What is a tardigrade?
Tardigrades are microscopic eight-legged animals that have been to outer space and would likely survive the apocalypse . Bonus: They look like adorable miniature bears.
Around 1,300 species of tardigrades are found worldwide. Considered aquatic because they require a thin layer of water around their bodies to prevent dehydration , they’ve also been observed in all kinds of environments, from the deep sea to sand dunes. Freshwater mosses and lichens are their preferred habitat, hence their nickname, moss piglet.
Despite looking squishy, tardigrades are covered in a tough cuticle, similar to the exoskeletons of grasshoppers, praying mantises, and other insects to which they are related. Like those insects, tardigrades have to shed their cuticles in order to grow. They have four to six claws on each foot, which helps them cling to plant matter, and a specialized mouthpart called a bucco pharyngeal apparatus, which allows them to suck nutrients from plants and microorganisms.

Tiny and tough
Tardigrades belong to an elite category of animals known as extremophiles , or critters that can survive environments that most others can't. For instance, tardigrades can go up to 30 years without food or water. They can also live at temperatures as cold as absolute zero or above boiling, at pressures six times that of the ocean’s deepest trenches, and in t he vacuum of space .
Their resiliency is in part due to a unique protein in their bodies called Dsup—short for "damage suppressor"—that protects their DNA from being harmed by things like ionizing radiation, which is present in soil, water, and vegetation.
Another amazing survival trick is cryptobiosis, a state of inactivity triggered by a dry environment. The micro-animals squeeze all the water out of their bodies, retract their heads and limbs, roll up into a little ball, and become dormant. When conditions improve, they unfurl themselves and go about their business.
Mating and reproduction
Naturally, tardigrades have unusual mating habits, too. Depending on the species, the animals may reproduce asexually or sexually. In some species, males deposit sperm inside the cuticle of a molting, egg-carrying female during an hour-long mating process . Some females shed their cuticle and then lay their eggs inside to be fertilized later by males.
Eggs take around 40 days to hatch, or as long as 90 days if they’ve been in a desiccated state.
DID YOU KNOW
Tardigrades have been on earth about 600 million years, preceding the dinosaurs by about 400 million years.
— American Museum of Natural History
They were first described in 1773 by German pastor J.A.E. Goeze, who called them kleiner Wasserbär , or “little water bear."
— SQ Online
The Israeli spacecraft Beresheet was carrying thousands of tardigrades when it crash-landed on the moon in April 2019. Later experiments show the animals likely died on impact.
- Environment
History & Culture
- History & Culture
- Mind, Body, Wonder
- Paid Content
- Terms of Use
- Privacy Policy
- Your US State Privacy Rights
- Children's Online Privacy Policy
- Interest-Based Ads
- About Nielsen Measurement
- Do Not Sell or Share My Personal Information
- Nat Geo Home
- Attend a Live Event
- Book a Trip
- Inspire Your Kids
- Shop Nat Geo
- Visit the D.C. Museum
- Learn About Our Impact
- Support Our Mission
- Advertise With Us
- Customer Service
- Renew Subscription
- Manage Your Subscription
- Work at Nat Geo
- Sign Up for Our Newsletters
- Contribute to Protect the Planet
Copyright © 1996-2015 National Geographic Society Copyright © 2015-2024 National Geographic Partners, LLC. All rights reserved
Water Bears Can Survive Impact Speeds of 1,845 Miles Per Hour
Tardigrades thrive in a variety of extreme conditions, so researchers wanted to know if they could withstand simulated space landing impacts
/https://tf-cmsv2-smithsonianmag-media.s3.amazonaws.com/accounts/headshot/gamillo007710829-005_0.png)
Elizabeth Gamillo
Daily Correspondent
:focal(771x531:772x532)/https://tf-cmsv2-smithsonianmag-media.s3.amazonaws.com/filer/70/3c/703c5c0c-3976-48e0-bfbf-f3781dadb704/sem_image_of_milnesium_tardigradum_in_active_state_-_journalpone0045682g001-2.png)
Tardigrades, also known as water bears, are hardy life forms that can survive extreme temperatures—from volcanic vents on the ocean floor to the frigid climes of Antarctica. The microscopic organism can also resist the vacuum of space and lethal doses of radiation, reports Jonathan O'Callaghan for Science .
To further test the water bear's survival limits, researchers loaded the microscopic beings into a gun and fired them at sand bag targets to test their impact survival rate, according to a study published in Astrobiology . It turns out, tardigrades can survive the violent impacts, but only to a certain point before they begin to fall apart. It could be the first step in exploring whether life can be distributed to other planets via asteroids—if the impact doesn't kill the lifeform first.
For years, scientists have speculated the possibility of panspermia , or microscopic lifeforms arriving from one planet to another via meteorites or comets, reports Becky Ferreira for Vice . Panspermia may potentially explain how life began on Earth. It could also determine whether a similar redistribution of life could happen by the same method on other hospitable planets.
In August 2019, Israel's lunar lander, Beresheet, crashed into the moon's surface while carrying thousands of tardigrades. Since then, researchers have wondered if the water bears survived the impact, reports Victor Tangermann for Futurism . With this event in mind, astrochemist Alejandra Traspas and astrophysicist Mark Burchell, who both work at the University of Kent, set out to find if the water bear's impact survival was possible.
They put the theory to the test by shooting tardigrades out of a lab-grade, two-stage, light-gas gun , which resembles a canon more than a gun. (A similar machine at NASA has a 24-foot-long "barrel" aimed at a target located 175 feet away. IT can shoot projectiles at speeds of 23,000 feet per second, or about four miles per second.) The "gun" in the study does use traditional gun powder and pressurized hydrogen or helium to fire at high speeds up to five miles per second, Futurism reports.
Before the experiment, the research team fed 20 freshwater tardigrades, Hypsibius dujardini , a diet of moss and mineral water before freezing them for two days, Science reports. Freezing the water bears places them into a "tun" state, sort of like hibernate. Once frozen, the microscopic organisms were placed into hollow nylon bullets and fired towards a sand target at velocities higher than a handgun can reach, Science reports.
Then, the water bears were collected from the target, poured into a water column, and observed to see how long it took them to wake from the hibernation state, reports Michelle Starr for Science Alert. Researchers found the bears could withstand an impact of up to 900 meters per second and shock pressures of up to 1.14 gigapascals. At higher speeds, the seemingly invincible water bears turned to mush.
The study results show that tardigrades can survive impact speeds of around 1,845 miles per hour. Still, meteorites that crash into other planets have higher shock pressures than those tested in the experiment, Vice reports, which means that tardigrades most likely won't survive an impact. However, some meteorites that hit Earth or Mars may experience lower shock pressures that a water bear could survive, Traspas explained to Science .
While the findings didn't conclude if the water bears survived the crash on the moon, the study's researchers said their conclusions determine how researchers can safely collect lifeforms from other planets without turning them to mush like the water bears.
The study also explained how researchers might detect life during flybys of Saturn's moon Enceladus and Jupiter's moon Europa. Both moon's eject plumes of saltwater that may or may not contain lifeforms, Vice reports. Overall, researchers may be one step closer to finding how life began on Earth. All thanks to a gun that fires plump water bears.
Get the latest stories in your inbox every weekday.
/https://tf-cmsv2-smithsonianmag-media.s3.amazonaws.com/accounts/headshot/gamillo007710829-005_0.png)
Elizabeth Gamillo | | READ MORE
Elizabeth Gamillo is a daily correspondent for Smithsonian and a science journalist based in Milwaukee, Wisconsin. She has written for Science magazine as their 2018 AAAS Diverse Voices in Science Journalism Intern.
- Skip to main content
- Keyboard shortcuts for audio player
Why NASA Is Blasting Water Bears And Bobtail Squid Into Space
Joe Hernandez

Scientists will study whether microgravity has an impact on the relationship between newly hatched bobtail squid and their symbiotic bacterium. Jamie S. Foster/University of Florida hide caption
Scientists will study whether microgravity has an impact on the relationship between newly hatched bobtail squid and their symbiotic bacterium.
When SpaceX makes its 22nd resupply mission to the International Space Station on Thursday, it will be carrying two very special guest species: water bears and bobtail squid.
The animals are being launched into the cosmos in the name of science, as NASA researchers attempt to learn more about how the conditions of spaceflight can affect biological organisms and, by extension, future astronauts.
Water bears: basically indestructible
Tardigrades are microscopic organisms better known as "water bears" because of their shape and the fact that they commonly live in the water. (They have also been called, endearingly, "moss piglets.")
Water bears can survive in conditions that would prove fatal for most other animals, such as exposure to extreme temperatures, pressure, and radiation. The fact that they are basically indestructible, according to NASA, makes them the perfect test subjects for an experiment about the effects of spaceflight on biological survival.

Water bears are basically indestructible, making them, according to NASA, the perfect test subjects for an experiment about the effects of spaceflight on biological survival. Thomas Boothby/University of Wyoming hide caption
Scientists want to see how traveling through the solar system affects water bears because it might help them understand what happens to astronauts when they are rocketing through space. The agency may use that information to develop "countermeasures" to make future voyages easier on human travelers.

NASA Picks Twin Missions To Visit Venus, Earth's 'Evil Twin'
"Spaceflight can be a really challenging environment for organisms, including humans, who have evolved to the conditions on Earth," said principal investigator Thomas Boothby.
"One of the things we are really keen to do is understand how tardigrades are surviving and reproducing in these environments and whether we can learn anything about the tricks that they are using and adapt them to safeguard astronauts."
The friendly microbe of a bobtail squid
Thousands of microbes live inside the human body and work to keep us healthy.
But scientists don't have a clear picture of how microgravity — which allows the kind of floating weightlessness experienced by astronauts when they travel into space — affects those microbes.
That is the subject of an ongoing NASA research program called the Understanding of Microgravity on Animal-Microbe Interactions, or UMAMI.
"Animals, including humans, rely on our microbes to maintain a healthy digestive and immune system," says UMAMI principal investigator Jamie Foster. "We do not fully understand how spaceflight alters these beneficial interactions."
That's where the bobtail squid come in.
Scientists will study whether microgravity has an impact on the relationship between newly hatched bobtail squid, or Euprymna scolopes , and their symbiotic bacterium, Vibrio fischeri .
The goal is to use what they learn about the relationship between squid and the microbes to help better prepare astronauts for lengthy space missions and preserve their health while they're out there.
The experiment could also lead to a new understanding of the ways animals and helpful microbes interact and may even have applications for improving health on Earth, NASA said.

The Two-Way
How one of the world's toughest creatures can bring itself back to life.
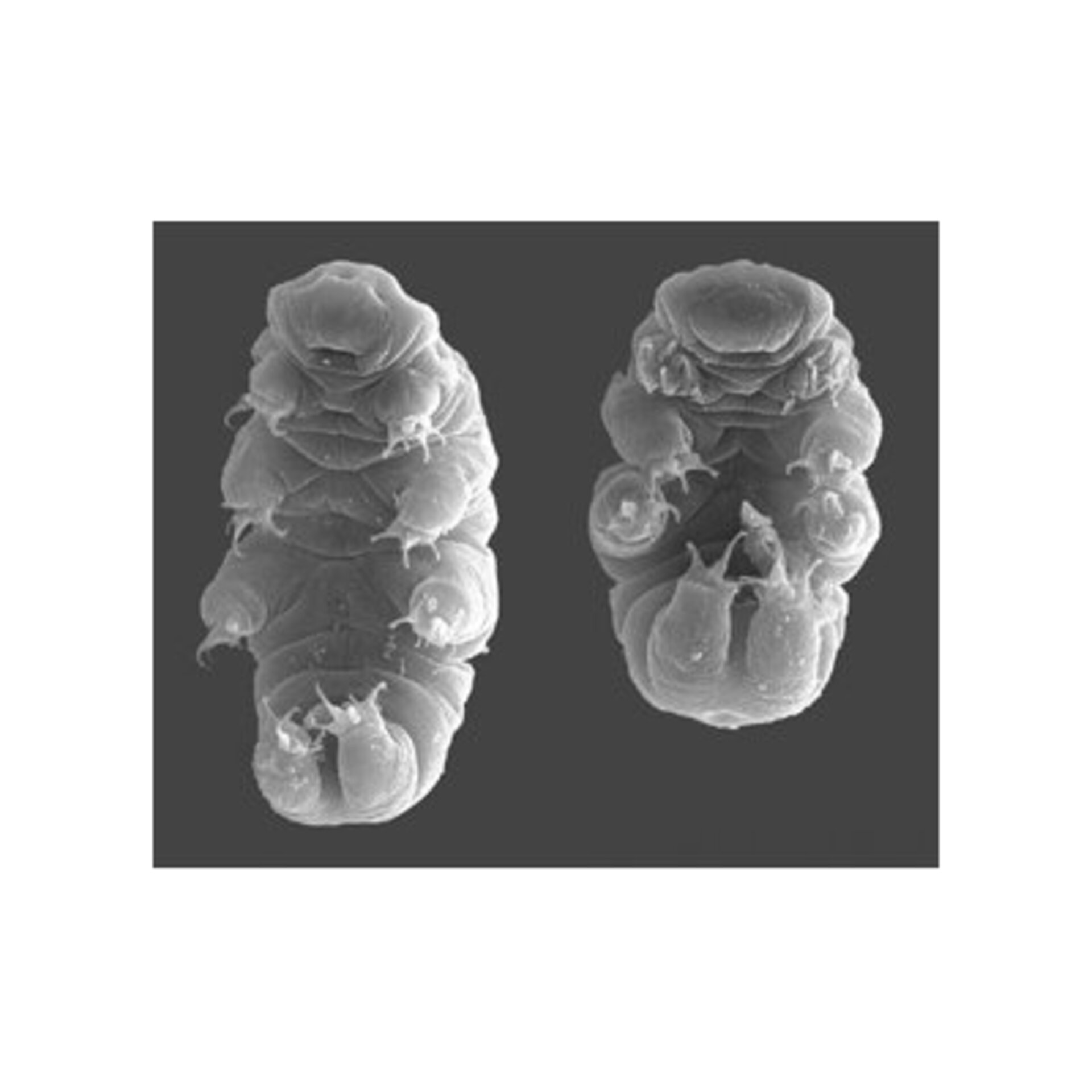
Tiny animals survive exposure to space
Scientists recently revealed that tiny creatures called water bears are the first animals to survive exposure to space. Sending water bears into space is one of several ESA experiments looking at organisms which can survive longer periods in open space.
Water bears, also known as tardigrades, are very small, segmented animals. The largest species is just over one millimetre in length. Water bears live in temporary ponds and droplets of water in soil and on moist plants. They are known to survive under conditions that would kill most organisms – they can withstand temperatures ranging from -272 deg C to +150 deg C, they can be without water for a period of 10 years, and they are extremely resistant to radiation.
Knowing them to be so hardy, the Swedish and German scientists behind the ‘Tardigrades in space’ (TARDIS) experiment wanted to find out how the water bears would fare in the harsh space environment. For 12 days in September 2007, some 3000 water bears hitched a ride into space on ESA’s orbital Foton-M3 mission.
