface layer, with a recently confirmed evidence of metal ores and other vital mineral substances. 3 Although no one has seriously demonstrated a practical means for the extraction and refining of these resources into useful products on Mars, a distant possibility of doing so is considered a principal point in favor of colonization. These features of the Red Planet have firmly cemented its status as an ultimate space colonization destination for near future, 4 despite the obvious immediate challenges such as a dusty carbon dioxide‐rich atmosphere, the pressure of which is reaching only 0.09 atm.

Composite image that shows the relative dimensions of Earth and Mars. The image of Earth was captured from the Galileo orbiter at about 6:10 a.m. Pacific Standard Time on December 11, 1990, when it was at a distance of ≈2.1 million kilometers away from Earth during the first of two Earth flybys on its journey to Jupiter. The image of Mars was captured by the Mars Global Surveyor in April of 1999. Image credit: NASA/Jet propulsion Lab. 5
Intense efforts by the world's space agencies and more recently, private enterprises have brought us ever closer to having broad technical capabilities to transport a small number of colonizers and equipment to Mars. These capabilities have been discussed in detail in several comprehensive review and opinion articles that describe various opportunities and challenges facing the Mars settlement program. 6 Proponents of Mars colonization consider present space technology as nearing the stage when it will be able to provide the necessary level of reliability and efficiency required for the one way journey from Earth to Mars. Indeed, a recent example of successful firing of thrusters on Voyager 1 after 37 years of space operation 7 attests to our ability to overcome such significant challenges of spacecraft development 8 , 9 as longevity, reliability, and operational readiness decades after launching. Ongoing advances in nanotechnology and materials engineering enhanced reliability and expanded functionality of contemporary electronics and robotics while reducing device mass, volume, and power consumption. 10 The affordability of small space assets has enabled greater exploration of space, allowing space agencies, universities, and commercial players to collect vital information about extraterrestrial environments in which space assets and living subject will be required to operate, guiding and informing the development of colonization programs. 11
Is it time to go extra‐terrestrial? Mars One program has beenoperating since 2012 and, considering the present level of financial and public support, it is very likely to continue. 12 Falcon Heavy, presently the world's most powerful rocket capable of delivering about 17 tons to Mars surface, was successfully launched on 6 February 2018, demonstrating its capacity to deliver payloads within the framework of Mars One program. 13 In parallel, efforts are made to develop plausible geodynamic scenarios and define relevant parameters, 14 including ambitious ideas of future Mars terraforming. 15 Materials suited for Mars‐oriented applications and operation environments are also under active development. 16 Technical aspects of these projects are described in numerous roadmaps and system architecture description documents. 17 To some, these developments provide confidence that it will indeed be possible to begin colonization of Mars within our lifetime, at least from a technological point of view. And there is certainly no lack of volunteers keen to take on the challenge of a 7 month long one‐way journey to the Red Planet. Indeed, since Mars One's call, thousands have applied and about 100 have been preselected as potential candidates to make up the first crew of four astronauts to be sent to Mars in 2031. 18
Upon reaching the surface, the astronauts will be expected to establish a permanent settlement on Mars, collecting vital data and conducting experiments, with the clear expectation never to return to Earth again ( Figure 2 ). 19

Modular Martian settlement (artistic representation). Several alternative modular concepts have been proposed, including one by Mars One. 11
Settlement of Mars—is it a dream or a necessity? From scientific publications to public forms, there is certainly little consensus on whether colonization of Mars is necessary or even possible, with a rich diversity of opinions that range from categorical It is a necessity! 20 to equally categorical Should Humans Colonize Other Planets? No. 21 A strong proponent of the idea, Orwig puts forward five reasons for Mars colonization, implicitly stating that establishing a permanent colony of humans on Mars is no longer an option but a real necessity. 20
Specifically, these arguments are:
- Survival of humans as a species;
- Exploring the potential of life on Mars to sustain humans;
- Using space technology to positively contribute to our quality of life, from health to minimizing and reversing negative aspects of anthropogenic activity of humans on Earth;
- Developing as a species;
- Gaining political and economic leadership.
The first argument captures the essence of what most space colonization proponents feel—our ever growing environmental footprint threatens the survival of human race on Earth. Indeed, a large body of evidence points to human activity as the main cause of extinction of many species, with shrinking biodiversity and depleting resources threatening the very survival of humans on this planet. Colonization of other planets could potentially increase the probability of our survival.
While being at the core of such ambitious projects as Mars One, a self‐sustained colony of any size on Mars is hardly feasible in the foreseeable future. Indeed, sustaining even a small number of colonists would require a continuous supply of food, oxygen, water and basic materials. At this stage, it is not clear whether it would be possible to establish a system that would generate these resources locally, or whether it would at least in part rely on the delivery of these resources (or essential components necessary for their local production) from Earth. Beyond the supply of these very basic resources, it would be quite challenging if not impossible for the colonists to independently produce hi‐tech but vitally important assets such as medicines, electronics and robotics systems, or advanced materials that provide us with a decent quality of life. In this case, would their existence become little more than the jogtrot of life, as compared with the standards expected at the Earth? 22
This brings us to the second argument—in order to deliver any positive change to the quality of life of humans on Earth, the question of Mars colonization should not only be about survival but also about development if it is to present a viable alternative to our current existence. Such development is inherently linked to the availability of local resources required to sustain life, which is in turn reliant on the availability of instrumentation and equipment necessary for their discovery, extraction and refining. There is little doubt that in early stages of Mars colonization, the greatest fraction of the payload delivered to Mars will be dedicated to equipment needed to provide critical infrastructure and sustain the most fundamental needs of the colony, and not scientific instruments for greater Mars exploration. However, it should be noted that with recent advancements in miniaturized, energy‐efficient electronic and robotics devices, it may in principle be possible to deliver a highly functional yet compact automated laboratory to Mars. A recent breakthrough discovery of (possible) ancient “building blocks of life” made by Curiosity rover greatly supports this notion. 23 , 24 Where Curiosity accommodates only 6.8 kg of scientific instruments, the scientific capabilities of a high‐tech laboratory delivered by one of Mars One landing units solely dedicated to such a mission (i.e., not carrying humans and related resources) could be quite considerable.
The third argument relates to technological advances related to space exploration, specifically how technologies that we may develop in our effort to colonize Mars may find their way into our daily life and deliver unintended benefits. As an example, Orwig points to the image analysis algorithm originally developed for extracting information from blurry images received from Hubble Space Telescope. After the technology was shared with a medical practitioner and as a result applied to medical images, such as X‐ray images, it enabled more accurate visualization of breast tissues affected by cancer, and subsequently led to the development of a minimally invasive stereotactic large‐core needle biopsy. 25 In a separate study, the sequencing and analysis methods developed by NASA to detect and characterize bacterial species on spacecraft to effectively prevent contamination of other worlds with Earth's biota was used to study the link between microorganisms in breast ductal fluid and breast cancer. 26
Finally, the fourth and fifth arguments refer to Mars colonization as an opportunity for humans to grow as a civilization, actively changing the way in which we interact with and exploit our environment. Indeed, in this aspect we can (following Pyne) consider Mars colonization as a kind of cultural invention. 27 Looking back to the Age of Exploration, could the exploration of near‐Earth space together with the Mars and Moon colonization be judged as unavoidable and intuitive continuation of processes started at the dawn of human civilization? Some would argue so, as Shiga points out: “All of the space shuttles – and the ill‐fated Mars rover, Beagle – were named after famous sea vessels.” 28 To many, such a deep attachment to rich history of nautical exploration certainly confirms this hypothesis.
At this point, it is not entirely clear what opportunities and challenges living on Mars will present, and how we as a species would respond to these, but there are certainly calls to embrace innovation and sustainability as the only means to ensure the quality of life for generations to come. Yet, who will oversee and enforce these ideals? Indeed, at its early stages of settlement, the small colony is likely to be composed of altruistic, selfless, technologically savvy individuals who may thrive in an equitable and libertarian society and may be prepared to sacrifice individual desires and benefits for the greater good of the group. However, it is far less likely that such a system can be sustained once the population of colonists grows to thousands and millions and becomes more diverse. Inevitably, a socioeconomic and political order will emerge, and it is likely to be different from the initial system. Would it be possible not to repeat mistakes that we have made when colonizing continents here on Earth?
As we race toward realizing technical aspects of Mars colonization, these and other questions certainly warrant further investigation and discussion. Should we spend a tremendous amount of intellectual, financial and material resources on a distant dream over addressing immediate and highly pressing problems that threaten our very existence on Earth? And is having technological capacity to get there a good enough reason for colonization? In the remainder of this Essay, we will briefly introduce a number of opinions on these issues from stakeholders and space science enthusiasts with diverse backgrounds.
2. Legal Considerations
Right now, the Outer Space Treaty 29 is the main document that governs international cooperation and intercommunication around space and other celestial bodies. While the Outer Space Treaty does not prohibit colonization of Mars, building a permanent colony on the surface of Mars will certainly call for the development of a new system of laws and regulations, which potential colonists would be required to abide by, and which would take precedence over any laws and regulations governing their country of origin. As already mentioned earlier, this may be possible for a small group of like‐minded individuals with common values. Yet, as the colony grows and becomes more diverse with respect to customs, beliefs, traditions and ways of thinking, this may become increasingly challenging. Will it be easy for all interested parties to outline and accept such “Mars constitution”? The success of this endeavor is at the very least questionable, since the major space‐faring nations could not even sign off on The Moon Treaty. 30 , 31 Now, we see efforts by the United Nations to initiate the coordination of space‐related activities, 32 along with active public debates on this problem. 33 , 34 Below we outline some specific legal considerations raised in the recent publications on the topic.
2.1. Do Earth Laws Apply To Mars Colonists?
A set of fundamental questions regarding governance on Mars was formulated by a known proponent of Mars colonization, professor of space law Dunk and discussed by Fecht in her paper Do Earth laws apply to mars colonists ? 35 , 36 Since the demise of Soviet Union, the funding for many national space programs, such as NASA, has not experienced a significant increase, thus keeping the available financial and human resources at a relatively stable level. 37 This provided private companies, such as those led by Musk, an opportunity to emerge and eventually become critical players in space exploration and colonization. Signed in 1967 when space exploration was dominated by nations and not private companies, the current Treaty does not preclude the latter from travelling to Mars, as pointed out by Dunk. 35 , 38 According to his interpretation, private companies can deliver payloads to the surface of the Red Planet and settle on it permanently. We should mention here that the Outer Space Treaty has an international character and does not list specific regulations. However, it does prohibit potential settlers from launching weapons of mass destruction and defining land ownership. These laws are modeled on those on Earth, where deployment of any rocket into space requires multiple levels of authorization at the government and international levels, with the specifics defined by the nature of proposed activities in space. For instance, the launch and operation of a telecom satellite requires approval by the Federal Communications Commission. 39 As global activities in space increase and the number of private enterprises engaged in space exploration grows rapidly, we should expect significant changes in the active regulatory environment in the near future.
While Mars One project has an essentially international character, it still may be bound by the US laws depending on the level of participation of American companies in the project. Mars One is known to rely on third‐party vendors for heavy rocket platforms, with the SpaceX Falcon Heavy, and possibly SLS 40 and BFR 41 being the only realistic options in the near future. Regardless of the country from which it is launched, the rocket produced by an American company will be regarded as an American ship, and, following a very similar approach that governs the behavior of sea‐fairing ships, the space ship would have to abide by the laws of the US legal system. In yet another analogy to the maritime system, the surface of Mars would not belong to any particular country or entity, just as international waters do not belong to any nation. Indeed, even upon reaching the surface of Mars and disembarking the ship, the colonists would be expected to follow the rules of the country that has jurisdiction over their ship. Furthermore, any permanent outpost would be expected to develop an independent governing system, yet the nature of this system is debatable. 35
Recent important efforts to develop an updated legislative system, such as U.S. Commercial Space Launch Competitiveness Act 42 and Act of 20 July 2017 on the exploration and use of space resources 43 aim to go beyond the Outer Space Treaty. These two sets of laws postulate that space resources can indeed be used and exploited by private companies and investors.