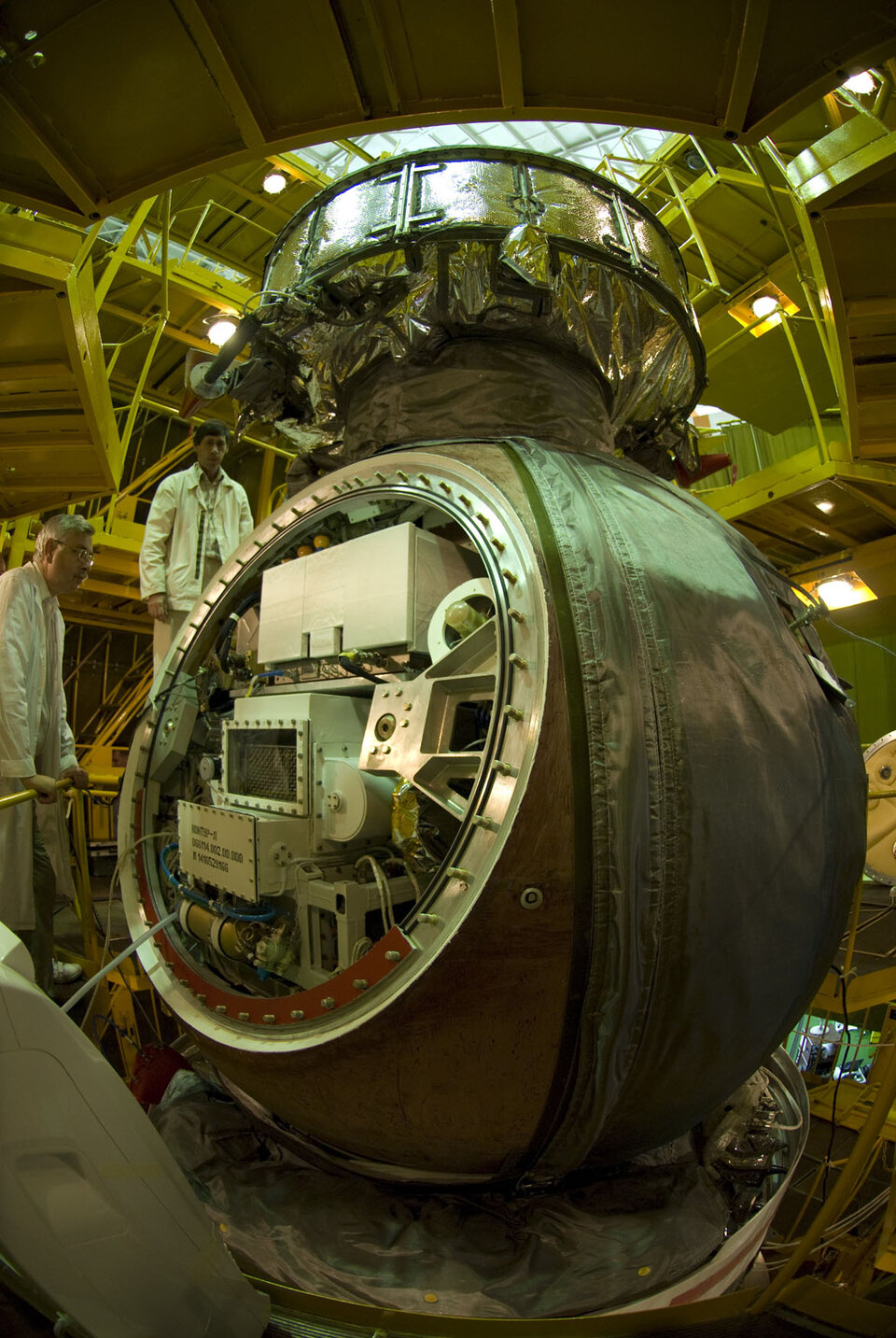
“Our principle finding is that the space vacuum, which entails extreme dehydration and cosmic radiation, were not a problem for water bears,” says TARDIS project leader Ingemar Jönsson, from the University of Kristianstad in Sweden.
That the water bears survived, shows just how robust they are. The next step will be to understand what mechanism makes this possible. Jönsson: “How do their cells stabilise the membrane and DNA when they dry out for example?” Understanding these mechanisms can open the door to many insights both in space bioscience and in other areas.
The tardigrades join a fairly select group of organisms which are able to cope with the extreme conditions in space. Over the past 10 years, other ESA experiments have shown that lettuce seeds and lichen were also able to survive exposure to space. If shaded from direct sunlight, bacterial spores are also known to survive for many years under space conditions.
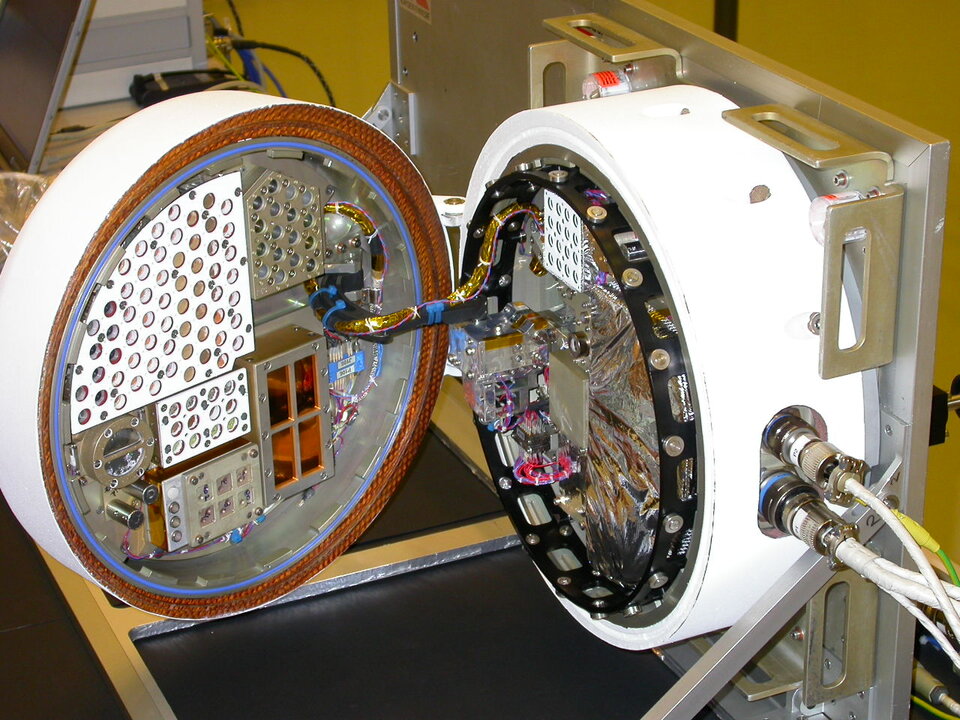
“The water bears are something new. Nobody knew about that capability” says René Demets, ESA project biologist. “The question is why are terrestrial organisms prepared to survive exposure to space conditions? Is there a rationale? Nobody knows at the moment.”
Survival in space is often linked to a much wider theory about how life originated here on Earth. “Perhaps the starting point for life was not even here on Earth,” says Demets. “Could life as we know it have started elsewhere, to be carried later for instance on a meteorite and delivered here on Earth? Favourable conditions meant that it could further propagate, develop, grow and live on. Now we have actually found some organisms that can survive under harsh space conditions.”
If these organisms were to travel on board a meteorite, they would also have to survive the entry through Earth’s atmosphere. ESA’s series of Stone experiments, also conducted on the Foton missions, show that in the upper layer of the meteorite, up to 2 cm depth, nothing could survive the atmospheric entry because of the high temperature and pressure. Only an organism that could live inside deeper cracks or pores in the rock could perhaps survive.
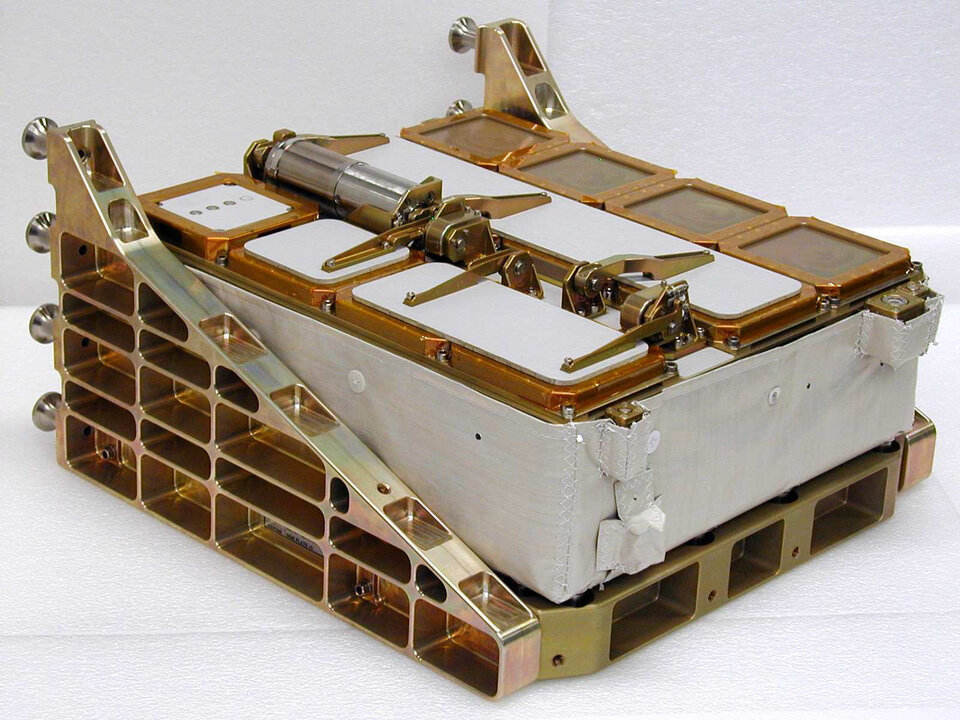
These space exposure experiments have so far been limited to one or two weeks in mission duration. ESA is now also testing longer duration exposure with an suite of experiments on the International Space Station called Expose. Several trays filled with terrestrial organisms are already installed on the outside of the European Columbus laboratory as one of the nine payloads of the European Technology Exposure Facility (EuTEF). Another Expose unit, scheduled for launch on a Russian Progress cargo carrier in November, will be attached to the Russian segment of the Space Station.
“After about one and a half years we will get the Expose trays back and see what the situation is after long duration exposure,” explains Martin Zell, Head of ESA's ISS Utilisation Department. “We could have a brilliant result with survival of the organisms as we have seen with the water bears, or we could have a quite negative result and know for sure that long-duration exposure will never work. With these experiments we are probing the alterations of organisms in space and their ultimate limits of survival.”
For more information please contact:
Martin Zell ESA Head of ISS Utilisation Department Directorate of Human Spaceflight Martin.Zell[@]esa.int
Thank you for liking
You have already liked this page, you can only like it once!
Related Links
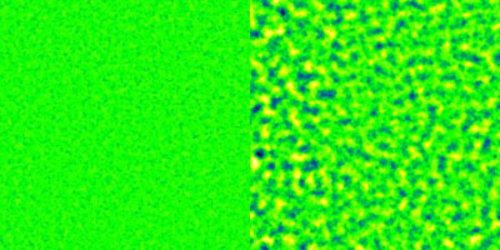
Fluid theory confirmed by Foton

Foton-M3 mission to launch European experiments

Lift-off for Foton microgravity mission
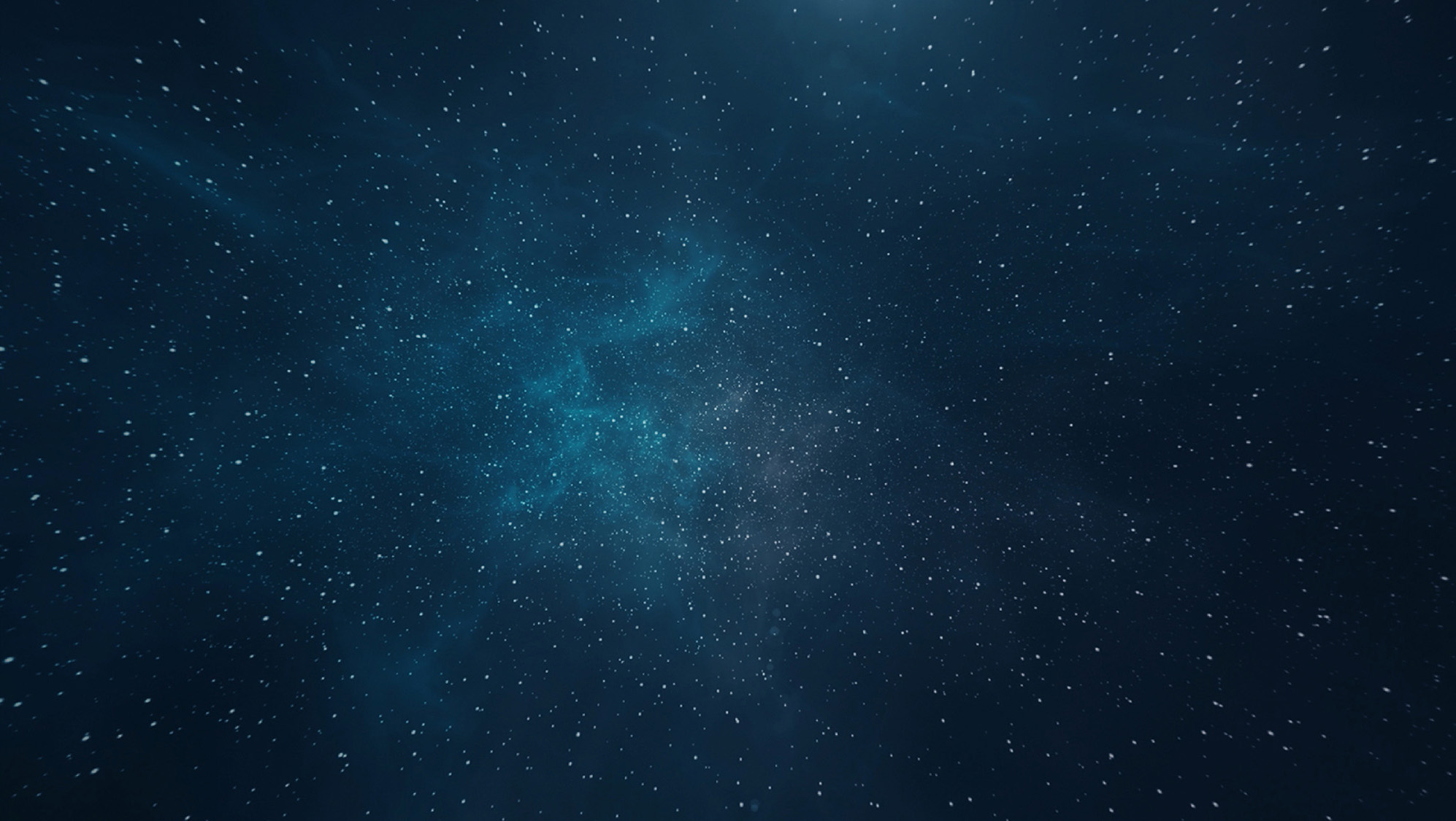
Tardigrades in space
Foton-m3 launch.

How tardigrades bear dehydration
A new mechanism explains how water bears survive in some extreme conditions.
Some species of tardigrades, or water bears as the tiny aquatic creatures are also known, can survive in different environments often hostile or even fatal to most forms of life. For the first time, researchers describe a new mechanism that explains how some tardigrades can endure extreme dehydration without dying. They explored proteins that form a gel during cellular dehydration. This gel stiffens to support and protect the cells from mechanical stress that would otherwise kill them. These proteins have also been shown to work in insect cells and even show limited functionality in human cultured cells.
Tardigrades often draw attention to themselves, despite being so tiny. Their uncanny ability to survive in situations that would kill most organisms has captured the public’s imagination. One could easily imagine that by decoding their secrets, we could apply the knowledge to ourselves to make humans more resilient to extreme temperatures, pressures, and even dehydration. This is just science fiction for now, but nevertheless, researchers, also captivated by the microscopic creatures, seek to understand the mechanisms responsible for their robustness, as this could bring other benefits too.
“Although water is essential to all life we know of, some tardigrades can live without it potentially for decades. The trick is in how their cells deal with this stress during the process of dehydration,” said Associate Professor Takekazu Kunieda from the University of Tokyo’s Department of Biological Sciences. “It’s thought that as water leaves a cell, some kind of protein must help the cell maintain physical strength to avoid collapsing in on itself. After testing several different kinds, we have found that cytoplasmic-abundant heat soluble (CAHS) proteins, unique to tardigrades, are responsible for protecting their cells against dehydration.”
Recent research into CAHS proteins reveals that they can sense when the cell encapsulating them becomes dehydrated, and that’s when they kick into action. CAHS proteins form gel-like filaments as they dry out. These form networks that support the shape of the cell as it loses its water. The process is reversible, so as the tardigrade cells become rehydrated, the filaments recede at a rate that doesn’t cause undue stress on the cell. Interestingly though, the proteins exhibited the same kind of action even when isolated from tardigrade cells.
“Trying to see how CAHS proteins behaved in insect and human cells presented some interesting challenges,” said lead author Akihiro Tanaka, a graduate student in the lab. “For one thing, in order to visualize the proteins, we needed to stain them so they show up under our microscopes. However, the typical staining method requires solutions containing water, which obviously confounds any experiment where water concentration is a factor one seeks to control for. So we turned to a methanol-based solution to get around this problem.”
Research on mechanisms related to dry preservation of cells or organisms could have many future applications. Kunieda and his team hope that through this new knowledge, researchers might find ways to improve the preservation of cell materials and biomolecules in a dry state. This could extend the shelf life of materials used for research, medicines with short expiry dates, or maybe even whole organs needed for transplants.
“Everything about tardigrades is fascinating. The extreme range of environments some species can survive leads us to explore never-before-seen mechanisms and structures. For a biologist, this field is a gold mine,” said Kunieda. “I’ll never forget New Year’s Day 2019, when I received an email from Tomomi Nakano, another author of the paper. She had been working late trying to see the condensation of CAHS proteins and observed the first CAHS filament networks in human cultured cells. I was astonished at seeing such clearly defined microscopic images of these. It was the first time I had seen such a thing. It was a very happy new year indeed!”
Knowing how to isolate and activate these special proteins, though, is just the beginning. Kunieda and his team plan to sift through more than 300 other kinds of proteins, some of which likely play a role in the incredible life-preserving ability of these tiny water bears.
- Cell Biology
- Molecular Biology
- Biotechnology
- Extreme Survival
- Developmental Biology
- Biotechnology and Bioengineering
- Dehydration
- Heat shock protein
- Natural killer cell
- Algal bloom
- Human biology
Story Source:
Materials provided by University of Tokyo . Note: Content may be edited for style and length.
Journal Reference :
- Akihiro Tanaka, Tomomi Nakano, Kento Watanabe, Kazutoshi Masuda, Gen Honda, Shuichi Kamata, Reitaro Yasui, Hiroko Kozuka-Hata, Chiho Watanabe, Takumi Chinen, Daiju Kitagawa, Satoshi Sawai, Masaaki Oyama, Miho Yanagisawa, Takekazu Kunieda. Stress-dependent cell stiffening by tardigrade tolerance proteins that reversibly form a filamentous network and gel . PLOS Biology , 2022 DOI: 10.1371/journal.pbio.3001780
Cite This Page :
Explore More
- New-To-Nature Enzyme Containing Boron Created
- New Target for Potential Leukemia Therapy
- 'Wraparound' Implants for Spinal Cord Injuries
- Climate May Influence Seismic Activity
- Atmosphere Surrounding Super-Earth?
- How Continents Stabilized
- Pressure to Be 'Perfect' Causing Burnout
- New Type of Memory State
- Mini-Robots to Clean Up Microplastics, Microbes
- Sound-Suppressing Silk Can Create Quiet Spaces
Trending Topics
Strange & offbeat.
Frontiers for Young Minds

- Download PDF
Water Bears—The Most Extreme Animals on The Planet (And in Space!)
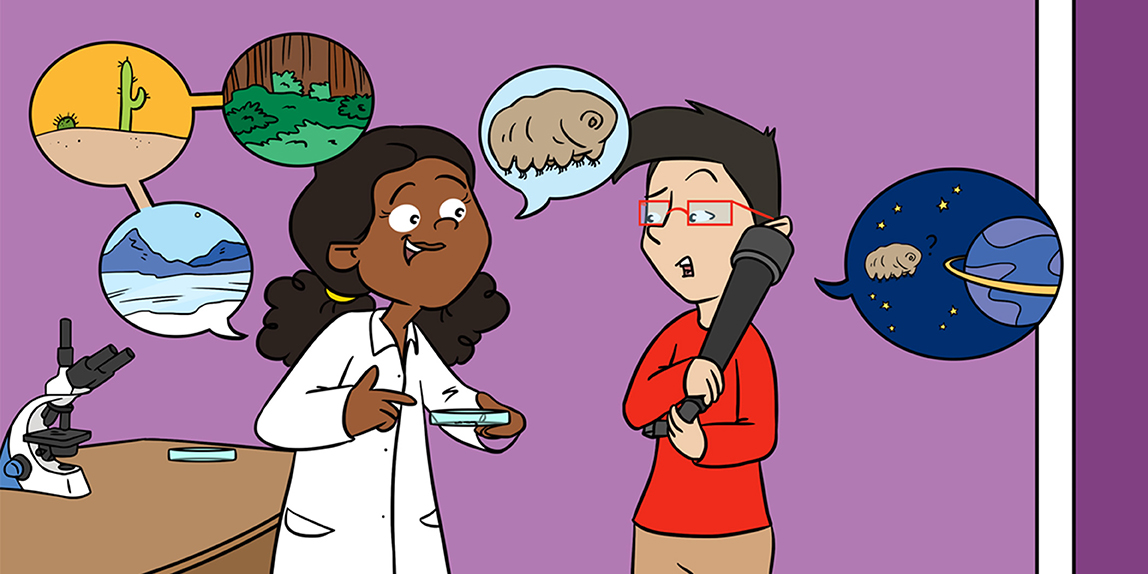
Can you imagine that there is an eight-legged bear that tolerates colder temperatures than the polar bears do in the Arctic? Can you imagine that this bear is able to grow older than the grizzly bears in North America? And can you imagine that this bear grows by molting, like spiders or snakes? These so-called water bears, scientifically named tardigrades, are the most extreme animals on our planet. They not only survive in ice, but also in boiling water. Moreover, they can stop breathing for long periods and they have even traveled to outer space, surviving without an astronaut’s suit. Since water bears can withstand the harshest conditions on earth and beyond, they may teach us how we can protect ourselves from extreme environmental conditions.
Are Water Bears True Bears?
What are water bears? Are they really bears? This question is easy to answer: no, the only thing that water bears and bears have in common is the fact that both are animals. The shape of a water bear slightly resembles that of true bears, such as the polar bear or the grizzly, but they are most closely related to the huge group called the arthropods , which includes insects, spiders, millipedes, and crabs. However, you cannot see a water bear with the naked eye, because these animals are very tiny. They usually grow to <1 mm ( Figure 1 ). Water bears were discovered more than 200 years ago [ 1 ]. The German pastor and biologist Johann Goeze initially named them “little water bears,” because of their size and their preference for wet living spaces.
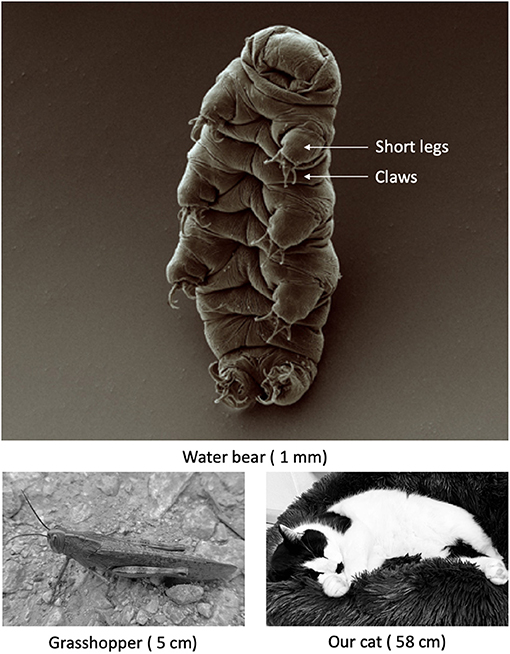
- Figure 1 - Water bears, also called tardigrades, are extremely small compared to other animals.
- This image of a water bear was taken with a scanning electron microscope. The water bear micrograph by Bob Goldstein and Vicky Madden ( https://en.wikipedia.org/wiki/Tardigrade#/media/File:Waterbear.jpg ). Photographs of the grasshopper and the cat by S. Elleuche.
Water bears love wet or at least humid environments where they can remain covered by a layer of water. They are among the most successful lifeforms known and are widely distributed all over our planet. We can observe water bears in all oceans, rivers, seas, and lakes, and in wetlands , but they are mainly found in mosses or swamps. Water bears have even conquered the highest mountains, rainforests, and Antarctica. Many different types of water bears have been found and described. They even conquered Hollywood, where you may have encountered water bears in the Marvel superhero movies “Ant-Man” and “Ant-Man and the Wasp,” when Scott Lang disappears into the quantum realm.
Water bears have a strange shape—they are of stout build with four pairs of short and stubby legs, ending with four to eight claws, and they appear to lumber along as they move ( Figure 1 ). The first three pairs of legs are used for moving, while the water bears use the last pair of legs to hang on to the surface on which they walk. Even with so many legs, water bears usually do not walk but instead passively slide, using the flow of water or wind. The way they move is also reflected by their scientific name: tardigrades . Tardigradum means “slow walker,” and this name was given to water bears by Loredano Spallanzani, a former Italian biologist, due to the slow and sedate behavior of these animals, which might look like laziness.
How Do Water Bears Grow?
Just like almost any other creature on our planet, water bears must eat food and breathe air to generate the energy needed for their cells to divide and their bodies to grow. In contrast to true bears, water bears are just too tiny to eat salmon or seals. Honey is also not on their menu. Nevertheless, water bears basically eat everything. While they mainly prefer vegetarian foods like plants and algae, they will also eat microscopic animals.
Unlike most other animals, the bodies of water bears are created following a specific plan. Every type of adult water bear even has exactly the same number of cells. Their cells are continuously dividing, but the water bear is covered by a non-growing and non-flexible sheath, or protective outer covering. As soon as the sheath becomes too tight, water bears will shed the sheath in a process called molting , similar to spiders and snakes. Although both humans and water bears need oxygen to survive, water bears do not breathe the way we do. In fact, they do not even possess respiratory organs like lungs. Water bears take up air through the surfaces of their bodies, just like insects. Water bears can even stop breathing and eating for some time, similar to the process of hibernation that allows other animals, such as polar bears, to slow down their bodily processes to survive the winter months. However, water bears are even more impressive, because not only can they sleep for a couple of months, but they can also become extremely old and thrive in the most extreme places on earth.
What Are the Most Extreme Living Spaces for Water Bears?
Water bears are the most extreme animals that we know—they basically tolerate almost every extreme condition that we can think of. They can survive in the Arctic alongside polar bears, or in Antarctica, where penguins feel at home ( Figure 2A ). Water bears even survive in the laboratory at temperatures below −200°C, which is more than twice as cold as the coldest temperature that was ever observed in nature. Under such extreme conditions, the water bears enter a stage that resembles death. During this death-like resting stage, called dormancy , water bears stop all functions that usually define life: they stop breathing, they stop moving and growing, and they even stop digesting their last meal [ 2 ]. Depending on how long they are in dormancy, it can take several hours to wake them up. Some water bears have even been seen to last for a century in dormancy.
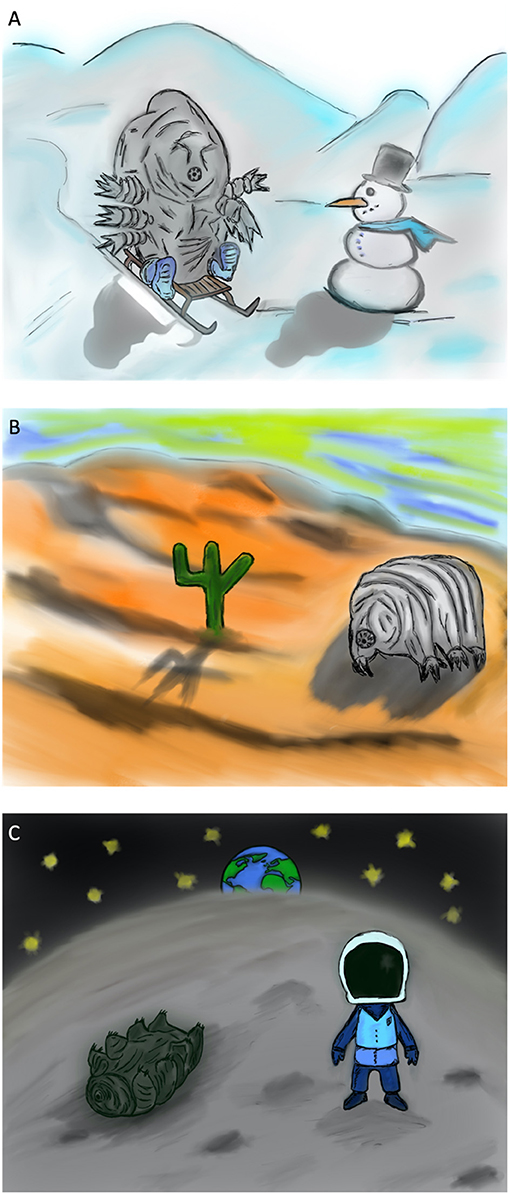
- Figure 2 - (A) Water bears can survive in extremely cold habitats, like the icy Himalaya mountains, and at temperatures as low as −150°C.
- (B) Water bears can survive in extremely hot habitats, like the hottest deserts, and at temperatures as high as 100°C. (C) Water bears can even survive in the vacuum of space!
On the other end of the temperature scale, there are microbes that can grow at temperatures around 120°C. These heat-loving microbes are called extremophiles [ 3 , 4 ]. Water bears do not love the extreme heat, but not only can water bears survive in the desert, they can even tolerate temperatures around 150°C ( Figure 2B )—temperatures that would kill most extremophiles. Even more impressive is the fact that water bears can be repeatedly heated up and frozen without dying. These abilities have allowed water bears to become unrivaled in their success over the course of evolution. More than 1,000 different types of water bears are known, with the oldest species dating back more than 500 million years.
Water bears do not only survive the coldest cold or the hottest heat without food and without air to breathe, but they can also go without water and they are resistant to radiation. Since those extreme conditions exist in space, scientists asked themselves whether water bears might even be able to travel in space ( Figure 2C ). Scientists knew that the high pressure present in the deep sea could be tolerated by water bears, but in space there is a vacuum, with lower pressure compared to earth. Nevertheless, several species of water bears were sent into space and all of them returned home in healthy condition. Moreover, more than 1,000 water bears in dormancy were crash-landed on the moon as passengers of a spacecraft in 2019. It is expected that most of these robust animals have survived the crash and could be revived by water and oxygen in the future.
Could Water Bears Be Used to Help Humans?
For a long time, scientists have been trying to understand the water bears’ resistance to radiation. Although radiation in the form of X-rays can be used by doctors to examine broken bones, radiation can also cause the destruction of the body’s instruction manual. This instruction manual is called the genome , and it is similar in every living organism on earth, including water bears. There must be a reason for the immense resistance to radiation seen in water bears, which is more than 1,000 times higher than humans’ resistance.
One part of the genome of water bears has recently been identified and reproduced in a laboratory [ 5 ]. When this factor was added to human cells grown in the same laboratory, the human cells tolerated more intense radiation than did human cells without the water bear factor. These early experiments may lead to future applications of water bear factors that could not only be used to protect the human cells against radiation, but possibly also to stabilize drugs or to increase the resistance of crop plants to environmental conditions like drought.
What We Have Learned From Water Bears
So, now you can see that those little water bears are quite different from the bears we know well. We have learned from these animals that they not only tolerate the most extreme conditions on our planet, they are even capable to survive in Space. Because of these unique properties, water bears are fascinating and among the most interesting model organisms for us to further study.
Arthropods : ↑ This group of animals is characterized by the outer skeleton and includes insects, spiders, millipedes, and crabs.
Wetland : ↑ A living space for multiple organisms that is temporary or permanently flooded by water and inhabited by aquatic plants.
Tardigrade : ↑ A scientific nomenclature for a group of animals that are also known as water bears or moss piglets.
Molting : ↑ Some animals, such as water bears, insects, spiders and snakes do not grow continuously. They have to replace their outer sheath when it became too tight.
Dormancy : ↑ Death-like resting stage during which each kind of activity such as growth or ingestion is temporarily stopped.
Extremophiles : ↑ Microorganisms that love to live in the most extreme environments on the planet. Water bears are no true extremophiles because, although they can tolerate extreme conditions, they do not prefer such environments.
Genome : ↑ A kind of construction plan that is included in every living cell in all organisms (Bacteria, Fungi, Plants, Animals etc.), which determines the look and composition of most cellular compon.
Conflict of Interest
The author declares that the research was conducted in the absence of any commercial or financial relationships that could be construed as a potential conflict of interest.
Acknowledgments
The author thanks Sylvia Wiese and Jan Friesen for critically reading the manuscript.