On one hand, the early system may capture and be driven by the altruistic nature of early settlers. At the same time, those first settlers will also be subject to a harsh environment, very limited resources and extreme social isolation and uncertainty, potentially necessitating a system that is more hierarchical and rigid. As the colony grows, an increasingly complex legal system may emerge on the back of multifaceted socioeconomic processes, yet it is still likely to be affected by scarcity of resources and a psychologically challenging living environment. As such, it would be necessary to create an authority that would enforce these laws, ensure their effectiveness, and manage those situations where these laws are challenged. Indeed, the latter is inevitable, both because the laws must evolve to adequately reflect a dynamic socioeconomic and technological environment, as well as for the reasons of human nature, where one has a propensity to take advantage of others. 44 With these factors considered, it is difficult to imagine that modern legal systems we currently have on Earth would be appropriate to govern the life on Mars.
2.2. Sovereignty
The question of sovereignty of permanent colonies on the surface of Mars and, possibly, in the Martian orbit is one that at present is not well articulated or defined in the current version of the Outer Space Treaty. At present, it is not possible for a nation or an entity to lay claim of sovereignty over a celestial body or any artificial habitable human outpost, such as a space station. However, it is not clear whether this principle can be upheld as we move into advanced stages of peaceful space colonization, such as that of Mars. Multiple models have been proposed. For instance, Bruhns and Haqq‐Misra suggest a so‐called “pragmatic approach to sovereignty on Mars”, where they explore the benefits of adopting a policy that balances “bounded first possession” against mandatory planetary parks. The former would allow nations to hold legal jurisdiction and exclusive rights to economic benefits derived from a parcel of land, whereas the latter would enable protection of areas of natural, ecological, scientific or cultural significance for the benefit of global community. The proponents of this approach assume that the private property rights‐based economy is the best option for the development of Mars society, and it may indeed be so for the advanced stage of Mars colonization. The relationships between such colonies would be managed diplomatically in accordance with international treaties, and if necessary, the resolution of conflicts may be administered by a formal commission, agency or tribunal with representatives from Mars colonies. Indeed, Bruhns and Haqq‐Misra suggest establishing a Mars Secretariat, the role of which would be to formally enable and facilitate diplomatic communication between interested parties. Broadly, this approach reflects the general principles of the Outer Space Treaty, while providing a more practical model for the management of resources and economic benefits that can be derived from Martian colonies by introducing changes to the non‐appropriation and province of mankind principles. 45 Clarification of the rules that govern the derivation and use of Martian resources by nations and private entities is essential to avoid conflict between future colonies at the stage when resource extraction and exchange would become possible.
2.3. Human Rights
It remains a subject of debate to which extent human rights can be ensured when one considers establishing a permanent colony on Mars. Indeed, there is little doubt that the journey first colonists undertake would be a “one‐way” endeavor. That is, they will have no physical means of ever returning to Earth. The romanticism of being the first to plant a step on the surface of Mars and the overall sense of this effort as being a giant leap for humanity has led to many expressing their strong interest in taking part in the project. At present, these enthusiasts are prepared to sign over their most basic rights of free choice of residence, profession, right to adequate medical treatment and many others for this opportunity. But do we have a legal and in fact a moral right to knowingly subject others to such a life, even with their consent? Below are examples of three different considerations that could play a significant role in such a discussion.
In the first scenario, let us consider a physical illness or mental breakdown that would lead to the volunteer requesting to withdraw their consent to be part of this journey. Would the organizers have a legal right to enforce the original agreement when the participant invokes their human rights and requests their return to Earth through a legal mechanism? Indeed, let us imagine an Earth‐based experiment where a person is subjected to the life‐term isolation in a relatively good, yet significantly restricted environment, e.g., an Antarctic base. The volunteers would document their consent to spend the rest of their lives under the experimental conditions, however at some stage would change their mind and withdraw their consent, requesting that they are removed from the experiment. Would the legal system and public opinion support the company in their choice of forcefully retaining the volunteer under experimental conditions in accordance with their original properly documented consent agreement? It is difficult to imagine that they would, as this would violate the basic human rights of the individual. If so, who will be financially responsible for retrieving these volunteers and returning them to Earth? This situation merits careful legal consideration prior to such a flight.
Let us consider the second scenario where the volunteer legally challenges the agreement on the basis of failure of the entity to comply with promises and conditions of the original agreement. It is hardly difficult to imagine that the reality and specific conditions of life on Mars will be different from even our best estimates and expectations. If these differences are quite substantial, mission participants may give legal grounds for a complaint. Considering that the first wave of colonizers may remain formally under jurisdiction of their country of origin, they would likely retain the full rights to call on their respective legal system and body of authority to protect their interests. Not only can it develop into a complicated legal case for which no precedent exists, it may potentially force the entity in question to take certain measures and as a result jeopardize the success of the mission or program. It is therefore likely that a range of legal and financial obligations will be placed on travel organizers to deal with such complaints. While it may be impossible to retrieve and return colonists to Earth during early stages of colonization, technological advances may eventually make such missions technically possible but prohibitively expensive endeavors. In the worst case scenario, a court's order may be issued, with the enforcement machinery ordering the organizers to take actions on starting the “return project.”
The third scenario that we are going to consider relates to the rights of children born on Mars. Reproductive rights are at the core of many legal systems, and as such would apply to colonists that settle on Mars. These include the right to decide on the number and spacing of offspring, and the right to attain an appropriate level of sexual and reproductive healthcare. Thus, one would expect children to be born on Mars. In fact, some argue that these children would be critical for the long‐term success of the colony as they should be better suited, both physically and psychologically, to the unique living conditions of the Red Planet. They would also be the driving force for the growth and development of the colony, as one could hardly expect all of its inhabitants to be shipped from Earth.
Again, drawing parallels to current legislation on Earth, children born to parents of particular nation would likely inherit the citizenship of their parents, able to exercise the rights of that particular legal system. This in itself may represent a challenge, since given a very small size of the colony, parents may belong to different systems, each having its own idea of how rights of children should be protected. Even within a single system, it is rather challenging to envisage what instruments and mechanisms will be put in place to protect the rights of children on Mars. Similarly, what authority would manage the relationships between children and their parents, or between parents in the case of their separation and divorce? Furthermore, community and family support are critical for families during the time of hardship or conflict, and children on Mars would most certainly lack this safety net.
However, before we even consider potential threats to children's health and wellbeing, at which point would standards of living on Mars reach a minimum acceptable level of health and safety for the reproduction to become ethical? Furthermore, even if we have sufficient technical capability to maintain a decent quality of health and safety of Mars, we would certainly not be able to provide the same degree of choice, e.g., in terms of education or profession, to these children as those available to children on Earth. What legal rights would these children have to request their relocation to Earth? Indeed, are we prepared to rationalize the life of isolation and restriction these children would have to endure—the life they have never consented to. Could—or should—they be considered by the relevant authorities as kids that are retained under what most describe as rather harsh or even inhumane living conditions? Article 6 of the Convention on the Rights of the Child states that “ Governments should ensure that children survive and develop healthily ”; article 24 states: “ Children have the right to good quality health care – the best health care possible .”; and Article 27 requests an adequate standard of living. 46
Apart from these legal considerations, ethical considerations related to the reproduction on Mars may be a significant issue, with some opinions presented in the following section.
We should also mention that these considerations are not exclusive to Mars. For instance, any woman of childbearing age is required to undergo mandatory pregnancy testing before she is allowed to take part in missions that involve extreme conditions, such as an expedition to Antarctica under the U.S. Antarctic Program. 47 And this is considering that it is possible and comparatively easy for the woman to be retrieved from the expedition in the case of medical emergency. In fact, the very nature of such expeditions is temporary, and all members are expected to return home within a relatively short period of time. This is in stark contrast to expeditions to Mars, where participants are expected to be responsible for their own healthcare and wellbeing and have to exclusively rely on their own human and technological capacity permanently.
Further, as the colonist population grows, it is likely that homicides, robberies, and other criminal actions will occur. These events would necessitate some form of criminal justice and punitive system to be established on Mars at the further stages of colonization to prosecute and deliver punitive measures to offenders. Yet, with every pair of hands and skill set being critical for the success of the colony, to which extent would conventional corrective actions be feasible within the unique environment of a space colony? Therefore, the question remains: which laws would apply?
2.4. Abortion
The issues around abortion are closely related to those of human rights, yet often are considered separately due to their intimate relationship to cultural and religious beliefs of different groups of people. Presently, in many nations abortion is viewed as a right of women and a matter of private choice, whereas in others it is legally considered a crime. Considering that a colony on Mars may comprise representatives from different cultural and religious belief systems, it may be difficult to design a policy that would be acceptable to all. Nevertheless, some expect the abortion policy of a Martian colony to be more liberal compared to that on Earth, particularly when it comes to choice based on medical grounds. Indeed, pregnancy termination may be required in instances where pregnancy endangers the life and health of the woman. Similarly, it is difficult to imagine that harsh Martian conditions would be suited for children with severe debilitating medical conditions simply due to the complete lack of infrastructure to afford them a decent quality of life. Caring for such a child would also be quite consuming in terms of time, human and physical resources, potentially redirecting these resources from activities critical to colony survival and development. Beyond these considerations, it is not clear what other medical and biological challenges of reproduction and living on Mars would inform the abortion policy. 48 It is likely that it would emerge and evolve in parallel with our understanding of what life on Mars would entail.
3. Ethical Considerations
Ethical considerations and issues around Mars colonization can be intuitively separated into two significantly different groups of questions, namely:
- • Ethical considerations with respect to humans, both colonists and people of Earth, and
- • Ethical considerations towards Mars itself, including possible extra‐terrestrial life.
Both are important, and below we will outline some opinions, sometimes controversial, around the ethics related to Mars colonization.
3.1. General
Decades of intense efforts by thousands of people and billions of dollars in funding would likely culminate in sending a small group of four to five individuals on a one‐way trip to Mars. The success of the mission would depend on how well these individuals can work together to handle an environment that is extreme both physically and psychologically. It is therefore likely that the greater good of the group and thus the success of the mission would supersede that of individuals, a pattern of behavior that is not typical of people in their natural habitat due to the differences in judgment of values. For this reason, a framework of decisions that benefit the group over an individual is likely to be defined, with considerations over such personal matters as termination of defective fetuses, euthanasia of individuals suffering from incurable debilitating conditions, and the act of sacrifice of individual life for the sake of the colony. 48 There are evident similarities with sacrifices made by individuals during exploration endeavors during the Age of Discovery on Earth. 49 Yet, these historic experiences also tell us that it is virtually impossible to foresee and control the behavior of individuals and groups when subjected to extreme survival situations. From this perspective, it is difficult to say what control if any flight organizations would have over the life of the colony.
NASA Human Research Program aims to study the risks associated with space flight over extended periods of time. Isolation and closed environment are some of the known factors to cause psychiatric distress. 50 These medical conditions can be as damaging to the overall health of the space traveller and success of the mission as effects of space radiation, bone and muscle loss, and treatment of sustained injuries. Studies involving individuals and groups subjected to isolation have shown that social isolation stimulated brain activity toward short‐term self‐preservation, characterized by enhanced implicit vigilance for social threats even in the absence of thereof. Isolation also promoted more abrasive and defensive behavior in individuals, which may negatively affect the social dynamics of a small crew, even to the extent of mission sabotage. These issues, both psychological and physiological, are difficult if not impossible to address, and are independent of cultural, religious or educational background. Knowing the significant risks that cannot be mitigated, how can we make this venture ethical? Of course, all participants will be made fully aware of all known risks associated with the mission, and asked for their consent. However, does informed consent immediately make it ethical? Before we can answer this question, a wide discussion involving stakeholders and general public is certainly necessary to draw a line of what sacrifices are we prepared to take to make space travel and colonization a reality, and whether the benefits of spacefaring truly outweigh all the costs and risks of such adventures. 51
3.2. Human Reproduction—Ethical Considerations
Biological and social challenges of human reproduction at a permanent Mars base are one more serious consideration that could potentially undermine the success of extra‐terrestrial colonization. 48 Studies of human population dynamics on Earth suggest that the success of settlements on Mars would be inherently linked to the ability of early settlers to produce a certain number of viable offspring as these would be critical for the survival and growth of the colonies as self‐sustained entities. Resettlement of individuals from Earth should provide the foundations for a colony, yet overtime should become only a secondary source of residents. According to Impey, a population of at least 5000 is required to ensure long‐term survival of an extra‐terrestrial colony. 52 It is difficult to estimate the physical and financial resources that would be required to realize a colony of such a size on Mars, and without a doubt would take a number of decades from the first successful mission. Indeed, the SpaceX Interplanetary Transport System is expected to carry only a small number of passengers, with a real possibility that not all of these individuals would be able to survive the 7–9 month‐long journey and the initial period of settlement and adaptation on Mars. This is not to say that such large‐scale transportation missions are not being seriously considered, and overtime it is expected that these missions would become more affordable and safer.
It is also difficult to predict the number of individuals that would be prepared to travel to and live on Mars. Indeed, on Earth, migration is an ancient phenomenon, yet it often carries significant negative impacts on health and mental well‐being of both the migrants and the local population. 53 This is often due to a number of factors, such as being not fully prepared to commit and adjust to the new environment, differences in cultural, social and legal norms, and others. Differences in the physical environment may also negatively affect the physical health and wellbeing of newcomers. From this perspective, individuals that are born and brought up within the colony may be better suited to physical and psychological conditions of Mars, and as such may be better prepared to embrace life as part of a colony.
However, realizing sustainable human reproduction on Mars may not be without its challenges. For one, the number of available individuals would be small, affecting genetic diversity and increasing the likelihood of recessive genetic disorders. It will therefore be essential to enforce genetic, epigenetic and phenotypic screening of potential parents prior to conception, and then monitor the health and development of the fetus across all stages of the pregnancy to anticipate and minimize the risks of offspring being born with debilitating conditions. In addition to a legislative framework surrounding termination of fetuses that are unlikely to result in a birth of a healthy child, 48 the same body of arguments may be applied to define which members of the colony should be encouraged or actively discouraged from having offspring.
Another consideration is the potential threat to the entire colony that may arise as a result of reproduction. Indeed, the success of the mission during the journey and within the early stages of the settlement is inherently linked to efficient utilization of human and physical resources. Bearing a child would divert some of these critical (and very limited) resources from the needs of the crew and activities associated with the survival of the crew during the flight and on the surface. Clearly, this warrants further investigation to have a better understanding of all the challenges and opportunities presented by pregnancy and child bearing on health and wellbeing of the crew during early space missions. 54 , 55
Finally, the general question of the growth of population in Mars colonies could be an issue. Indeed, will “native” Mars colonists accept newcomers, especially if living conditions are hard? After which period of time and at what stage of the colony development could they claim the land, or Mars in its entirety, as their property? In short, at which point in time would they come to consider themselves as the real Martians?
3.3. Social Isolation and no Privacy—Rolled Into One
Considering the aforementioned moral and ethical challenges that would need to be reconciled before we venture to Mars, it is evident that the definition of value of human life, choice, and privacy may take quite a different meaning on Mars to that on Earth. From this, one can conclude that the moral and ethical belief system of Martian society would be different to that of their Earthly counterparts, yet these individuals will still be subject to laws of the nation of their citizenship, at least at early stages of colonization. 48 Furthermore, the role of these early settlers is to explore their environment and its effects on human body and social structure. It is likely that these individuals will be subject to ongoing monitoring and surveillance, which can have serious detrimental effects on their mental and physical health. These can exacerbate mental health consequences of physical confinement and social isolation, causing excessive suspiciousness, abrasiveness, stress, depression, and fatigue. 52
In his “Those sent to live and die on the red planet face untold risk of mental illness,” Chambers explores a scenario of what might happen when the psychological pressure of isolation and a complete lack of privacy tip the colonists over the edge of mental breakdown, prompting them to temporarily or even permanently sever these surveillance channels. 56 There is little published research on the extent of extreme psychological burden Mars colonists would be subjected to as part of, e.g., Mars One mission. Yet understanding these would be necessary to inform the selection of prospective participants. For example, resilience, adaptability, curiosity, creativity, and ability to place trust in others were listed as key traits for applicants to Mars One program, yet it is not clear how these will be measured and evaluated, and which traits will be deemed as not appropriate for the mission. Furthermore, it is not evident whether these traits are considered critical for minimizing the likelihood of one developing a mental illness because of prolonged social isolation or whether they are predictors of better emotional stability. Regardless of their attitude, there is little doubt that some of the selected individuals will develop mental illness, since even the most experienced members of space crew develop symptoms of anxiety, depression and apathy after extended period of time in space. This is despite decades of training, and a clear understanding that they will return to Earth upon completion of the mission.
According to an expert in psychology of space exploration and a Principal Investigator on several NASA‐funded and ESA‐sponsored international psychological research projects Kanas, upon departing Earth on their one way journey to Mars, the crew are likely to experience extreme homesickness, boredom, and loneliness ( Figure 3 ), which can lead to anything from dysphoria to psychosis and suicidal thinking. Upon reaching the surface of Mars, the colonists will swap their small spacecraft for an equally restricted base environment (≈50 m 2 per person) in which they would spend the vast majority of their time. 57 This is because Martian atmosphere is unbreathable for a human, with ≈96% CO 2 and <≈1% of O 2 , as opposed to <≈1% CO 2 and 21% of O 2 on Earth. The surface temperature on the Red Planet averages −55 °C (218 K), reaching a peak of ≈20 °C at the equator, and a low of ≈−153 °C at the poles. There is evidence that the enjoyment of natural outdoor environment and diverse sensory experiences reduces stress and improves mental health. 58

Social isolation on Mars would be a great source of stress to the colonists. While Earth is in close proximity to the International Space Station (ISS), it becomes a remote planet when seen from the surface of the Moon and is desperately lost in space when observed from the surface of Mars. Earth photos credit: NASA/Jet propulsion Lab.
“Worse still, imagine a mission that has no Third Quarter. Or no quarters at all! Step forward Mars One. During such a mission, our contestants will be without any of the psychological buffers that every crew has had since Gagarin. No real time interaction with family. No instant access to mission control. No option of returning home” —writes Erik Seedhouse. 59
3.4. Advocacy for Mars—Is It Ethical at All to Colonize It?
One of the strongest arguments in favor of Mars colonization is the survival of humankind in the case of a global event that would significantly compromise or even destroy modern civilization, e.g., a global catastrophe that would make Earth no longer habitable for our species. Having a distant outpost on Mars would allow us to escape the consequences of such an event, and persist as a species. Yet our history tells us that colonists, no matter how responsible, would inevitable affect the environment they colonize. Although our chances of discovering intelligent life in space are quite low, 60 there remains a possibility of discovery of abiogenesis on Mars. Such a discovery would have tremendous scientific and philosophical significance, providing a second, potentially novel example of biochemistry and evolutionary history, and providing evidence for the phenomenon of life being spread across the universe. And most importantly, as an astrobiologist McKay points out, this will be an ultimate proof that extra‐terrestrial life in higher forms is possible. 61
However, what if the native life, no matter how primitive, is incompatible with out notion of what Mars should become in order to accommodate human life. While the environment of Mars is certainly harsh, it may still support extremophiles. Indeed, on Earth there are a number of examples of microorganisms that can withstand extreme temperatures, e.g., Pyrococcus furiosus and Pyrolobus fumarii , pH, e.g., Natronobacterium and Clostridium paradoxum , pressures, e.g., Pyrococcus sp., and radiation conditions, e.g., Thermococcus gammatolerans . If native life is discovered, should it be preserved and protected? Would it even be possible to discover and recognize these most probably microscopic organisms before changing their environment? Currently, to reduce the possibility of contaminating other worlds with microorganisms from Earth, efforts are made to ensure that both the robotic and human exploration of extra‐terrestrial environments is biologically reversible. It should therefore be possible to reverse any possible contamination of Mars if signs of abiogenesis are detected.
However, should we in fact protect this life? On Earth, microbial decontamination is widespread and in fact critical to food safety, healthcare, and in many instances our survival. At which point our own need for survival would give us permission to threaten theirs? 62 If life on Mars is discovered, it may be possible to consider other celestial bodies, e.g., the moons or sufficiently large asteroids, yet at present point in time, Mars appears to be the humanity's best option. 63
Even in the absence of native life forms, there is an obligation for the colonists to attempt to preserve where possible the unspoiled alien environment, to ensure our sustained survival on the Red Planet. Yet, it is unclear how these ideas of preservation of native environment would balance those of terraforming of Mars through global engineering to make its surface and climate hospitable to humans. If attainable, the latter would make colonization of Mars safer and more sustainable. 64 Clearly, it would not be possible to transport all the raw materials required for sustained growth and operation of a colony from Earth. Thus, these would have to be extracted from Martian environment, inevitably changing it.
“Do we deserve to become multi‐planetary? Let us become productive participants in the glorious dance of life. If we can dream of the insurmountable task of becoming multi‐planetary, then surely we can fathom expending the energy, resources and willpower that come with making mindful purchase and waste decisions. If we can succeed in preserving our current planet and its ecosystems, we save human consciousness and the integrity of our values. As Elon Musk describes his desire to keep the “light of consciousness” alive, I press that we also ensure it's brightly illuminated and worthy of traversing this magnificent universe,” writes Shivika Sinha. 65
Apart from moral aspects surrounding the protection of possible life on Mars, there are potential legal issues directly related to preservation of Martian environment. Indeed the Outer Space Treaty does not directly prohibit colonization of Mars, but it explicitly states that “States Parties to the Treaty… pursue studies of outer space, including the Moon and other celestial bodies, and conduct exploration of them so as to avoid their harmful contamination and also adverse changes in the environment of the Earth resulting from the introduction of extra‐terrestrial matter and, where necessary, shall adopt appropriate measures for this purpose” (Outer Space Treaty, Article IX 29 ). Yet, one can hardly imagine Mars colonization to proceed without any significant effect on the planet, let alone Mars terraforming, a process that assumes a significant and irreversible transformation of the environment. In this context, the Outer Space Treaty prescribes international consultations to take place before proceeding with such a project. Yet, what would be considered a harmful effect? It is definitely a gray area with considerable room for interpretation. Moreover, The Committee on Space Research (COSPAR) has also issued the Planetary Protection Policy, designed to regulate biological and other types of contaminations of celestial bodies stemming from human space exploration efforts. 66
4. Consideration of Resources
Finally, let us consider the financial and resource aspects of Mars colonization projects and Mars exploration in general. Could it be a lucrative venture, or will Martian colony become a “groundnut scheme” of our generation?