[1] ↑ Jönsson, K. I. 2019. Radiation tolerance in tardigrades: current knowledge and potential applications in medicine. Cancers 11:1333. doi: 10.3390/cancers11091333
[2] ↑ Fontaneto, D. 2019. Long-distance passive dispersal in microscopic aquatic animals. Mov. Ecol . 7:10. doi: 10.1186/s40462-019-0155-7
[3] ↑ Elleuche, S., Schröder, C., Stahlberg, N., and Antranikian, G. 2017. “Boiling water is not too hot for us!”—preferred living spaces of heat-loving microbes. Front. Young Minds . 5:1. doi: 10.3389/frym.2017.00001
[4] ↑ Schröder, C., Burkhardt, C., Antranikian, G. 2020. What we learn from extremophiles. ChemTexts 6:8. doi: 10.1007/s40828-020-0103-6
[5] ↑ Hashimoto, T., Horikawa, D. D., Saito, Y., Kuwahara, H., Kozuka-Hata, H., Shin, I. T., et al. 2016. Extremotolerant tardigrade genome and improved radiotolerance of human cultured cells by tardigrade-unique protein. Nat. Commun . 7:12808. doi: 10.1038/ncomms12808
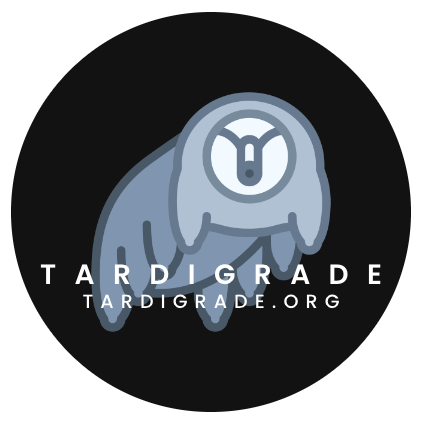
Survivalists at the Micro Level: The Amazing World of Tardigrades
Welcome to the incredible microcosm of survivalists – tardigrades. Known as water bears, these microscopic marvels defy the limits of endurance in the most challenging environments. From the deep sea to towering mountains, tardigrades showcase unparalleled adaptability. In this exploration, we unravel the secrets behind their resilience, cryptobiosis mastery, and survival in space, unveiling the amazing world of tardigrades as true champions at the micro level.
Microscopic Wonders
Tardigrades, affectionately termed water bears, are exceptional microscopic organisms, epitomizing extraordinary resilience. Despite their diminutive size, these creatures exhibit an unparalleled ability to withstand extreme conditions, navigating environments where others falter. From the depths of the ocean to the highest mountain peaks, tardigrades showcase a tenacity that defies their minuscule stature. Colloquially celebrated as water bears, these microscopic wonders capture the essence of survival at the micro level, embodying an awe-inspiring ability to endure and thrive in the face of challenges that surpass the limits of larger organisms.
Diverse Habitats
Tardigrades, commonly known as water bears, emerge as masters of adaptation, thriving across a spectrum of diverse habitats that span the Earth’s extremes. From the lightless depths of the deep sea to the lofty heights of mountainous terrains, these microscopic marvels showcase an unparalleled adaptability to the harshest conditions nature offers.
In the ocean’s abyssal plains, tardigrades navigate the crushing pressures, demonstrating a resilience that sets them apart. Scaling mountainous landscapes, they endure oxygen-thin air and temperature extremes. Tardigrades are equally at home in mosses, lichens, and leaf litter, showcasing their ability to persist in microenvironments that pose challenges to most life forms.
The astounding adaptability of tardigrades across such diverse ecosystems reflects their prowess in conquering extremes. This adaptability not only fuels their survival but also positions them as extraordinary indicators of life’s potential in environments deemed inhospitable to many. As we explore their presence in various habitats, we gain a profound appreciation for the resilience and versatility that define these microcosmic survivalists.
Resilience Unveiled
Tardigrades, colloquially known as water bears, reveal a profound mystery in the realm of resilience, defying conventional limits and thriving where few organisms dare to venture. This microscopic wonder unfolds a tale of unparalleled endurance, showcasing the secrets behind their ability to withstand extreme conditions. Whether subjected to harsh temperatures, intense pressures, or the vacuum of space, tardigrades stand resilient, unraveling nature’s secrets of survival at a scale that challenges our understanding. The water bear’s resilience unveils a microcosmic marvel, inviting us to explore the extraordinary mechanisms that enable them to triumph in the face of adversity.
Cryptobiosis Mastery
Tardigrades, the microscopic marvels often referred to as water bears, wield an extraordinary survival strategy – cryptobiosis. In this remarkable state, they adeptly shut down their metabolism, a feat that enables them to endure the harshest conditions, including desiccation. This microcosmic mastery allows water bears to suspend their biological activities, essentially entering a state of suspended animation. Cryptobiosis emerges as the key to their resilience, unveiling a fascinating adaptation that empowers tardigrades to navigate extreme environments and persevere in the face of challenges that would spell doom for many other life forms.
Space Survivors:
Embark on a cosmic journey with tardigrades, the resolute water bears, as they venture into the inhospitable realms of outer space and emerge as extraordinary space survivors. In pioneering experiments, these microscopic marvels have defied the odds, enduring the vacuum and radiation of space aboard spacecraft. Strapped to the exterior of satellites and shuttles, tardigrades have withstood the harsh conditions, showcasing an incredible resilience that challenges our perceptions of life’s fragility beyond Earth. As space travelers, they offer insights into the potential for life to endure in extraterrestrial environments, inspiring awe and curiosity about the microorganisms that defy the cosmic challenges of the void.
Biological Adaptability:
Tardigrades, endearingly known as water bears, unveil a stunning display of biological adaptability, demonstrating an unparalleled ability to persist in diverse and hostile environments. These diminutive creatures navigate extremes with remarkable ease, showcasing a versatility that defies conventional limits. From the depths of the ocean to the peaks of mountains, tardigrades embody an exceptional adaptability that allows them to thrive where others struggle. Their ability to confront and conquer varied challenges reflects a microcosmic triumph of biological resilience, offering a testament to nature’s ingenuity in creating life forms capable of flourishing in the most contrasting landscapes.
Anhydrobiosis Feat:
Delve into the extraordinary phenomenon of anhydrobiosis, a captivating survival strategy mastered by tardigrades, the resilient water bears. In this remarkable process, these microscopic marvels can shed almost all body water content, entering a desiccated state, only to spring back to life upon rehydration. Anhydrobiosis allows tardigrades to withstand extreme dehydration, navigating environments where water scarcity would typically be lethal. Witnessing this feat of desiccation tolerance unveils a microcosmic spectacle of adaptation, where tardigrades seemingly defy the constraints of conventional life, showcasing a remarkable ability to suspend life processes and revive when conditions once again become conducive.
Scientific Marvels:
Tardigrades, often affectionately called water bears, emerge as true scientific marvels, unravelling mysteries that captivate researchers and broaden our understanding of extremophiles and life’s potential in extreme environments. These microscopic organisms, with their unparalleled resilience, become invaluable subjects of study, providing critical insights into the boundaries of life on Earth and beyond.