In recent years, the idea of sustainable space economy where nations and private enterprises may derive financial benefits from extraction and utilization of extra‐terrestrial material and energy resources has gained notable attention. The proposed activities range from mining asteroids and the Moon to space tourism and development of large‐scale on‐orbit platforms that could offer a range of technical capabilities. Development of scientific research stations on the surface of large asteroids, the Moon and Mars are also considered. 67
These are very ambitious yet tremendously costly projects that are highly risky from an investment point of view. What is the current financing model for Mars One project? The realization of Mars One mission to bring humans to Mars is managed by the not‐for‐profit Mars One Foundation, which relies on established aerospace suppliers to develop and assemble its aerospace hardware systems. At present, the cost of delivering a crew of four colonists to the surface of Mars is estimated at about US$ 12 billion with the cumulative cost of about US$ 100 billion, 5 however, their business case would accommodate twice that budget. Although Mars One is in part financed through money from donors across 100 countries and their numbers are growing, the donated money is not sufficient to fully finance the operation. As such, the non‐for‐profit arm of the business works closely with the for‐profit Mars One Ventures, the focus of which is to derive and maximize revenue from activities associated with the mission. These include sales of merchandise, brand partnerships, speaking engagements, and, once the mission is closer to the first human launch to Mars, broadcasting rights, Intellectual Property rights, entertainment content, and events. A portion of the proceeds from these revenue streams (as 5% of gross turnover) feed into the mission. 68
It is evident that at present any potential revenue derived from the mission centers on selling the unique historic experience of sending humans to Mars, rather than from discovery and extraction of resources. There have been speculations by Mars colonization enthusiasts, such as Walker and Zubrin that it may be possible for Mars colony to become profitable by exploiting vast domestic resources of deuterium, which can be used as fuel for fusion reactors. 69 Yet others, including Musk, argue that it is unlikely that Mars would offer anything material that would be financially viable to export to Earth. 70
So, what might be the major benefit of Mars exploration? Should we not start by fixing our own planet and learning from this experience before attempting to conquer another outpost? Stratford tackles this notion from a different angle, and proposes to consider Mars colonization as a stimulus that is desperately needed by our contemporary society to move forward and once again regain our ability to tackle pressing problems head on:
“We need an inspired generation to take fast action on so many fronts, but so far, our generation is not inspired. We have instead grown cynical and soft. Sending humans to Mars is the wildcard our world needs to change us from a stagnating, inward‐looking society into a problem solving, frontier‐looking society. It can be done now, and humans can be on Mars within the next ten to fifteen years. We just have to make that decision to go. If we can do this with Mars, this will be the first step forward for our society becoming a “can do” world. Let's take that step” —writes Frank Stratford . 71
5. Quo Vadis, the Only Civilization We Know?
Even among space enthusiasts, there is a rich diversity of opinions regarding “if,” “how” and “when” we should proceed with our space exploration and colonization ambitions. Unless we face a major cataclysm that would immediately threaten our existence on Earth, it is unlikely that a consensus on whether we need a Martian outpost would be reached any time soon. As it stands now, Mars One and similar projects are likely to continue, evolving and morphing as we learn more about the worlds beyond our own. As we gain new technological capabilities and grow our presence in the near‐Earth space, with both areas showing no sign of slowing down, we may be faced with moral and ethical challenges of sending humans to Mars far sooner than anticipated.
At present, it is challenging to comprehensively outline all related questions, let alone offer feasible solutions to these formidable challenges. The aim of this brief Essay is to introduce the interested reader to a vast range of arguments pro and contra Mars colonization, and many often contradictory and antilogous drivers for this project. This is not surprising for such a global challenge, and there is little doubt more questions will emerge, from shorter‐term “Would the colonists be representative of the global human population?” and “Who will finally decide who gets to go?” to longer reaching question around legal matters, the growth of Mars population and development of the social life on Mars.
Even the selection of the most proper “model of civilization” is still an open question. Indeed, there is no monolithic human civilization on Earth to mirror. Furthermore, establishment of societies of altruistic technologically savvy individuals may be far more challenging that it is anticipated. Indeed, with no relevant experience in building similar isolated, artificially built societies, the experience of polar investigators and long‐term space station expeditions, possibly complemented with the long‐term Moon station experience, will have to be used as the best available approximation for the self‐establishing, self‐organizing Mars colonies.
Conflict of Interest
The authors declare no conflict of interest.
Acknowledgements
This work was supported in part by OSTIn‐SRP/EDB, the National Research Foundation (Singapore), Academic Research Fund AcRF Tier 1 RP 6/16 (Singapore), and the George Washington Institute for Nanotechnology (USA). I.L. acknowledges the support from the School of Chemistry, Physics and Mechanical Engineering, Science and Engineering Faculty, Queensland University of Technology.
Levchenko I., Xu S., Mazouffre S., Keidar M., Bazaka K., Global Challenges 2019, 3 , 1800062 10.1002/gch2.201800062 [ PMC free article ] [ PubMed ] [ CrossRef ] [ Google Scholar ]
Contributor Information
Igor Levchenko, Email: [email protected] , Email: [email protected] .
Kateryna Bazaka, Email: [email protected] .
Academia.edu no longer supports Internet Explorer.
To browse Academia.edu and the wider internet faster and more securely, please take a few seconds to upgrade your browser .
- We're Hiring!
- Help Center
Life on Mars
- Most Cited Papers
- Most Downloaded Papers
- Newest Papers
- Mars Follow Following
- SETI (Search for Extraterrestrial Intelligence) Follow Following
- Grateful Dead Follow Following
- Iroquoian Societies (Archaeology) Follow Following
- Iroquoian linguistics Follow Following
- Ancient Astronaut Theory Follow Following
- Hopi studies (Anthropology) Follow Following
- Ziggurat Follow Following
- Universe expansion Follow Following
- Norse-Gaelic contact Follow Following
Enter the email address you signed up with and we'll email you a reset link.
- Academia.edu Journals
- We're Hiring!
- Help Center
- Find new research papers in:
- Health Sciences
- Earth Sciences
- Cognitive Science
- Mathematics
- Computer Science
- Academia ©2024
Mars: The Exploration of the Red Planet Research Paper
- To find inspiration for your paper and overcome writer’s block
- As a source of information (ensure proper referencing)
- As a template for you assignment
Introduction
Mars, the fourth planet in order of increasing distance from the sun and the first beyond the earth’s orbit. Under favorable conditions, it appears in the night sky as a yellowish red object (hence the name “red planet”) of the first magnitude. Mars has long fascinated us because of its many similarities to the earth and because of the possibility that life might exist there. The flyby of the crewless spacecraft Mariner 4 past the planet in 1965 started an era of intense exploration that still continues. Following several crewless flybys and orbiters launched by the United States (Mariners 4, 6, 7, and 9) and by the Soviet Union (Mars 3, 4, 5, and 6), the first successful soft landing of a spacecraft on another planet was achieved on July 20, 1976, when the U.S. spacecraft Viking 1 landed on the surface of Mars. Since then, Mars has been visited by several unpiloted craft, including the Mars Pathfinder spacecraft in 1997 and the Mars Global Surveyor from 1997 to 2006. (Squyres, 12) When images from these two probes were compared, scientists began to suspect that water had once flowed at several locations. Since 2004, NASA’s (National Aeronautics and Space Administration’s) Mars Exploration Rovers twin robot-geologists Spirit and Opportunity have explored the harsh Martian environment in search of water. The Phoenix Mars Lander, which safely reached the planet’s the North Pole in 2008, will analyze the icy soil for evidence of past microbial life. Mars is now perceived as a planet of spectacularly diverse topography with huge volcanoes, deep canyons, dry riverbeds, and extensive sand seas. While evidence of life there continues to be elusive, Mars remains interesting for geologic, chemical, and meteorological comparisons with the earth (Paolo, p.89).
Telescopic Observations
Following the first telescopic observations of Mars by Galileo in 1610, the planet has been observed continually, with changes in its appearance noted and mapped. Mars is too distant for any surface relief to be discerned through the telescope. All that is seen are bright and dark markings, which may be in the atmosphere or on the surface. Most surface markings are in the equatorial regions, where various dark features contrast with the light areas or “deserts.” The shape and size of most markings change both seasonally and, slowly, over many years. Despite the many changes, the most prominent features are recognizable even on the earliest maps. The markings show poor correlation with topographic features revealed by spacecraft observations. Most are thought to result from thin deposits of windblown debris whose distribution changes with time. Bright polar caps are clearly visible through the telescope, and the larger size of the northern polar cap long has been recognized.
Most of the changes in appearance through the telescope are due to atmospheric effects of various kinds. Large arrays of white clouds commonly form in the middle latitudes and may persist for weeks. Those around the volcanic centers of Tharsis and Elysium most likely form when the air cools as it rises over the high volcanic regions. Other white clouds probably are caused by the daily recycling of water between the soil and the lower atmosphere. Frontal clouds and standing-wave clouds, seen clearly in spacecraft pictures, are probably not visible from the earth. During the fall thick clouds gather in the high latitudes to form polar hoods, which mask the growth of the polar caps. Brightening at the poles in this season is probably the result of both these clouds and the cap itself. Brightening in low areas such as Hellas and Argyre may also result from a combination of surface frost and clouds.
Whereas white clouds generally are brightest when observed in blue light, yellow clouds are brightest in yellow and orange. Yellow clouds occur mostly in the mid-southern latitudes at midsummer when large lateral and vertical temperature gradients cause extreme turbulence, which lifts large amounts of dust into the atmosphere. Activity generally starts in the region 320° W to 30° W and 30° S to 60° S and in most years spreads widely, so that ultimately the whole planet is engulfed in a gigantic dust storm. After the midsummer turbulence declines, dust slowly settles out of the atmosphere and the surface markings reappear. Global dust storms of this type were observed close up in 1971 by the Mariner 9 flyby space probe and in 1977 by the Viking orbiters (Squyres, p. 32).
No other aspect of Mars has aroused such widespread interest and controversy as the so-called canals. The controversy started in 1877 with the Italian astronomer Giovanni Schiaparelli’s publication of a map of Mars that showed many dark lines on the surface. These he called Canali, the Italian word for both “canal” and “channel.” In the ensuing decades, Mars observers were divided between those who claimed the canals existed and those who claimed they did not. The strongest proponent of the canals was the American astronomer Percival Lowell, who produced ever more intricate maps of linear markings based on observations at the observatory he founded in Flagstaff, Ariz. In a book published in 1908, he aroused considerable popular attention by suggesting that the markings were irrigation canals built by an advanced civilization. As better telescopes were built and instrumental measurements failed to confirm their existence, belief in the canals declined (Furniss, p. 68). The various space probes that have since visited Mars found no evidence for most of the lines on the early maps, with the result that most are now regarded as optical illusions.
Spacecraft Explorations
Most current knowledge of Mars is derived from space-probe observations, initially from the Mariner 9 and Viking missions. In 1965 the U.S. Mariner 4 flyby space probe returned the first close-up pictures of the planet, followed in 1969 by two additional flyby missions, Mariners 6 and 7. All three probes flew over the parts of the planet that most resemble the moon and presented a rather deceptive view of the planet as a moonlike body. The diverse geologic character of the Martian surface was not fully recognized until 1971. During that year Mariner 9 and two Soviet spacecraft, Mars 2 and 3, were placed in orbit around Mars. The Soviet spacecraft was short-lived, and their accompanying Landers failed to return useful data from the surface, but Mariner 9 continued to operate for a year, returning more than 7,000 pictures of the planet. (Paolo, 45) Additional photographs were obtained in 1974 by the Soviet vehicles Mars 4, 5, and 6. In 1976 two Viking spacecraft were placed in orbit around Mars, and two additional spacecraft landed on the surface. The Viking 2 and 1 orbiters continued to function, respectively, until 1978 and 1980, by which time they had taken over 50,000 pictures of the planet and returned a wealth of other data. Contact with the Viking 2 and 1 Landers was lost, respectively, in 1980 and 1982 (Squyres, p. 57).