As extremophiles, tardigrades redefine our understanding of where life can thrive. Their ability to endure extreme temperatures, pressures, and even the vacuum of space positions them as extraordinary contributors to astrobiology and the exploration of extraterrestrial life.
Studying tardigrades not only expands our knowledge of these microscopic marvels but also fuels scientific curiosity about the adaptability of life in the cosmos. In unlocking the secrets held by water bears, scientists glimpse into the intricacies of survival, resilience, and the potential for life to persist in the most challenging corners of the universe. Tardigrades stand at the intersection of scientific fascination, offering a gateway to exploring the frontiers of life’s tenacity and adaptability in environments previously thought uninhabitable.
Microbial Survivors:
Acknowledging the microscopic wonders known as tardigrades reveals an extraordinary narrative of tiny survivors contributing significantly to our understanding of microbial survival strategies. These water bears, though minuscule, play a pivotal role in unraveling the intricacies of how microorganisms persist in diverse and often challenging environments.
Tardigrades, with their resilience to extreme conditions, act as ambassadors for microbial life, showcasing adaptability that transcends the limitations of size. By navigating the abyssal depths of the sea, scaling towering mountains, and even braving the rigors of outer space, these microbial survivors become invaluable subjects in the exploration of microbial survival tactics.
Understanding the strategies employed by tardigrades sheds light on the broader realm of microbial life. Their ability to withstand desiccation, endure radiation, and thrive in various ecosystems underscores the versatility of microbial survival. As we acknowledge the significance of tardigrades, we gain insights into the fundamental mechanisms that govern microbial resilience, fostering a deeper appreciation for the myriad ways these tiny survivors contribute to the intricate tapestry of life on our planet and, potentially, beyond.
Conclusion
In delving into the amazing world of tardigrades, the microscopic survivalists, we unveil a testament to nature’s ingenuity and the resilience of life at the micro level. From the depths of the ocean to the vastness of outer space, these water bears defy conventional limits, showcasing unparalleled adaptability and survival strategies.
Tardigrades, with their mastery of cryptobiosis, endurance in space, and ability to navigate extreme environments, stand as extraordinary ambassadors of microbial resilience. Their significance extends beyond their diminutive size, offering valuable insights into extremophiles and challenging our understanding of where life can thrive.
As we conclude this exploration, we acknowledge the exceptional role tardigrades play in advancing scientific knowledge. Their microcosmic triumphs inspire awe and curiosity, inviting us to ponder the broader implications for life’s potential in the universe. Tardigrades, the unsung heroes of microscopic survival, continue to illuminate the wonders of life at the smallest scales, leaving an indelible mark on the scientific landscape.
- A: Tardigrades, colloquially known as water bears, are microscopic, water-dwelling animals known for their resilience.
- A: Tardigrades inhabit diverse environments, including mosses, lichens, leaf litter, deep-sea environments, and even outer space.
- A: Tardigrades earned the nickname “water bears” due to their lumbering movement reminiscent of a bear’s walk.
- A: Cryptobiosis is a state where tardigrades suspend their metabolism, enabling them to survive extreme conditions like desiccation.
- A: Yes, tardigrades have demonstrated the ability to survive the vacuum and radiation of outer space.
- A: Tardigrades have been discovered in a wide range of environments, from the deep sea to high mountain peaks.
Leave a Comment Cancel Reply
Your email address will not be published. Required fields are marked *
Save my name, email, and website in this browser for the next time I comment.
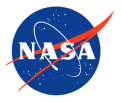
Suggested Searches
- Climate Change
- Expedition 64
- Mars perseverance
- SpaceX Crew-2
- International Space Station
- View All Topics A-Z
Humans in Space
Earth & climate, the solar system, the universe, aeronautics, learning resources, news & events.
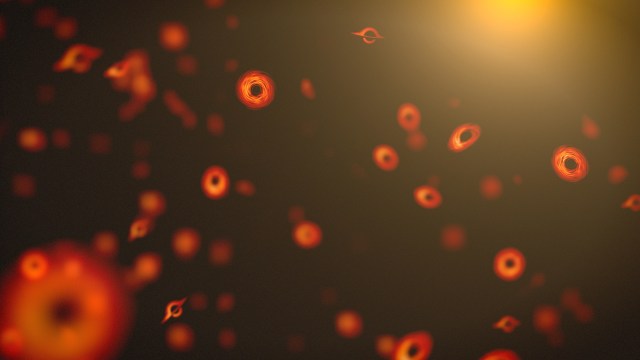
How NASA’s Roman Mission Will Hunt for Primordial Black Holes
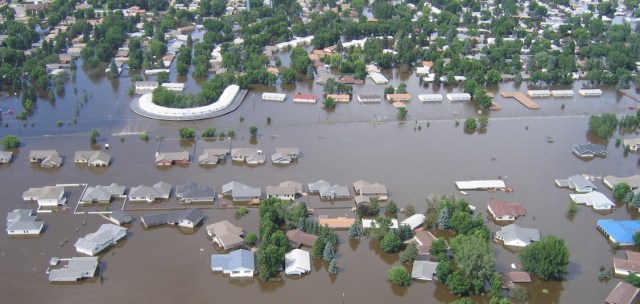
International SWOT Mission Can Improve Flood Prediction
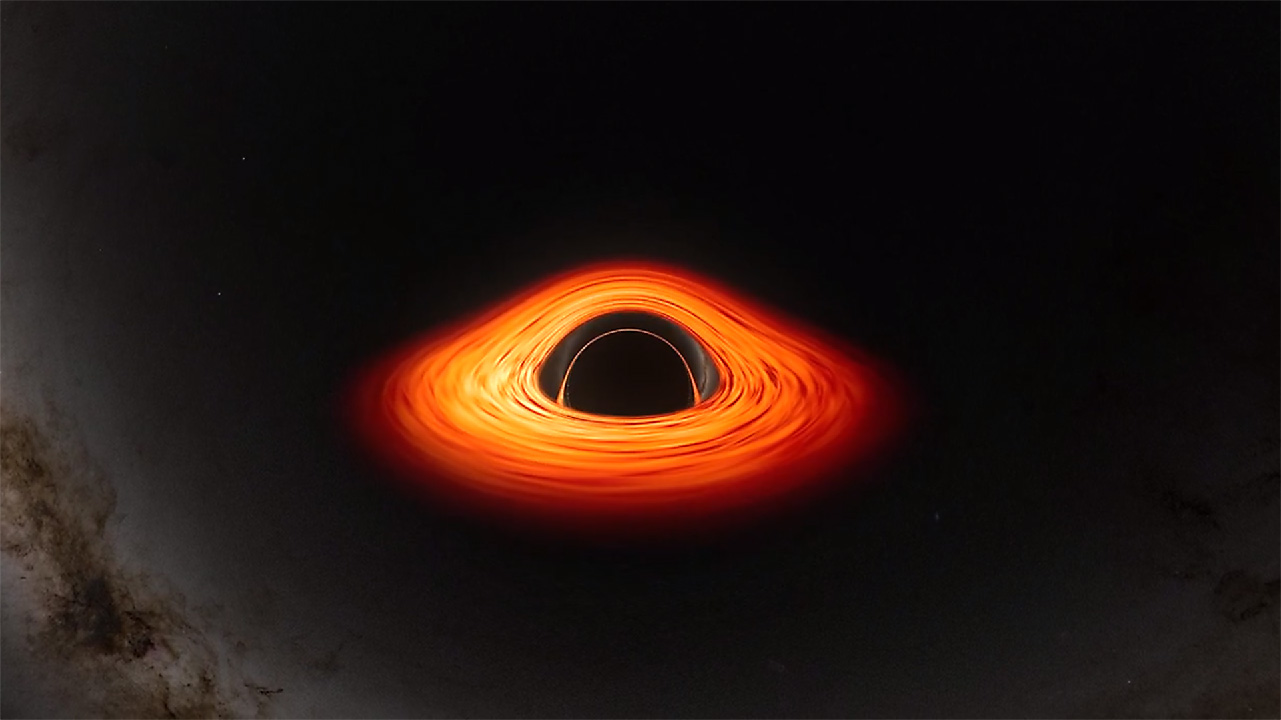
New NASA Black Hole Visualization Takes Viewers Beyond the Brink
- Search All NASA Missions
- A to Z List of Missions
- Upcoming Launches and Landings
- Spaceships and Rockets
- Communicating with Missions
- James Webb Space Telescope
- Hubble Space Telescope
- Why Go to Space
- Astronauts Home
- Commercial Space
- Destinations
- Living in Space
- Explore Earth Science
- Earth, Our Planet
- Earth Science in Action
- Earth Multimedia
- Earth Science Researchers
- Pluto & Dwarf Planets
- Asteroids, Comets & Meteors
- The Kuiper Belt
- The Oort Cloud
- Skywatching
- The Search for Life in the Universe
- Black Holes
- The Big Bang
- Dark Energy & Dark Matter
- Earth Science
- Planetary Science
- Astrophysics & Space Science
- The Sun & Heliophysics
- Biological & Physical Sciences
- Lunar Science
- Citizen Science
- Astromaterials
- Aeronautics Research
- Human Space Travel Research
- Science in the Air
- NASA Aircraft
- Flight Innovation
- Supersonic Flight
- Air Traffic Solutions
- Green Aviation Tech
- Drones & You
- Technology Transfer & Spinoffs
- Space Travel Technology
- Technology Living in Space
- Manufacturing and Materials
- Science Instruments
- For Kids and Students
- For Educators
- For Colleges and Universities
- For Professionals
- Science for Everyone
- Requests for Exhibits, Artifacts, or Speakers
- STEM Engagement at NASA
- NASA's Impacts
- Centers and Facilities
- Directorates
- Organizations
- People of NASA
- Internships
- Our History
- Doing Business with NASA
- Get Involved
- Aeronáutica
- Ciencias Terrestres
- Sistema Solar
- All NASA News
- Video Series on NASA+
- Newsletters
- Social Media
- Media Resources
- Upcoming Launches & Landings
- Virtual Events
- Sounds and Ringtones
- Interactives
- STEM Multimedia
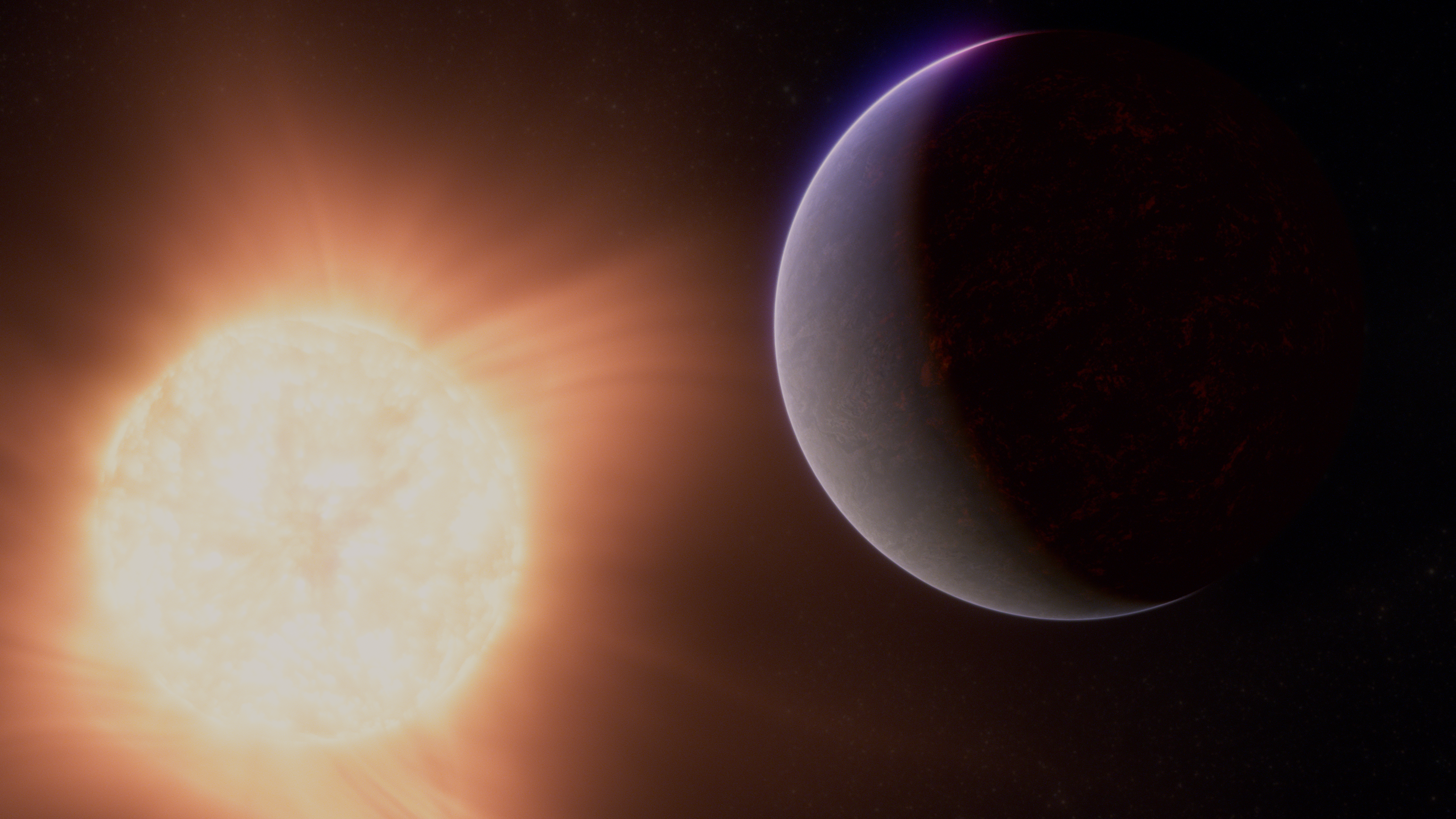
NASA’s Webb Hints at Possible Atmosphere Surrounding Rocky Exoplanet
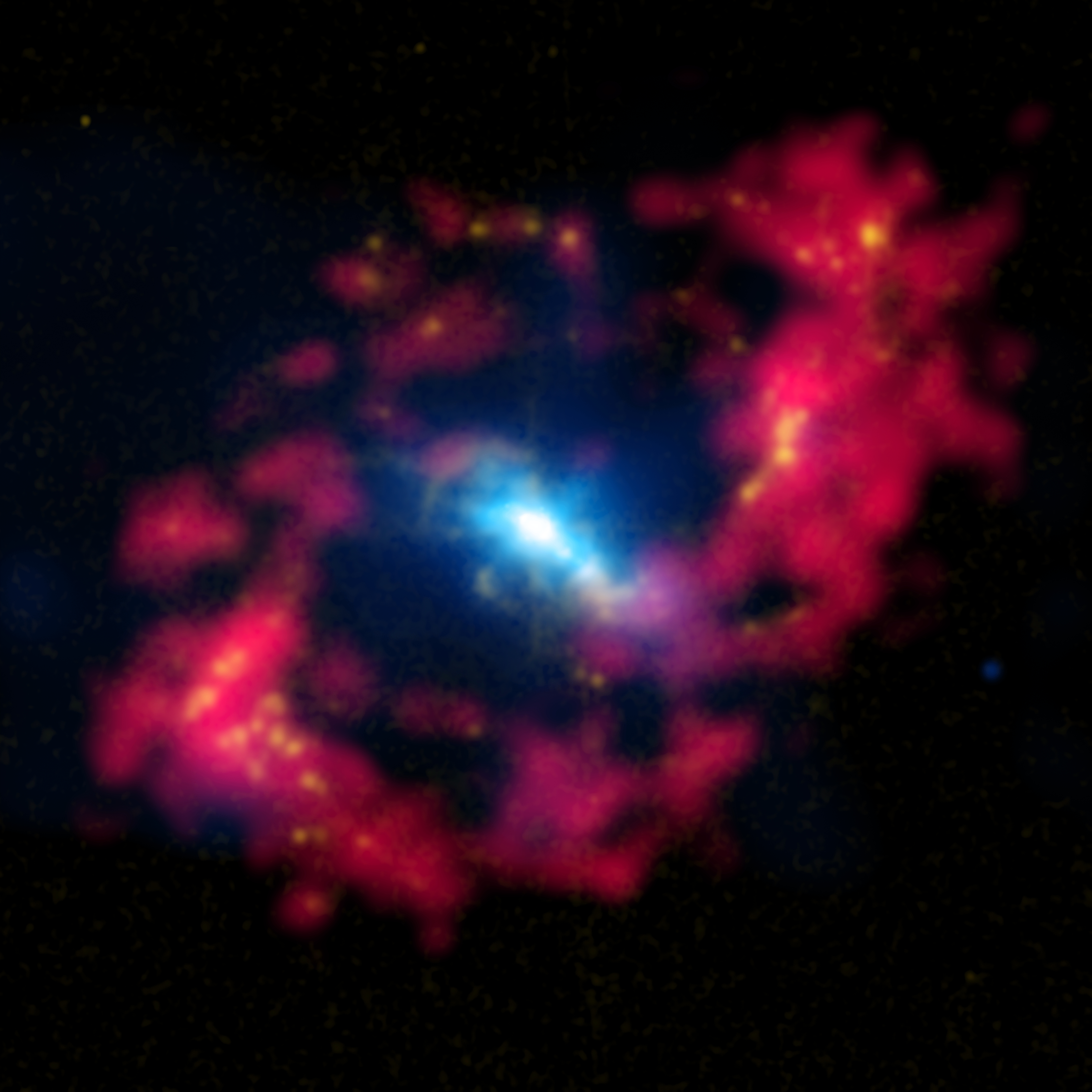
NASA, JAXA XRISM Spots Iron Fingerprints in Nearby Active Galaxy
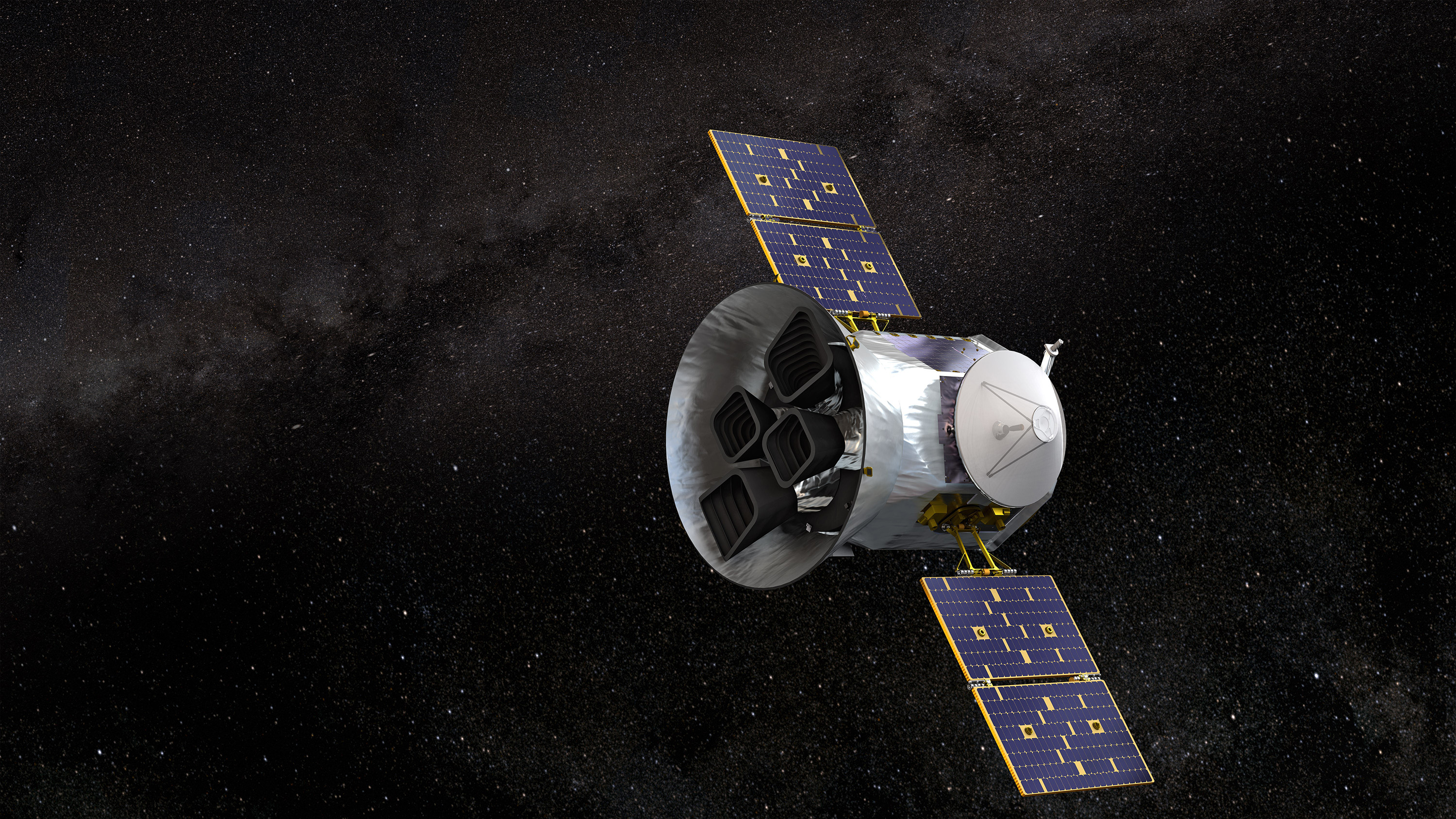
NASA’s TESS Returns to Science Operations
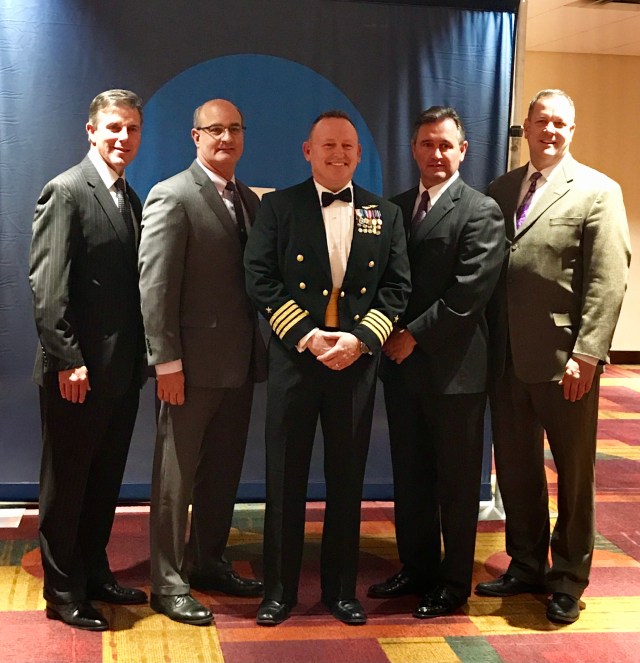
NASA Mission Strengthens 40-Year Friendship
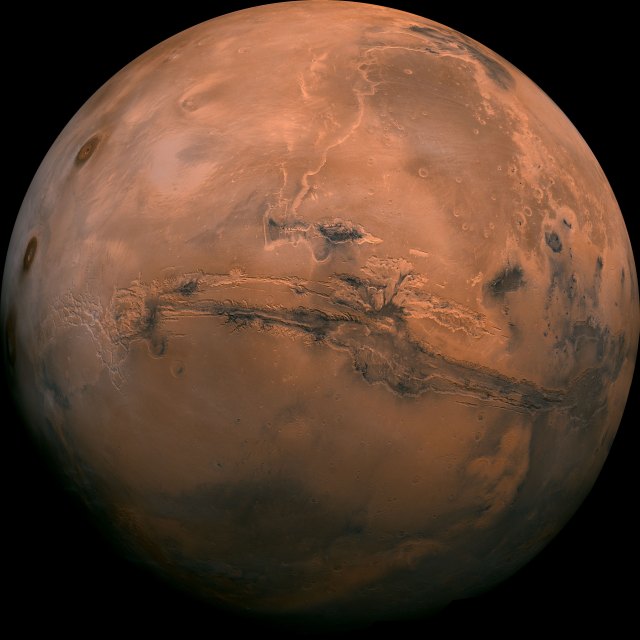
NASA Selects Commercial Service Studies to Enable Mars Robotic Science
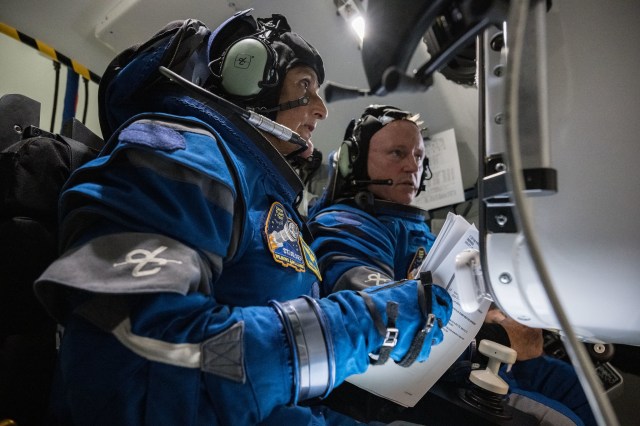
NASA’s Commercial Partners Deliver Cargo, Crew for Station Science
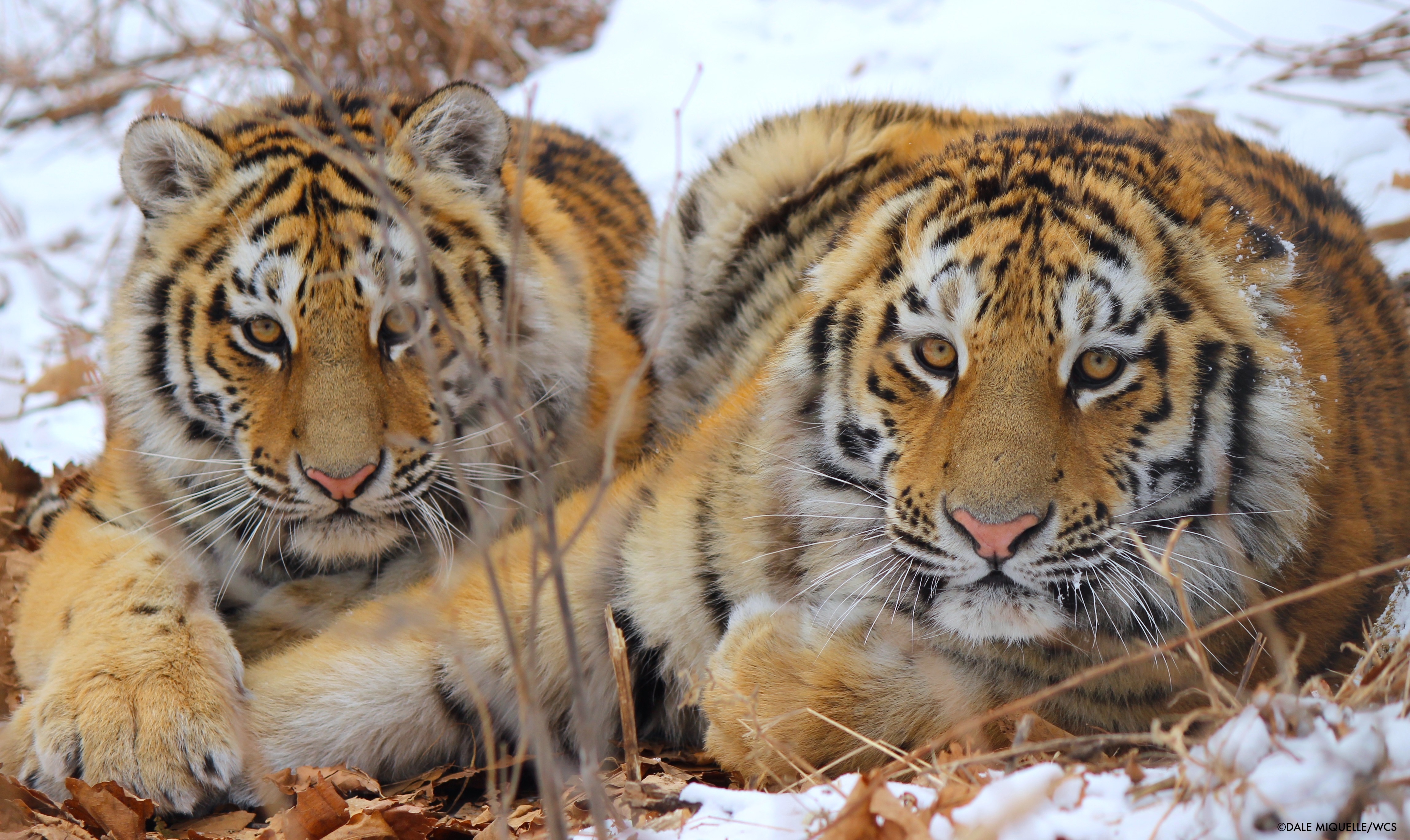
NASA Is Helping Protect Tigers, Jaguars, and Elephants. Here’s How.