After a 17-year hiatus in Mars exploration, the United States launched Mars Observer in 1992. Mission objectives were to study geology, geophysics, and climate of the red planet, but it ended in disappointment when contact was lost with the craft just before it entered Martian orbit. In 1996 Mars Pathfinder was launched to demonstrate that an unpiloted spacecraft could deliver and deploy a robotic rover. Not only was the mission a success, but also Pathfinder and its rover, Sojourner, returned unprecedented amounts of information including images, soil analyses, and wind measurements before their final data transmission in September 1997. The next two missions to Mars failed: Climate Orbiter burned up on entering Mars’s atmosphere in September 1999; and three months later Polar Lander and Deep Space 2 were lost on arrival.
These disappointments were followed by a spate of successes, beginning in 1997 when Mars Global Surveyor slipped into Martian orbit. For nine years the probe mapped the red planet returning dramatic evidence of hillside water flows before succumbing to battery failure in 2006. The Mars Odyssey spacecraft, launched in 2001, has captured more than 130,000 images and continues to transmit information about Martian geology, climate, and mineralogy. NASA joined with the European Space Agency and the Italian Space Agency for the Mars Express mission in 2003 (Paolo, p. 23). Despite losing Beagle 2, its land rover, Mars Express has provided information about various surface features, including buried impact craters, evidence of glacial activity, and the presence of methane gas. The pursuit of geological clues to the possibility of life on Mars continued with NASA’s land rovers Spirit and Opportunity, twin robotic vehicles that rolled off their airbag-encased Landers on opposite sides of Mars in 2004 (Furniss, p. 102). Sporting names selected from more than 10,000 entries in a student essay contest, the two solar-powered rovers have outlived their intended three-month mission and continue to transmit high-resolution, full-color images of Martian terrain, soil surfaces, and rocks. The Mars Reconnaissance Orbiter, launched in 2005, currently orbits high above the red planet, using a sounding device to search for subsurface water.
In May 2008 the Mars Reconnaissance Orbiter relayed photographs of the safe descent of the Phoenix Mars Lander as it parachuted onto the planet’s frozen North Pole. Daily instructions were sent from the earthbound control center, directing the Lander to collect soil samples from the icy surface. Phoenix used its robotic arm to deliver soil and ice samples to its onboard experiment platforms. The samples are to be analyzed in hopes of determining whether the location could have supported microbial life during the planet’s past.
General Physiography
Mars is markedly asymmetrical in the distribution of its surface features. Much of the Southern Hemisphere is heavily cratered like the lunar highlands and includes two large impact basins, Hellas and Argyre. In contrast, much of the Northern Hemisphere is covered with sparsely cratered plains. The planet has two major volcanic regions, the Tharsis region centered at 110° W on the equator and the Elysium region centered at 25° N, 212° W. Extending eastward from Tharsis are several large canyons that together makeup Valles Marineris, while east and north of the canyons are several huge dry riverbeds. The poles are distinctly different from the rest of the planet and appear to have thick deposits of layered sedimentary rocks exposed at the surface. The North Pole is also surrounded by extensive sand dunes.
Densely Cratered Terrain
This terrain is characterized by many large, relatively shallow craters; smooth intercrater plains; and a relatively small number of smaller craters (those less than 30 miles, or 50 km, in diameter). The terrain probably dates from very early in the planet’s history, possibly from 4 billion years ago, when the impact rate was higher than at present. The most extensive cratered areas are in the Southern Hemisphere. Fresh Martian craters differ markedly in appearance from those on the moon and Mercury. Most lunar craters are surrounded by disordered rubble-like ejecta that appears to have been deposited from ballistic trajectories. In contrast, the Martian craters are surrounded by ejecta that appears to have flowed along the ground. The fluid properties of the ejecta have been attributed to the presence in the Martian surface of large amounts of ground ice, which melts on impact and is incorporated into the ejecta. Crater examination by the Opportunity probe has revealed evidence of a watery and possibly habitable past on Mars.
Sparsely Cratered Plains
Plains cover much of the Northern Hemisphere and also occur within large impact basins such as Hellas and Argyre in the south. They are distinguished from the densely cratered terrain by the almost total absence of impact craters larger than 30 miles (50 km) in diameter. The plains have a different appearance in different areas. Around the large volcanoes in Tharsis and Elysium, the plains appear to be a thick succession of lava flows. In other areas, such as Chryse Planitia, where the Viking 1 spacecraft landed, the plains resemble those of the lunar maria, being relatively featureless except for impact craters and low winding ridges. These plains are probably also volcanic. The plains in the high northern latitudes have a variety of poorly understood features. Extensive areas have a polygonal fracture pattern, with individual polygons averaging 6 miles (10 km) across. In other areas are parallel linear markings, low escarpments, and intricate patterns of light and dark. Many of the unique characteristics of the northern plains have been attributed to repeated deposition and removal by the wind of layers of ice-rich debris. The number of impact craters on most of the plains, while considerably smaller than on the densely cratered terrain, is still sufficiently large to indicate an old age, probably in the range of 1 to 4 billion years. The only possible exceptions are the plains around the large volcanoes, which appear younger.
Volcanic and Tectonic Features
The large volcanoes of Tharsis are among the most spectacular features of the planet. The largest, Olympus Mons, is 15.5 miles (25 km) high and more than 340 miles (550 km) across at its base. Three other volcanoes in Tharsis reach approximately the same height. Each is topped by a central caldera, or crater, 50 to 75 miles (80 to 120 km) across, and on the flanks are numerous lava channels, lava tubes, flow fronts, and other features indicative of very fluid lava. Analysis of photographs transmitted by Odyssey in 2007 of the massive Arsia Mons volcano reveals seven black spots that scientists suspect are caves the size of football fields. If so, they would shield their contents from surface radiation and could potentially shelter life (Squyres, p. 78).
The style of volcanism on Mars is similar to that in Hawaii, except that the Martian features are ten times larger. The volcanoes are relatively young and may still be active. Tharsis is also the center of a set of fractures that occur over almost an entire hemisphere of the planet. They appear to have formed as a result of the loading of the crust by the Tharsis bulge.
Volcanoes also occur elsewhere on Mars, but they tend to be older and smaller than those in Tharsis. In 2007 the Spirit rover rolled onto evidence of an ancient volcanic explosion near its landing site dubbed “Home Plate” in Gusev Crater. Analysis revealed high chlorine content in the 2-meter- (6-foot) thick plateau of bedrock, suggesting that fluid basalt lava had come in contact with brine, indicating that water had been involved (Paolo, p. 59).
To the east of Tharsis and aligned along with the radial fractures are several enormous canyons. They stretch from the summit of the Tharsis bulge eastward for approximately 3,000 miles (5,000 km). Individual canyons are up to 125 miles (200 km) across and 1 to 4 miles (2 to 7 km) deep. The walls are steep and in many sections deeply gullied. In some parts, the walls have collapsed to form gigantic landslides several tens of miles across that have traveled more than 60 miles (100 km) along the canyon floor. The canyons are believed to have formed mainly by down faulting, followed by slumping and gullying of the walls (Furniss, p. 62).
Channels pose some of the more puzzling problems of Martian geology. Much of the densely cratered terrain is dissected by small channels that form drainage networks much like terrestrial river valleys. Liquid water, however,
- Furniss, Tim. The History of Space Exploration: And Its Future… Mercury Books London: 2005
- Paolo, Ulivi & Harland, David. Robotic Exploration of the Solar System. Springer Praxis Books: 2008
- Squyres, Steve. Roving Mars: Spirit, Opportunity, and the Exploration of the Red Planet Hyperion; Reprint edition: 2007
- Hubble Deep Field Video Image: The Most Important Image Man Has Ever Taken
- Extrasolar Planets and Search for Life
- Astronomy Exploration of Planets and Satellites in Comparison With the Earth
- “Mars the Abode of Life” by Percival Lowell
- Mercury Exploration and Space Missions
- The Status of Pluto Needs to Remain as a Planet
- Astronomy: Ancient History of Science
- Cosmology and Astronomical Observation
- Near-Earth Objects and Planetary Defences
- The Search for Life on Titan and Other Planets
- Chicago (A-D)
- Chicago (N-B)
IvyPanda. (2021, October 11). Mars: The Exploration of the Red Planet. https://ivypanda.com/essays/explorations-of-mars/
"Mars: The Exploration of the Red Planet." IvyPanda , 11 Oct. 2021, ivypanda.com/essays/explorations-of-mars/.
IvyPanda . (2021) 'Mars: The Exploration of the Red Planet'. 11 October.
IvyPanda . 2021. "Mars: The Exploration of the Red Planet." October 11, 2021. https://ivypanda.com/essays/explorations-of-mars/.
1. IvyPanda . "Mars: The Exploration of the Red Planet." October 11, 2021. https://ivypanda.com/essays/explorations-of-mars/.
Bibliography
IvyPanda . "Mars: The Exploration of the Red Planet." October 11, 2021. https://ivypanda.com/essays/explorations-of-mars/.
Home — Essay Samples — Science — Astronomy — Mars

Essays on Mars
There are so many interesting things to learn about Mars, making it a great topic for an essay. Whether you are writing for a school assignment or just want to expand your knowledge, writing an essay about Mars can be both fun and educational.
When choosing a topic for your Mars essay, think about what aspect of the planet interests you the most. You could explore the possibility of life on Mars, the history of Mars exploration, or the potential for future human colonization. There are so many different angles to consider when writing about Mars, so choose a topic that excites you.
If you're considering writing an argumentative essay about Mars, you could explore topics such as the ethical considerations of colonizing Mars, the scientific evidence for past or present life on Mars, or the potential benefits of investing in Mars exploration. For a cause and effect essay, you might consider topics such as the impact of climate change on Mars, the consequences of a successful Mars colonization mission, or the effects of Mars exploration on Earth's economy and technology.
For an opinion essay, you could share your thoughts on whether humans should attempt to colonize Mars, the potential risks and rewards of Mars exploration, or the ethical implications of terraforming Mars. And if you're interested in writing an informative essay, you could explore topics such as the geological features of Mars, the history of Mars exploration missions, or the challenges of living and working on Mars.
- " The potential for human colonization on Mars presents both exciting opportunities and ethical dilemmas. "
- " The search for evidence of past or present life on Mars has significant implications for our understanding of the universe. "
- " Mars exploration missions have provided valuable insights into the planet's climate, geology, and potential for sustaining life. "
- " As humans continue to push the boundaries of space exploration, the idea of colonizing Mars has captured the imagination of scientists, engineers, and aspiring astronauts around the world. "
- " The red planet, Mars, has long been a source of fascination and mystery for scientists and space enthusiasts alike. "
- " The exploration and potential colonization of Mars present both incredible opportunities and complex challenges for humanity. "
- " As we continue to learn more about the red planet, Mars, it is clear that our understanding of the universe and our place within it is forever changed. "
The Prospects and Challenges of Human Exploration on Mars
Why we shouldn't colonize mars, made-to-order essay as fast as you need it.
Each essay is customized to cater to your unique preferences
+ experts online
Challenges and Benefits of Manned Mission to Mars
The negative effect of chasing the dream of colonizing mars, mars, "the red planet": facts and information, spacex's intentions on landing humans on mars in 2022, let us write you an essay from scratch.
- 450+ experts on 30 subjects ready to help
- Custom essay delivered in as few as 3 hours
Analysis of The Mission to Colonize Mars
Strategies of creating a self-sustaining environment, the geohazards that astronauts may encounter on mars, different benefits of space exploration for humankind, get a personalized essay in under 3 hours.