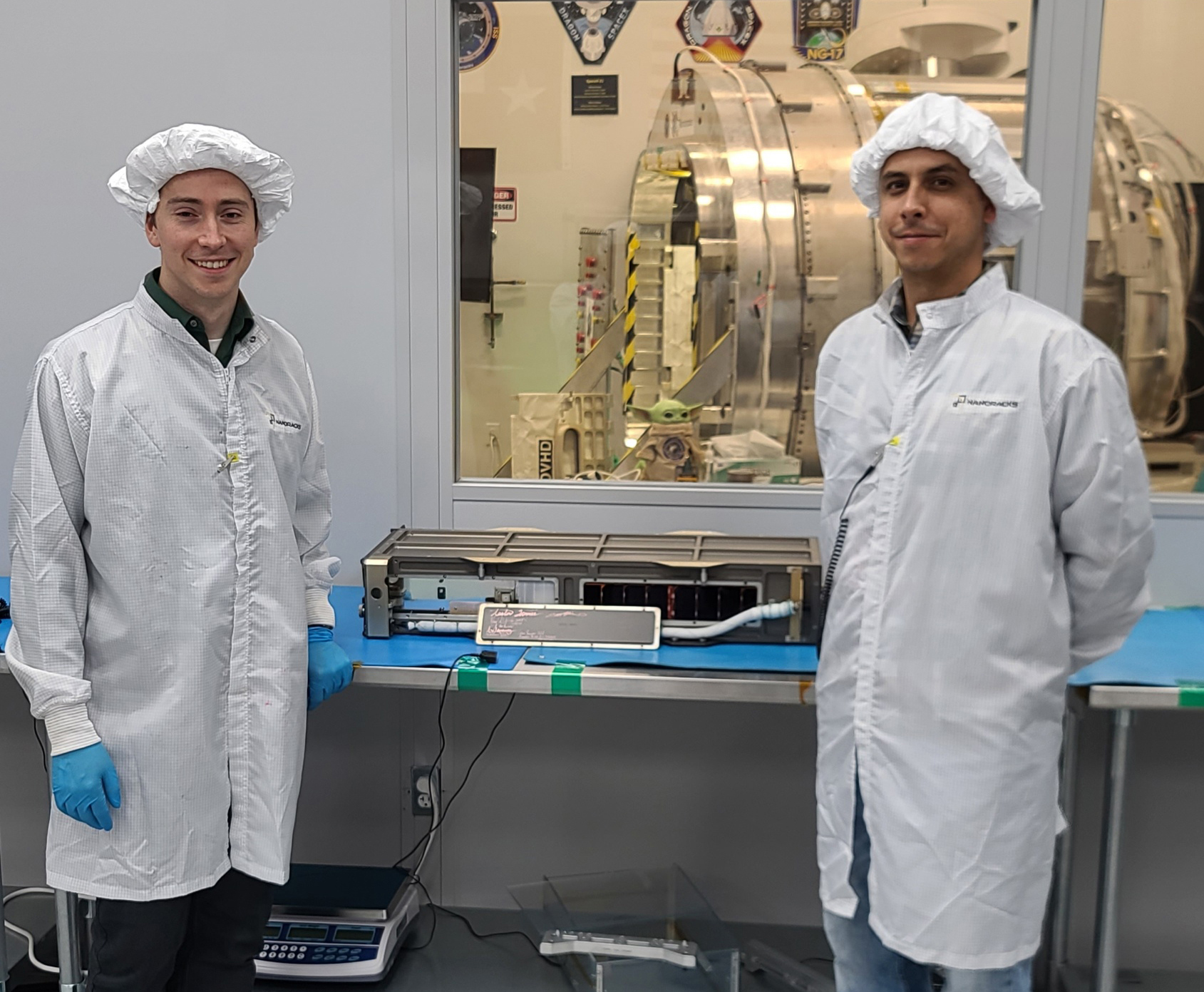
Two Small NASA Satellites Will Measure Soil Moisture, Volcanic Gases
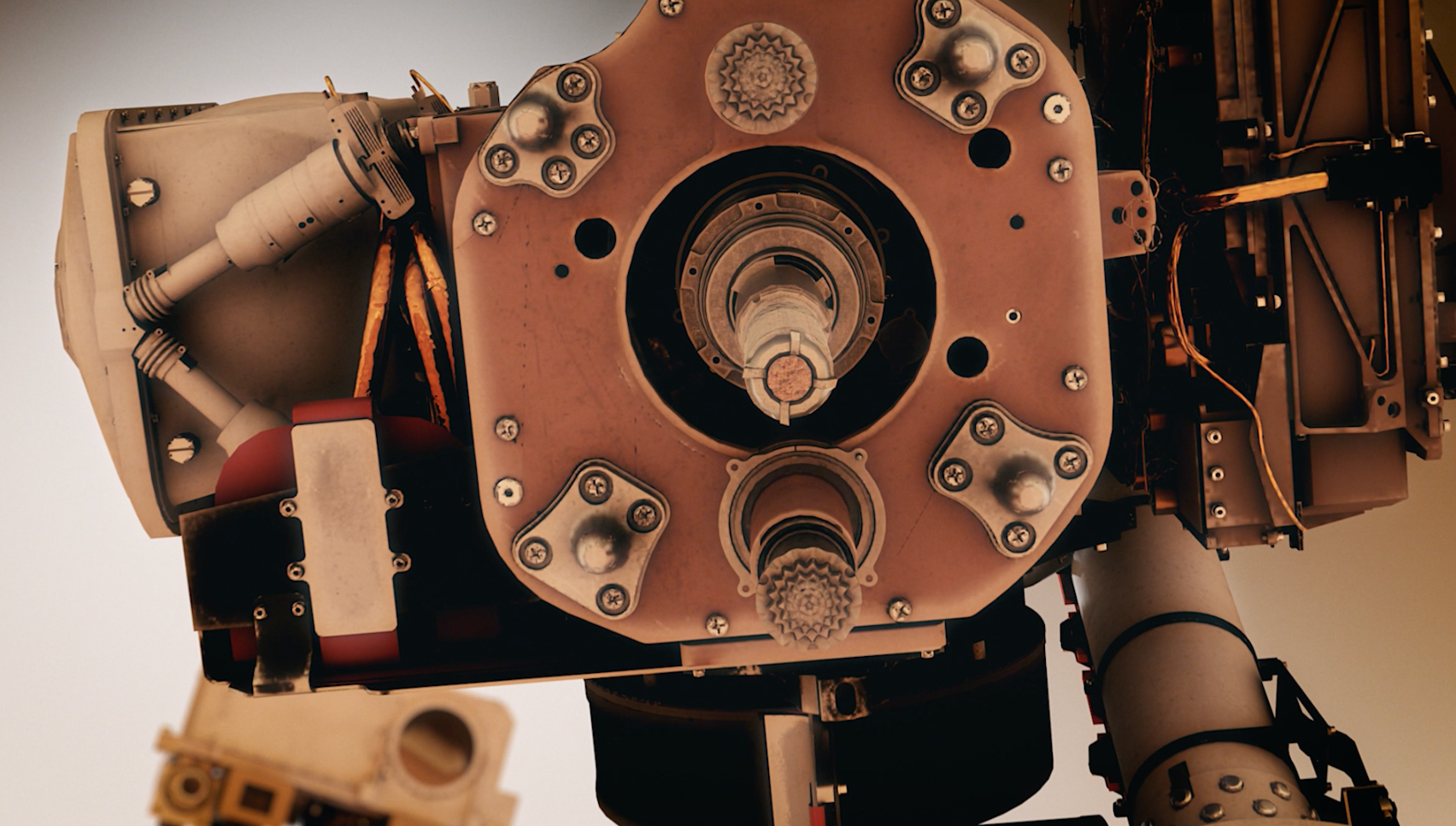
C.26 Rapid Mission Design Studies for Mars Sample Return Correction and Other Documents Posted
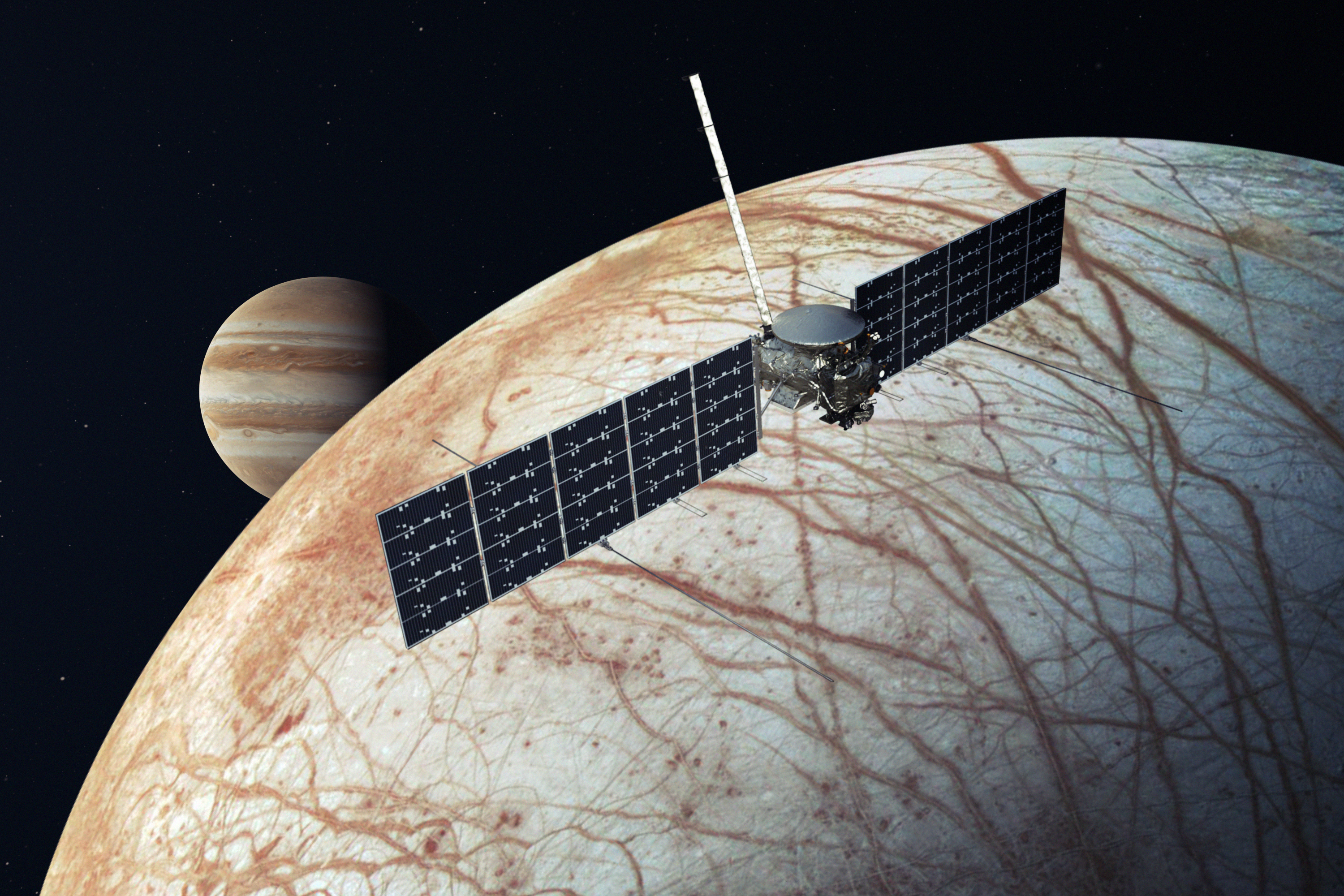
NASA Selects Students for Europa Clipper Intern Program
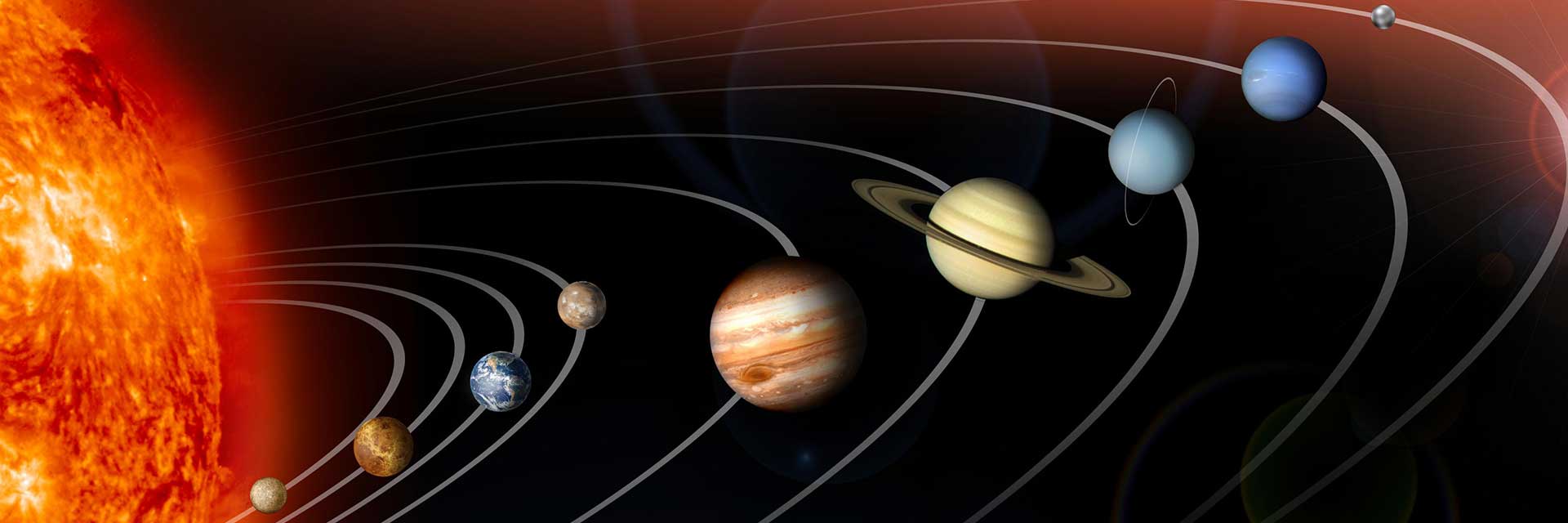
Orbits and Kepler’s Laws
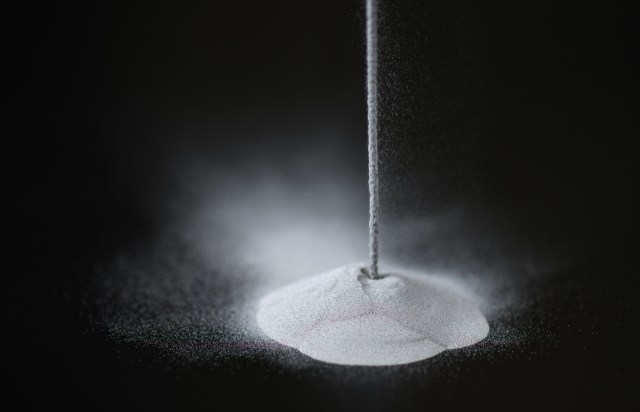
NASA Licenses 3D-Printable Superalloy to Benefit US Economy
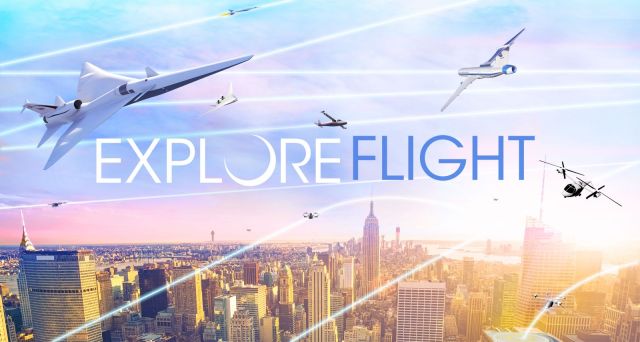
ARMD Solicitations

NASA’s Commitment to Safety Starts with its Culture
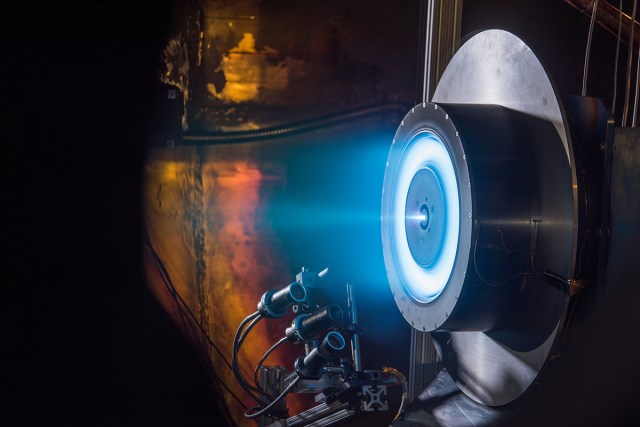
Tech Today: NASA’s Ion Thruster Knowhow Keeps Satellites Flying
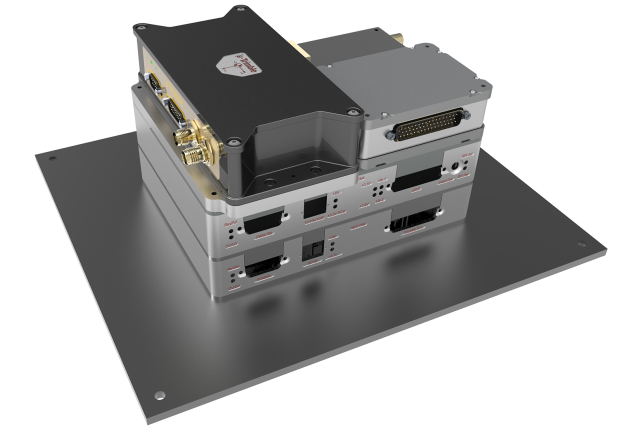
Big Science Drives Wallops’ Upgrades for NASA Suborbital Missions
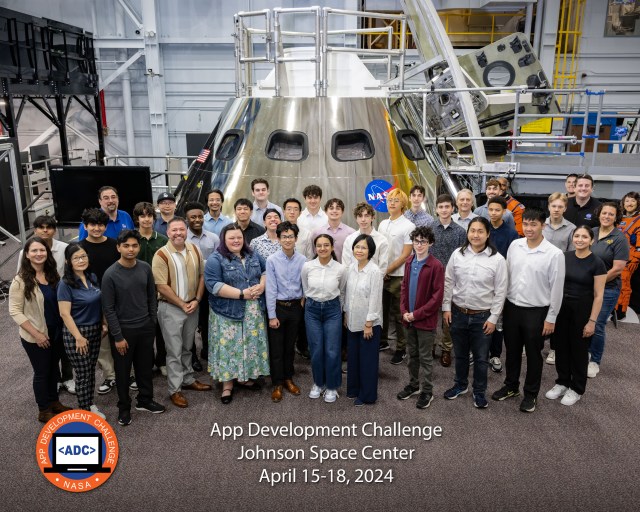
NASA Challenge Gives Artemis Generation Coders a Chance to Shine
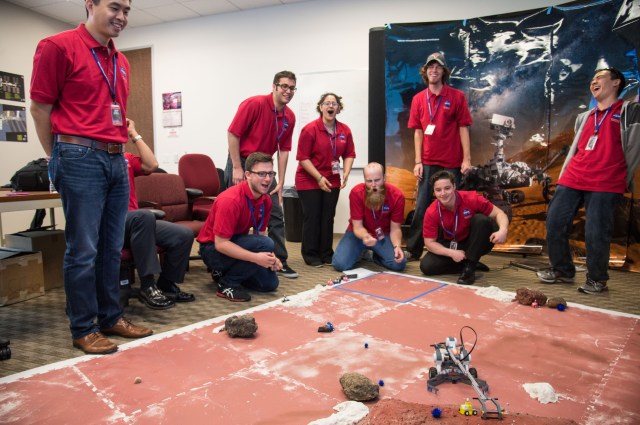
NASA Community College Aerospace Scholars
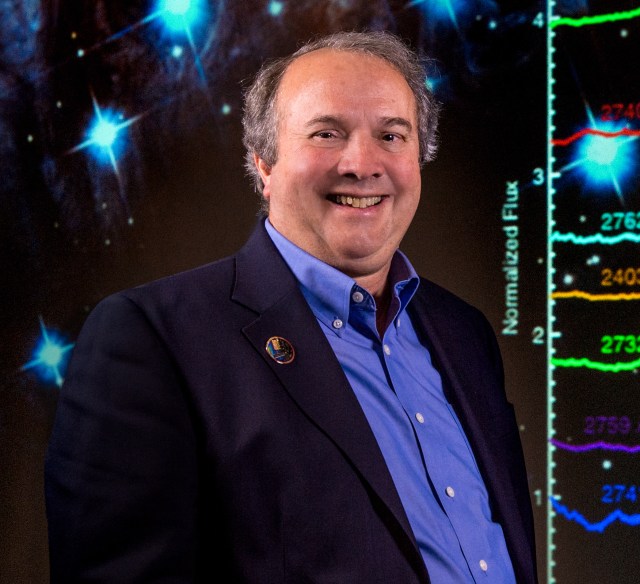
Ken Carpenter: Ensuring Top-Tier Science from Moon to Stars
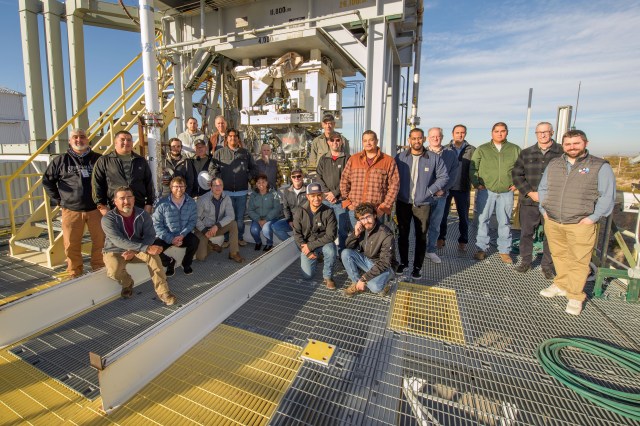
White Sands Propulsion Team Tests 3D-Printed Orion Engine Component
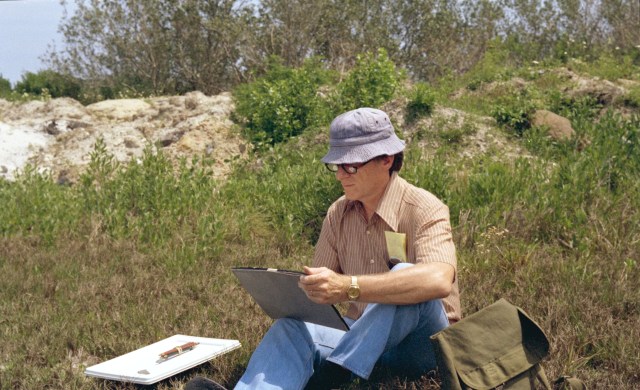
A Different Perspective – Remembering James Dean, Founder of the NASA Art Program
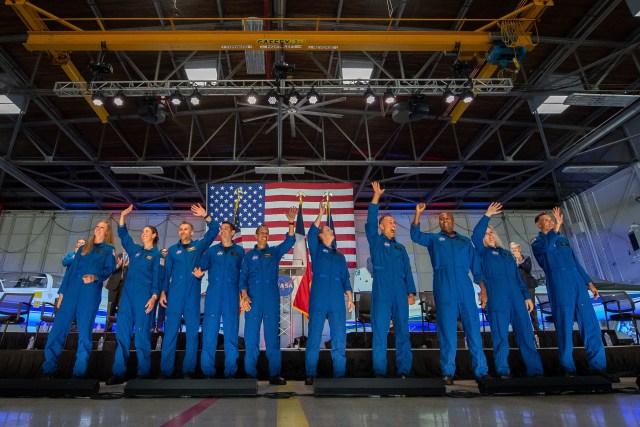
Diez maneras en que los estudiantes pueden prepararse para ser astronautas
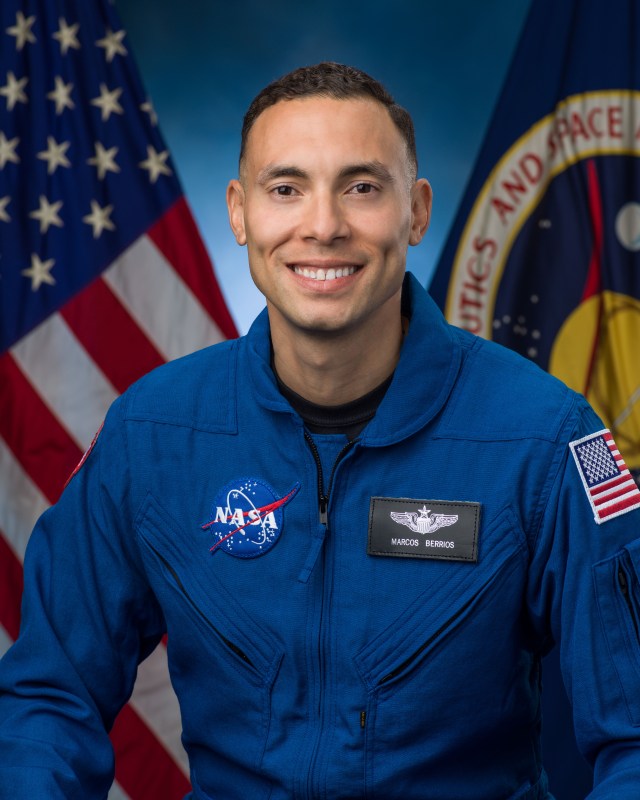
Astronauta de la NASA Marcos Berríos
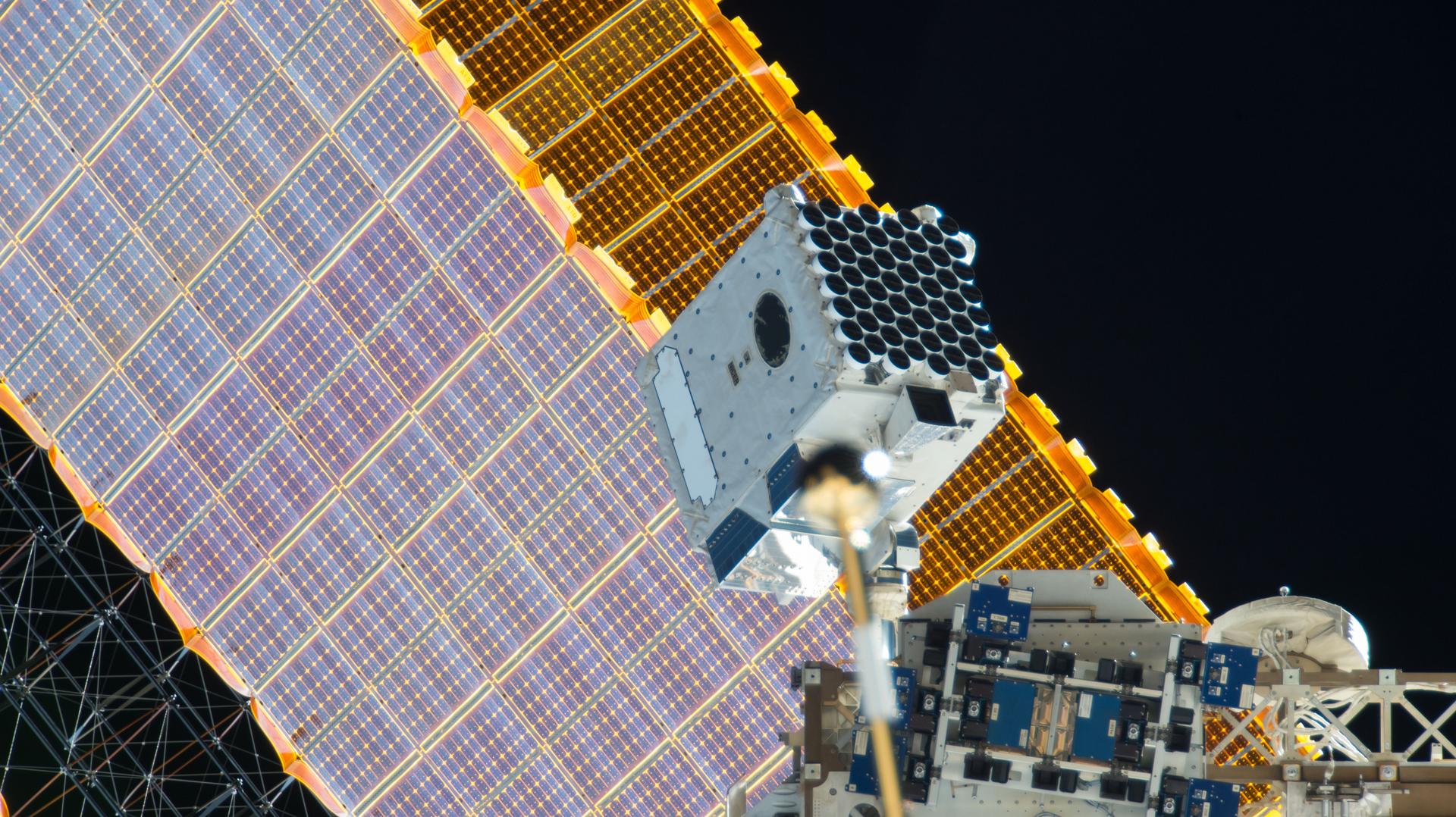
Resultados científicos revolucionarios en la estación espacial de 2023
Water bears in space.