Expert-written essays crafted with your exact needs in mind
Mars Exploration: All About The "Red Planet"
Robotic arm technology development for research and mars exploration, discussion of the history of space exploration and colonization, life on mars: an exploration of the possibility and implications, mars the red planet, relevant topics.
- Space Exploration
- Neil Armstrong
- Big Bang Theory
- Natural Selection
- Mathematics in Everyday Life
By clicking “Check Writers’ Offers”, you agree to our terms of service and privacy policy . We’ll occasionally send you promo and account related email
No need to pay just yet!
We use cookies to personalyze your web-site experience. By continuing we’ll assume you board with our cookie policy .
- Instructions Followed To The Letter
- Deadlines Met At Every Stage
- Unique And Plagiarism Free

100+ Space Research Topics [Updated]
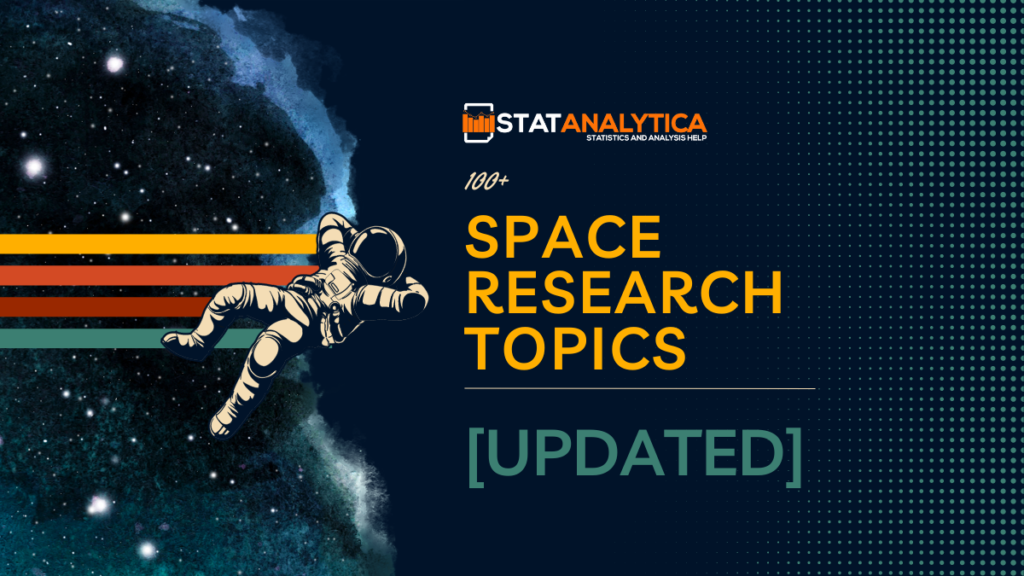
Space has always attracted humanity’s imagination. The vastness of the cosmos, with its twinkling stars, mysterious planets, and enigmatic black holes, beckons us to explore its depths. But why do we study space? What are the research topics that drive scientists to reach for the stars? In this blog, we’ll delve into the fascinating world of space research topics, exploring key topics that continue to inspire and challenge researchers around the globe.
Why Do We Study Space?
Table of Contents
Here are some key points explaining why we study space:
- Understanding our Origins: Space research helps us uncover the origins of the universe, including how galaxies, stars, and planets formed.
- Advancing Scientific Knowledge: Studying space leads to breakthroughs in physics, astronomy, and other scientific fields, expanding our understanding of the cosmos.
- Technological Innovation: Space exploration drives the development of new technologies, such as satellite communication and medical imaging, benefiting society as a whole.
- Exploration and Discovery: Humans are inherently curious, and space offers a vast frontier for exploration, fueling our desire to discover new worlds and phenomena.
- Earth Observation: Space-based observations provide valuable data on Earth’s climate, weather patterns, and environmental changes, aiding in disaster management and conservation efforts.
- Search for Life: Investigating other planets and celestial bodies helps us understand the conditions necessary for life, potentially leading to the discovery of extraterrestrial life forms.
- Inspiration and Education: Space exploration inspires future generations of scientists, engineers, and explorers, fostering innovation and curiosity about the universe.
100+ Space Research Topics: Category Wise
Astronomy and astrophysics.
- Exoplanet detection methods and recent discoveries
- The life cycle of stars: from birth to death
- Supermassive black holes and their role in galaxy formation
- Gravitational waves: detection and implications
- Dark matter and dark energy: understanding the mysteries of the universe
- Supernovae explosions: studying the aftermath of stellar deaths
- Galactic dynamics: exploring the structure and evolution of galaxies
- Cosmic microwave background radiation: insights into the early universe
- Gamma-ray bursts: uncovering the most energetic explosions in the cosmos
- The search for extrasolar planets with potential habitable conditions
Planetary Science
- Martian geology and the search for signs of past life
- Jupiter’s Great Red Spot: dynamics and longevity
- Saturn’s rings: composition, structure, and origin
- Lunar exploration: past missions and future prospects
- Venusian atmosphere: understanding the greenhouse effect and extreme conditions
- Io, Europa, Ganymede, and Callisto: Jupiter’s diverse moons
- Titan: Saturn’s moon with an Earth-like atmosphere and hydrocarbon lakes
- The Kuiper Belt and Oort Cloud: reservoirs of comets and icy bodies
- Dwarf planets: Pluto, Eris, Haumea, Makemake, and Ceres
- Planetary volcanism: processes and consequences on various celestial bodies
Space Technology and Engineering
- Satellite constellations for global internet coverage
- CubeSats: miniaturized satellites for scientific research and technology demonstration
- Space debris mitigation strategies and technologies
- Ion propulsion systems: efficient propulsion for deep space missions
- Space telescopes: next-generation observatories for astronomy and astrophysics
- Space-based solar power: harvesting solar energy in orbit
- Asteroid mining: extracting resources from near-Earth objects
- In-situ resource utilization on other planets and moons
- Additive manufacturing (3D printing) in space exploration
- Autonomous spacecraft navigation and control for long-duration missions
Astrobiology and the Search for Life
- Extremophiles: organisms thriving in extreme environments on Earth and their implications for extraterrestrial life
- Biosignatures: markers of past or present life on other planets
- Methanogenesis on Mars: potential evidence for subsurface microbial life
- Europa’s subsurface ocean: exploring the possibility of life beneath the ice
- Enceladus: hydrothermal vents and the search for life in its subsurface ocean
- The habitability of exoplanets: assessing conditions for life beyond the solar system
- Panspermia: the transfer of life between celestial bodies
- Astrobiology field research in extreme environments on Earth
- SETI: the search for extraterrestrial intelligence and communication
- The implications of discovering microbial life on Mars or other celestial bodies
Space Policy and Ethics
- International collaboration in space exploration and research
- The Outer Space Treaty: principles governing the use of outer space
- Space tourism regulations and safety considerations
- Space law and jurisdiction: legal frameworks for activities in space
- Military applications of space technology and potential arms race in space
- Space resource utilization and ownership rights
- Space environmentalism: advocating for the protection of celestial bodies and their environments
- Space colonization ethics and implications for human societies
- Space governance beyond national boundaries
- Cultural heritage preservation on the Moon and other celestial bodies
Challenges and Future Directions
- Funding challenges and opportunities in space research and exploration
- Space radiation hazards and mitigation strategies for astronauts
- Interplanetary communication and navigation for deep space missions
- Long-duration spaceflight: physiological and psychological effects on astronauts
- Terraforming Mars: engineering a habitable environment on the Red Planet
- Space elevator concept: a revolutionary approach to space access
- Next-generation space launch vehicles and propulsion technologies
- Nuclear propulsion for crewed missions to Mars and beyond
- Space settlement design and infrastructure requirements
- Advancing artificial intelligence and robotics for autonomous space exploration
Space Weather and Space Environment
- Solar flares and coronal mass ejections: impacts on Earth’s magnetosphere and technology
- Space weather forecasting and its applications in satellite operations
- Magnetospheres of Earth and other planets: comparative studies and dynamics
- Solar wind interactions with planetary atmospheres and magnetospheres
- Aurora phenomena on Earth and other planets
- Radiation belts: understanding and mitigating hazards for spacecraft and astronauts
- Cosmic rays: sources, composition, and effects on space missions
- Space climate change: long-term variations in solar activity and their consequences
- Space weather effects on satellite communications, navigation, and power systems
- Space weather monitoring and prediction networks
Space Exploration and Missions
- Mars Sample Return mission: challenges and scientific objectives
- Artemis program: NASA’s plans for returning astronauts to the Moon
- Asteroid impact mitigation strategies and planetary defense initiatives
- The James Webb Space Telescope: capabilities and scientific goals
- Europa Clipper mission: exploring Jupiter’s icy moon for signs of habitability
- China’s Chang’e lunar exploration program: past achievements and future missions
- Commercial crew and cargo transportation to the International Space Station
- Voyager and Pioneer missions: the farthest human-made objects in space
- Space missions to study near-Earth objects and potential asteroid mining targets
- International Mars exploration collaborations and missions
Space Communication and Navigation
- Deep space communication networks and relay satellites
- Laser communication technology for high-speed data transmission in space
- Satellite-based navigation systems: GPS, Galileo, and GLONASS
- Interplanetary Internet: protocols and architectures for space communications
- Radio astronomy and interferometry: probing the universe with radio waves
- Quantum communication in space: secure and ultra-fast communication channels
- Delay-tolerant networking for deep space missions
- Autonomous navigation systems for spacecraft and rovers
- Optical communications for small satellites and CubeSats
- Space-to-ground communication systems for remote sensing and Earth observation satellites
Space Medicine and Human Spaceflight
- Microgravity effects on human physiology and health
- Countermeasures for mitigating bone and muscle loss in space
- Psychological challenges of long-duration space missions
- Space food technology: nutrition and sustainability in space
- Medical emergencies in space: protocols and procedures for astronaut health care
- Radiation shielding and protection for crewed missions beyond Earth orbit
- Sleep and circadian rhythms in space: optimizing astronaut performance
- Artificial gravity concepts for maintaining crew health on long-duration missions
- Telemedicine applications for space exploration missions
- Bioastronautics research: advancing human spaceflight capabilities and safety
Space Industry and Commercialization
- NewSpace companies: the rise of private space exploration ventures
- Satellite constellation deployments for global internet coverage
- Space tourism: opportunities, challenges, and market trends
- Commercial spaceports and launch facilities around the world
- Space manufacturing and in-space assembly techniques
Tips To Write Space Research Papers
Crafting space research papers can be a thrilling and fulfilling pursuit, yet it demands meticulous planning and implementation to guarantee that your efforts effectively convey your discoveries and make meaningful contributions to the discipline. Here are some tips to help you write space research papers:
- Choose a Narrow Topic: Space is a vast field with numerous sub-disciplines. Narrow down your topic to something specific and manageable, ensuring that it aligns with your interests and expertise.
- Conduct Thorough Research: Before you start writing, immerse yourself in the existing literature on your chosen topic. Familiarize yourself with key concepts, theories, and recent discoveries to provide context for your research.
- Develop a Clear Thesis Statement: Define the central argument or hypothesis of your paper in a concise and focused thesis statement. This statement should guide your writing and serve as the foundation for your research.
- Outline Your Paper: Create a detailed outline outlining the structure of your paper, including the introduction, literature review, results, and conclusion sections. This will help you organize your thoughts and ensure that your paper flows logically.
- Write a Compelling Introduction: Begin your paper with a captivating introduction that offers context about your subject, underscores its importance, and delineates the paper’s framework . Grab the reader’s interest and inspire them to delve further into your work.
- Provide a Comprehensive Literature Review: Synthesize the existing research on your topic in a literature review section. Examine pertinent research, theories, methodologies, and results, pinpointing any disparities or deficiencies in the existing literature that your study seeks to rectify.
- Detail Your Methodology: Describe the methods you used to conduct your research, including data collection, analysis, and interpretation techniques. Provide enough detail for readers to understand how your study was conducted and to evaluate its validity and reliability.
- Present Your Results Clearly: Present your research findings in a clear, concise manner, using tables, figures, and charts to illustrate key data points. Interpret your results objectively and discuss their implications in relation to your research question or hypothesis.
- Engage in Critical Analysis: Analyze your findings in the context of existing literature, discussing their significance, strengths, limitations, and potential implications for future research. Be critical and objective in your evaluation, acknowledging any potential biases or limitations in your study.
- Craft a Strong Conclusion: Summarize the main findings of your research and reiterate their significance in the conclusion section. Discuss any implications for theory, practice, or policy and suggest avenues for future research.
- Proofread and Revise: Before submitting your paper, carefully proofread it for spelling, grammar, and punctuation errors. Edit your writing to ensure clarity, coherence, and consistency, guaranteeing that your points are adequately backed and logically organized.
- Follow Formatting Guidelines: Follow the formatting instructions provided by the journal or conference to which you intend to submit your paper. Pay attention to details such as font size, margins, citation style, and reference formatting to ensure that your paper meets the publication requirements.
Space research offers a window into the vastness of the cosmos, revealing the beauty and complexity of the universe we inhabit. From the depths of space to the surfaces of distant planets, scientists are uncovering new wonders and answering age-old questions about our place in the universe. As we look to the stars, let us be inspired by the spirit of exploration and discovery that drives humanity ever onward, towards new horizons and unknown worlds. I hope you find the best space research topics from the above list.
Related Posts
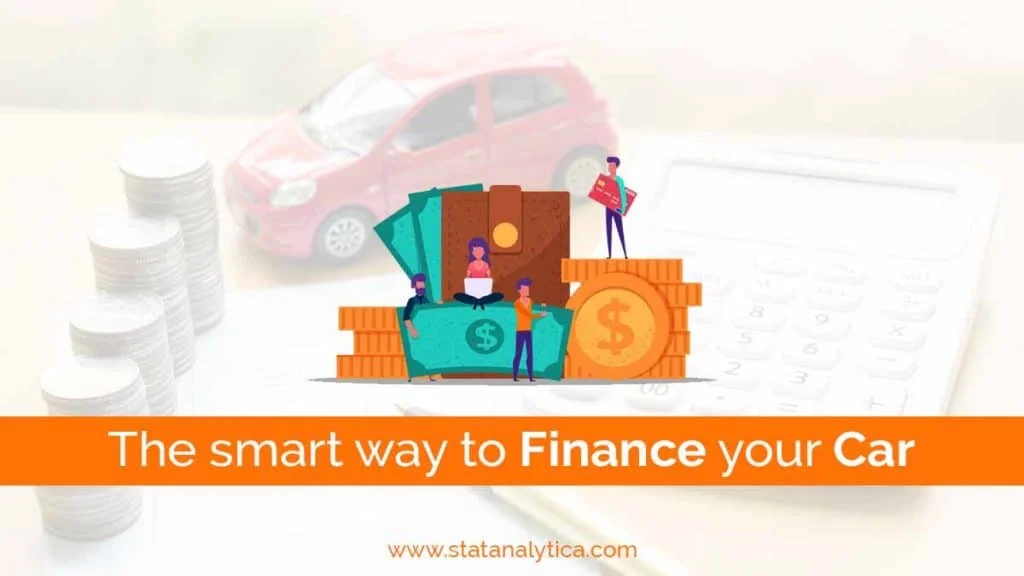
Step by Step Guide on The Best Way to Finance Car
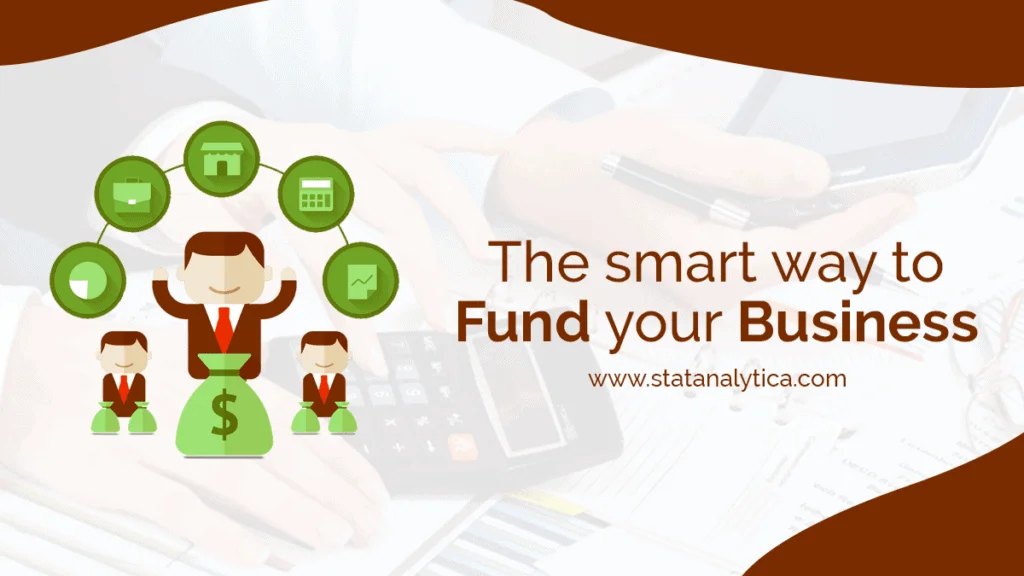
The Best Way on How to Get Fund For Business to Grow it Efficiently
Suggestions or feedback?
MIT News | Massachusetts Institute of Technology
- Machine learning
- Sustainability
- Black holes
- Classes and programs
Departments
- Aeronautics and Astronautics
- Brain and Cognitive Sciences
- Architecture
- Political Science
- Mechanical Engineering
Centers, Labs, & Programs
- Abdul Latif Jameel Poverty Action Lab (J-PAL)
- Picower Institute for Learning and Memory
- Lincoln Laboratory
- School of Architecture + Planning
- School of Engineering
- School of Humanities, Arts, and Social Sciences
- Sloan School of Management
- School of Science
- MIT Schwarzman College of Computing
New transistor’s superlative properties could have broad electronics applications
Press contact :.
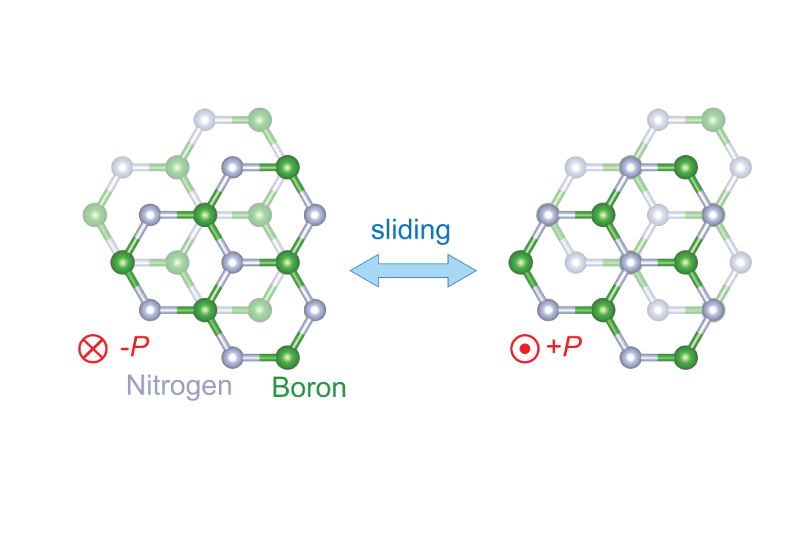
Previous image Next image
In 2021, a team led by MIT physicists reported creating a new ultrathin ferroelectric material, or one where positive and negative charges separate into different layers. At the time they noted the material’s potential for applications in computer memory and much more. Now the same core team and colleagues — including two from the lab next door — have built a transistor with that material and shown that its properties are so useful that it could change the world of electronics.
Although the team’s results are based on a single transistor in the lab, “in several aspects its properties already meet or exceed industry standards” for the ferroelectric transistors produced today, says Pablo Jarillo-Herrero, the Cecil and Ida Green Professor of Physics, who led the work with professor of physics Raymond Ashoori. Both are also affiliated with the Materials Research Laboratory.
“In my lab we primarily do fundamental physics. This is one of the first, and perhaps most dramatic, examples of how very basic science has led to something that could have a major impact on applications,” Jarillo-Herrero says.
Says Ashoori, “When I think of my whole career in physics, this is the work that I think 10 to 20 years from now could change the world.”
Among the new transistor’s superlative properties:
- It can switch between positive and negative charges — essentially the ones and zeros of digital information — at very high speeds, on nanosecond time scales. (A nanosecond is a billionth of a second.)
- It is extremely tough. After 100 billion switches it still worked with no signs of degradation.
- The material behind the magic is only billionths of a meter thick, one of the thinnest of its kind in the world. That, in turn, could allow for much denser computer memory storage. It could also lead to much more energy-efficient transistors because the voltage required for switching scales with material thickness. (Ultrathin equals ultralow voltages.)
The work is reported in a recent issue of Science . The co-first authors of the paper are Kenji Yasuda, now an assistant professor at Cornell University, and Evan Zalys-Geller, now at Atom Computing. Additional authors are Xirui Wang, an MIT graduate student in physics; Daniel Bennett and Efthimios Kaxiras of Harvard University; Suraj S. Cheema, an assistant professor in MIT’s Department of Electrical Engineering and Computer Science and an affiliate of the Research Laboratory of Electronics; and Kenji Watanabe and Takashi Taniguchi of the National Institute for Materials Science in Japan.
What they did
In a ferroelectric material, positive and negative charges spontaneously head to different sides, or poles. Upon the application of an external electric field, those charges switch sides, reversing the polarization. Switching the polarization can be used to encode digital information, and that information will be nonvolatile, or stable over time. It won’t change unless an electric field is applied. For a ferroelectric to have broad application to electronics, all of this needs to happen at room temperature.
The new ferroelectric material reported in Science in 2021 is based on atomically thin sheets of boron nitride that are stacked parallel to each other, a configuration that doesn’t exist in nature. In bulk boron nitride, the individual layers of boron nitride are instead rotated by 180 degrees.
It turns out that when an electric field is applied to this parallel stacked configuration, one layer of the new boron nitride material slides over the other, slightly changing the positions of the boron and nitrogen atoms. For example, imagine that each of your hands is composed of only one layer of cells. The new phenomenon is akin to pressing your hands together then slightly shifting one above the other.
“So the miracle is that by sliding the two layers a few angstroms, you end up with radically different electronics,” says Ashoori. The diameter of an atom is about 1 angstrom.
Another miracle: “nothing wears out in the sliding,” Ashoori continues. That’s why the new transistor could be switched 100 billion times without degrading. Compare that to the memory in a flash drive made with conventional materials. “Each time you write and erase a flash memory, you get some degradation,” says Ashoori. “Over time, it wears out, which means that you have to use some very sophisticated methods for distributing where you’re reading and writing on the chip.” The new material could make those steps obsolete.
A collaborative effort
Yasuda, the co-first author of the current Science paper, applauds the collaborations involved in the work. Among them, “we [Jarillo-Herrero’s team] made the material and, together with Ray [Ashoori] and [co-first author] Evan [Zalys-Geller], we measured its characteristics in detail. That was very exciting.” Says Ashoori, “many of the techniques in my lab just naturally applied to work that was going on in the lab next door. It’s been a lot of fun.”
Ashoori notes that “there’s a lot of interesting physics behind this” that could be explored. For example, “if you think about the two layers sliding past each other, where does that sliding start?” In addition, says Yasuda, could the ferroelectricity be triggered with something other than electricity, like an optical pulse? And is there a fundamental limit to the amount of switches the material can make?
Challenges remain. For example, the current way of producing the new ferroelectrics is difficult and not conducive to mass manufacturing. “We made a single transistor as a demonstration. If people could grow these materials on the wafer scale, we could create many, many more,” says Yasuda. He notes that different groups are already working to that end.
Concludes Ashoori, “There are a few problems. But if you solve them, this material fits in so many ways into potential future electronics. It’s very exciting.”
This work was supported by the U.S. Army Research Office, the MIT/Microsystems Technology Laboratories Samsung Semiconductor Research Fund, the U.S. National Science Foundation, the Gordon and Betty Moore Foundation, the Ramon Areces Foundation, the Basic Energy Sciences program of the U.S. Department of Energy, the Japan Society for the Promotion of Science, and the Ministry of Education, Culture, Sports, Science and Technology (MEXT) of Japan.
Share this news article on:
Related links.
- Raymond Ashoori
- Ashoori Group @ MIT
- Pablo Jarillo-Herrero
- Jarillo-Herrero Group
- Materials Research Laboratory
- Department of Physics
Related Topics
- Materials science and engineering
- Electronics
- Computer science and technology
- Research Laboratory of Electronics
- Electrical Engineering & Computer Science (eecs)
Related Articles
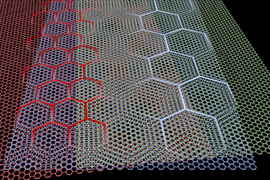
Physicists coax superconductivity and more from quasicrystals
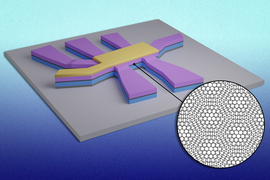
Study: Superconductivity switches on and off in “magic-angle” graphene
Physicists engineer ferroelectricity in boron nitride
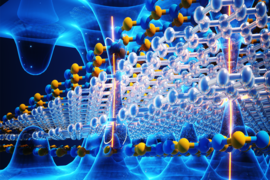
Physicists discover important new property for graphene
Previous item Next item
More MIT News

Edgerton Center hosts workshop for deaf high school students in STEM
Read full story →
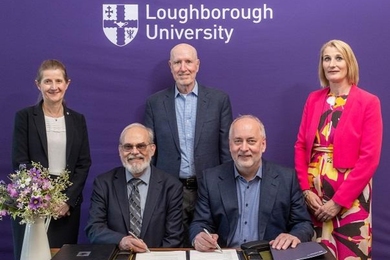
MIT Global SCALE Network expands by adding center at Loughborough University
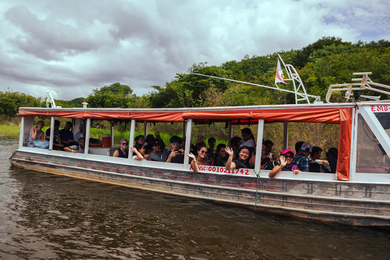
When learning at MIT means studying thousands of miles away
Flying high to enable sustainable delivery, remote care
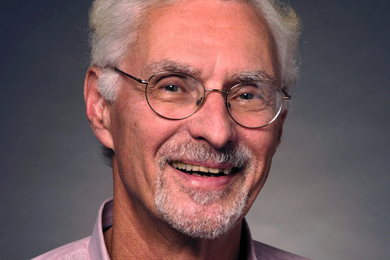
Professor Emeritus Ralph Gakenheimer, mobility planner and champion of international development, dies at 89
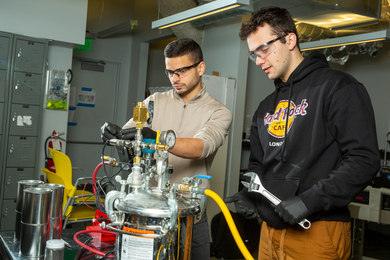
A recipe for zero-emissions fuel: Soda cans, seawater, and caffeine
- More news on MIT News homepage →
Massachusetts Institute of Technology 77 Massachusetts Avenue, Cambridge, MA, USA
- Map (opens in new window)
- Events (opens in new window)
- People (opens in new window)
- Careers (opens in new window)
- Accessibility
- Social Media Hub
- MIT on Facebook
- MIT on YouTube
- MIT on Instagram
Get the Reddit app
The scientific study of the origin, history, and structure of the Earth.
Research Paper Ideas about Mars
Hello r/Geology !
For one of my classes, I have to do a research paper and PowerPoint presentation on a particular topic. The paper is 5-8 pages, double spaced and I can have pictures within the pages. PowerPoint doesn't really have a limit.
I am going to do my paper on something regarding the geology of mars, but I am not sure what about yet. I was thinking about observing something about the Valles Marineris, or something with the Gale Crater.
If you guys have ANY suggestions, please let me know. I am open to any and all ideas! Could be about climate conditions, geology, ANYTHING relating to geology really.
P.S. This is an undergraduate intro level class so obviously the level of complexity isn't Grad school level or Ph.D. thesis level.
By continuing, you agree to our User Agreement and acknowledge that you understand the Privacy Policy .
Enter the 6-digit code from your authenticator app
You’ve set up two-factor authentication for this account.
Enter a 6-digit backup code
Create your username and password.
Reddit is anonymous, so your username is what you’ll go by here. Choose wisely—because once you get a name, you can’t change it.
Reset your password
Enter your email address or username and we’ll send you a link to reset your password
Check your inbox
An email with a link to reset your password was sent to the email address associated with your account
Choose a Reddit account to continue

IMAGES
VIDEO
COMMENTS
Three spacecraft from three different nations arrived at Mars in February 2021. Two of those nations are newcomers to Mars and the third successfully set out the path for a Mars sample return.
Scientists from around the world are gathering this week in California to take stock of the state of science from Mars. Here are 10 recent significant events that got scientists talking ... but further research is needed. ... Discover More Topics From NASA. Perseverance Science. Curiosity Rover Science. MRO Science. Mars Odyssey Science.
After thorough review, all the accepted papers in the present Research Topic were novel, comprehensive, and informative with emphasis on the systematic and recent advances in our knowledge, tools, techniques, and methods for Mars research and exploration.
Planet Mars News. Read astronomy articles on how Mars could have once supported life, the Mars Rover and more. See images and read full-text articles on Mars exploration.
Here we present a probabilistic assessment of Mars' Noachian habitability to H 2 -based methanogens and quantify their biological feedback on Mars' atmosphere and climate. We find that ...
The Mars 2020 Perseverance mission is part of NASA's Moon to Mars exploration approach, which includes Artemis missions to the Moon that will help prepare for human exploration of the Red Planet. NASA's Jet Propulsion Laboratory, which is managed for the agency by Caltech, built and manages operations of the Perseverance rover.
Searching for evidence of life on Mars is a major impetus for exploration. A new study published in Nature Communications finds that current Mars mission instruments lack the essential sensitivity ...
The interest towards Mars research and exploration has gained significant momentum in the past three decades owing to the advances in computing, hardware, remote sensors, and general awareness. The next stages of this exploration demand more multidisciplinary efforts to effectively use the vast planetary data being gathered using various platforms and techniques. Our Research Topic aims to ...
Curiosity's scientific tools found chemical and mineral evidence of past habitable environments on Mars. It continues to explore the rock record from a time when Mars could have been home to microbial life.
The Mars 2020 Perseverance mission is part of NASA's Moon to Mars exploration approach, which includes Artemis missions to the Moon that will help prepare for human exploration of the Red Planet. JPL, which is managed for NASA by Caltech in Pasadena, California, built and manages operations of the Perseverance rover.
The NASA Mars rover examined a rock containing organic compounds and "leopard spots" that, on Earth, are associated with microbial life.
Finally, we propose dust storm monitoring and predicting for the upcoming Chinese Tianwen-3 Mars sample return mission. It concludes by depicting the future research topics aimed at systematically understanding Martian dust storms.
The Mars 2020 Perseverance rover landed in Jezero crater on 18 February 2021. After a 100-sol period of commissioning and the Ingenuity Helicopter technology demonstration, Perseverance began its first science campaign to explore the enigmatic Jezero crater floor, whose igneous or sedimentary origins have been much debated in the scientific community. This paper describes the campaign plan ...
NASA selected a new team of four research volunteers to participate in a simulated mission to Mars within HERA (Human Exploration Research Analog) at the agency's Johnson Space Center in Houston. Erin Anderson, Sergii Iakymov, Brandon Kent, and Sarah Elizabeth McCandless will begin their simulated trek to Mars on Friday, Aug. 9.
NASA says it found possible signs of life on Mars. There are a lot of maybes Some see hype over intriguing rock as plea for troubled multibillion-dollar Mars Sample Return mission
NASA's search for life on Mars: a rocky road for its rovers, a long slog for scientists - and back on Earth, a battle of the budget. Amy J. Williams, University of Florida. Determining whether ...
Mars residual north polar cap: Earth‐based spectroscopic confirmation of water ice as a major constituent and evidence for hydrated minerals. Journal of Geophysical Research: Solid Earth, 87(B1), 367-370.
This paper will compile research objectives relevant to a Martian presence in an attempt to create a coherent justification for human expeditions to Mars. It will organize these objectives in a ...
Astronomers have published a step-by-step guide to unlock the potential of NASA's James Webb Space Telescope for exoplanet research. It describes how to make data gathering more efficient and to account for stellar contamination.
TECHNICAL PUBLICATION. Reports of completed research or a major significant phase of research that present the results of NASA Programs and include extensive data or theoretical analysis. Includes compilations of significant scientific and technical data and information deemed to be of continuing reference value. NASA counterpart of peer-reviewed formal professional papers but has less ...
Mars: Among other potential outposts, the Red Planet has always been shrouded by a veil of romanticism and mystery. Beyond an active target for space exploration, colonization of Mars has become a popular topic nowadays, fuelled by a potentially naive and somewhat questionable belief that this planet could at some point in time be terraformed to sustain human life. 1 Indeed, the Moon, while ...
1998, Advances in Space Research. It is possible in the future that Mars might be transformed into a habitable planet by a process of global environmental engineering known as terraforming. This paper provides a thumb-nail sketch of the terraforming concepts that have appeared in the technical literature, focussing on the steps required in ...
View Life on Mars Research Papers on Academia.edu for free.
Mars, the fourth planet in order of increasing distance from the sun and the first beyond the earth's orbit. Under favorable conditions, it appears in the night sky as a yellowish red object (hence the name "red planet") of the first magnitude. Mars has long fascinated us because of its many similarities to the earth and because of the ...
Absolutely FREE essays on Mars. All examples of topics, summaries were provided by straight-A students. Get an idea for your paper
Explore the wonders of 100+ space research topics, from exoplanets to astrobiology. Join us on a journey to unlock the mysteries of the cosmos!
At Mars, we're fostering diverse scientific thought and exploration, strengthening traditional R&D with emerging capabilities like AI, leveraging computational science to revolutionize the way we approach science and research, and using genomics and sensomics to improve human and pet health.
Propofol, a drug commonly used for general anesthesia, induces unconsciousness by disrupting the brain's normal balance between stability and excitability, according to a new study by MIT researchers.
A new transistor's superlative properties could change electronics. The ultrathin material it is made of, developed by MIT's Materials Research Laboratory, enables superfast switching and extreme durability.
Hello r/Geology ! For one of my classes, I have to do a research paper and PowerPoint presentation on a particular topic. The paper is 5-8 pages…