Thomas Boothby, assistant professor for the Department of Molecular Biology at the University of Wyoming, teaches us about tardigrades, more commonly known as water bears, that are headed up to the International Space Station for a scientific study to learn how these extremophiles adapt to microgravity. HWHAP Episode 197
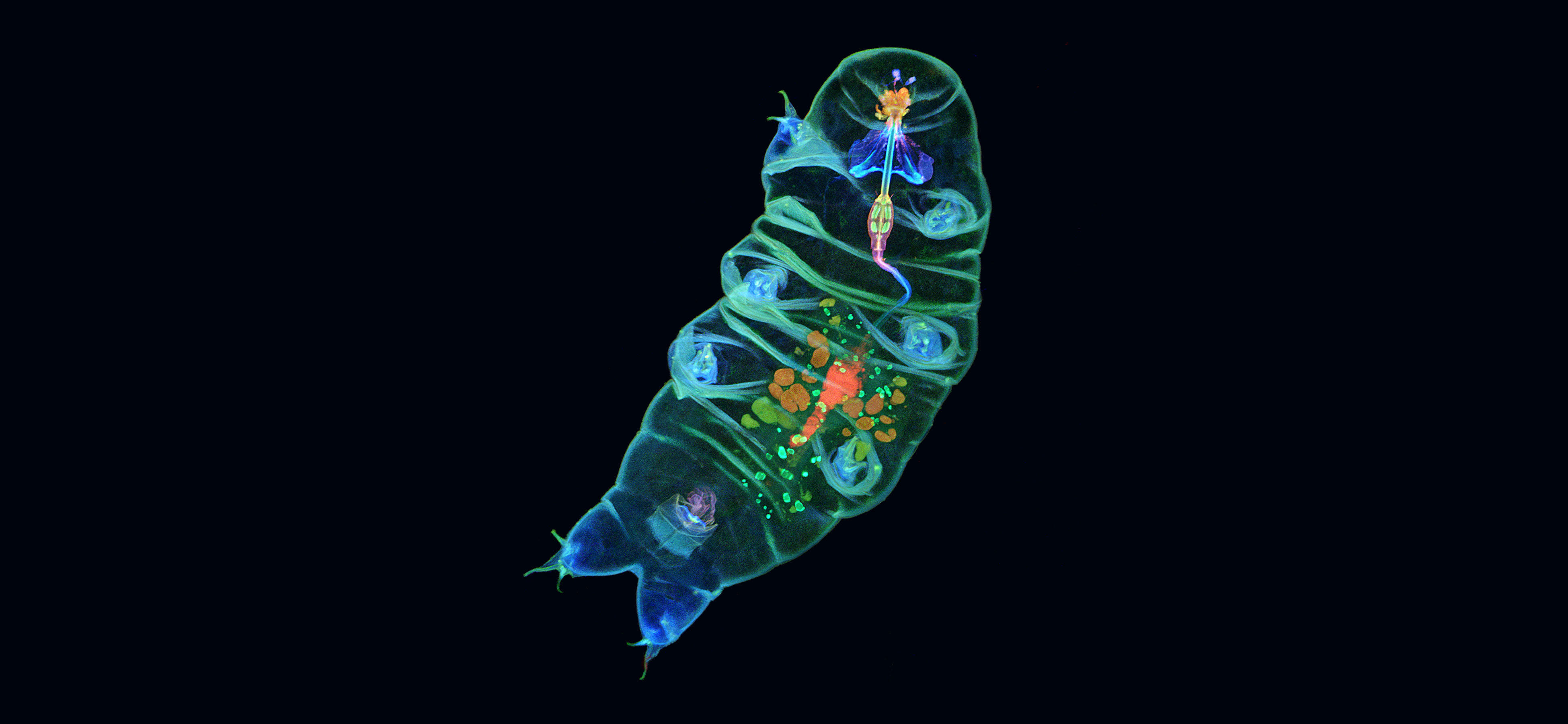
Listen to the Podcast
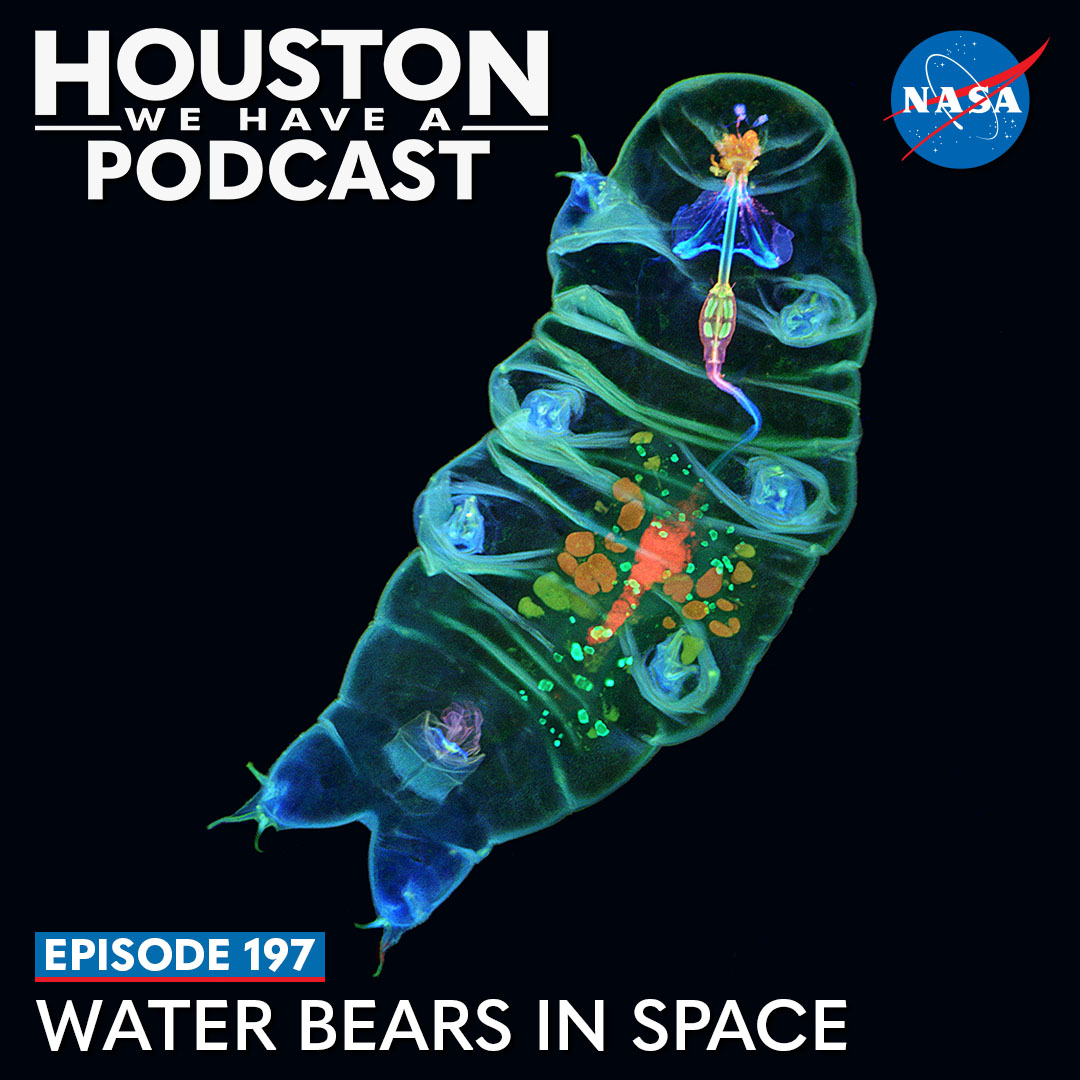
If you’re fascinated by the idea of humans traveling through space and curious about how that all works, you’ve come to the right place.
“Houston We Have a Podcast” is the official podcast of the NASA Johnson Space Center from Houston, Texas, home for NASA’s astronauts and Mission Control Center. Listen to the brightest minds of America’s space agency – astronauts, engineers, scientists and program leaders – discuss exciting topics in engineering, science and technology, sharing their personal stories and expertise on every aspect of human spaceflight. Learn more about how the work being done will help send humans forward to the Moon and on to Mars in the Artemis program.
On Episode 197, Thomas Boothby, assistant professor for the Department of Molecular Biology at the University of Wyoming, teaches us about tardigrades, more commonly known as water bears, that are headed up to the International Space Station for a scientific study to learn how these extremophiles adapt to microgravity. This episode was recorded on May 6, 2021.
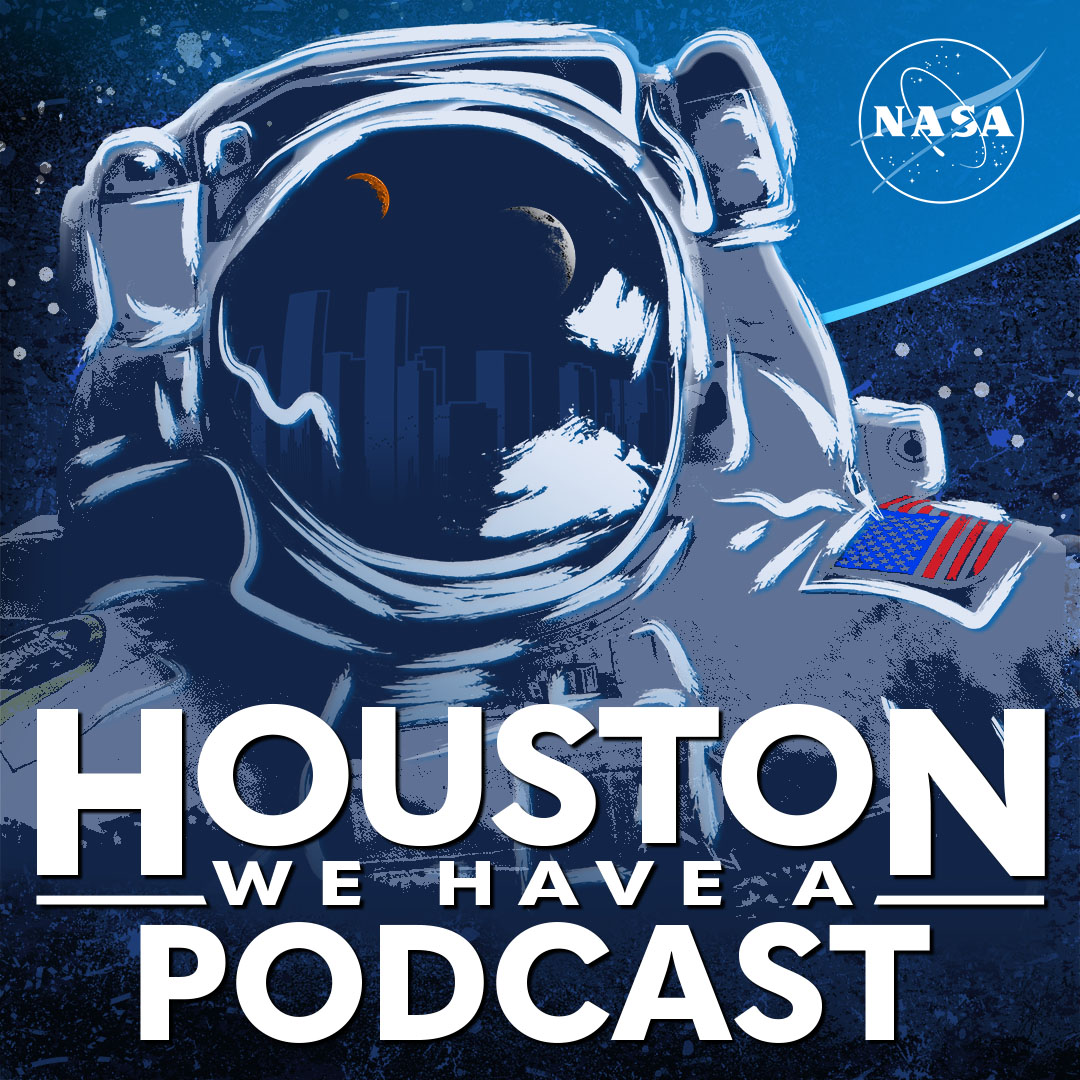
Gary Jordon (Host): Houston, we have a podcast. Welcome to the official podcast of the NASA Johnson Space Center, Episode197, “Water Bears in Space.” I’m Gary Jordan and I’ll be your host today. On this podcast we bring in the experts, scientists, engineers, and astronauts, all to let you know, what’s going on in the world of human spaceflight. Water bears are about to head to the International Space Station. If you’re not familiar with water bears, or tardigrades, they are super-tiny animals that are best known for their ability to survive in some of the harshest conditions: extreme heat, extreme cold, bottom of the ocean, near volcanoes, highly radioactive environments, and even the vacuum of space. Exactly how they survive in these conditions is something that Dr. Thomas Boothby has been studying for years. Thomas is an assistant professor at the University of Wyoming Department of Molecular Biology, and he’s taking his research to the International Space Station as the principal investigator for Cell Science-04, which is, you guessed it, sending water bears to space to study how they adapt to microgravity. I got a chance to chat with Thomas about water bears and this investigation that will be making its way to the space station aboard the SpaceX Dragon on the upcoming CRS-22 mission, so let’s get right to it. Enjoy.
Host: Dr. Thomas Boothby, thanks for coming on Houston We Have a Podcast today.
Thomas Boothby: Absolutely, my pleasure to be here.
Host: Hey, this mission that’s going to be carrying your experiment to the International Space Station is right around the corner, about to launch. How are you feeling in anticipation of this, of this launch coming up?
Thomas Boothby: Me, personally, I’m extremely excited. We’ve been working on this since 2015, so a lot of hard work and time has gone into this, and really exciting that the launch is right around the corner.
Host: Well, let’s get right into it, Thomas. We’re going to be talking about water bears today and I got to say, I am a huge fan. I discovered them like a, I think it was back, man, it was a couple years ago. “Animal Planet” did this, did this show called “The Most Extreme,” and they did one on like the most extreme survivors, and that’s where they just went deep into the survivability of a water bear. And I was like absolutely fascinated, I could not believe what these things were capable of surviving in. So, let’s just start there, understanding water bears, tardigrades, because that will sort of help us transition into this specific science investigation that’s going to space station. So, let’s start with Tardigrades 101, Thomas, take us through what these things are.
Thomas Boothby: Well, so, tardigrades, or water bears as they’re sometimes commonly referred to as, are a group of microscopic animals that are capable of surviving some of the harshest conditions that we know of. So, despite being these like teeny, tiny, little microscopic organisms that you need a microscope to see, they’re extremely robust, so they can survive a number of extremes that we typically think of as being restrictive to life. So, some examples of sort of extreme environments or conditions that tardigrades can survive include being dried out to the point where they essentially have no water left inside their body or cells; they can be frozen down to just above absolute zero, they can be heated up, in some cases, past the boiling point of water, they can survive thousands of times as much radiation as you or I could; they can go days or weeks with little or no oxygen, and maybe the sort of most remarkable feat that they’ve been shown to perform is that they can actually survive in the vacuum of outer space. They’re the only animal that we know of that can, that can do this, so they’re really, they’re really quite amazing and unique.
Thomas Boothby: So, if I were to, if you were to have a picture of a tardigrade, how would you, how would you describe sort of what they look like?
Thomas Boothby: Yeah, so, what I tell people is think about the little, like gummy bear candy, and imagine that, but with eight legs instead of four. They look like these kind of chubby, little eight-legged gummy bears. Yeah, I think, you know, they’re pretty adorable. If people have seen pictures of them, they’re pretty charismatic, but that’s usually how I describe them.
Host: Yeah, and they’re, I mean the pictures I’ve seen of them, they kind of look clear, right?
Thomas Boothby: Yeah, so depending on what kind of microscope you’re using to look at them, if you’re using like a light microscope, many tardigrades are transparent, so you can, you can see through them. Others aren’t, so different species of tardigrades actually, like morphologically, like how they look, is pretty distinct. You have some that, yeah, as you said, there’s kind of clear. You have others that almost look like they have like armored plates on their backs; they look like little tanks, and those are a little bit harder to see through, but yeah, there’s actually quite a bit of a sort of a morphological diversity within the group of animals.
Host: So, in the beginning of this chat you talked about all of these different, very extreme conditions that the water bears can survive in. So, if I were to go hiking around planet Earth, where could I find them?
Thomas Boothby: So, tardigrades have actually been found almost any, anywhere and everywhere that folks have looked for them. They’ve been found, you know, on the tops of mountains, like in the Himalayas, in the deep ocean, in mud volcanoes, tropical rainforests, in Antarctica, but amazingly, if you just go in your backyard, they’re probably living back there.
Host: Oh, wow, I didn’t realize they were, they were so widespread.
Thomas Boothby: Absolutely.
Host: So, really, when it comes to the extreme stuff, right, I guess, my backyard, I wouldn’t really consider that very extreme, but let’s just say, you know, like near a volcano or in like a very high-pressure environment, you know, what are they doing? Are they just sort of swimming around or do they go into some sort of process to help them survive these extreme conditions?
Thomas Boothby: Yeah, so one of, one of the tardigrade’s sort of greatest tricks is this ability to go into an ametabolic state, so a state where, essentially, they shut down all the sort of life processes that are going on inside of them. And when they do this, they pull their eight little legs and head inside their cuticle, that’s sort of like their exoskeleton that surrounds their body. And they curl up in this little ball-like structure known as a tun. So, have you ever seen like one of these little like roly-poly bugs?
Host: Yeah.
Thomas Boothby: They’re kind of like a tiny, little, microscopic version of that, where they curl up in this little ball, they shut down all their life processes that are going on and, you know, for all intents and purposes, you know, they sort of, it’s almost like they’re dead, but they’re in this state, they’re extremely resilient, and they’re able to ride out the, the sort of rough, harsh, extreme conditions. So, you know, if that’s a desiccating environment where water is being lost, you know, they’ll curl up in this little ball-like structure, dry out, and then, you know, when water returns to the environment, they uncurl. They come out of this ball-like structure and within an hour or so, you’ll see them scrambling around, eating, reproducing like nothing happened to them.
Host: Unbelievable. I’m sure you’ve been studying this for a long time, so, you know, you talk about when water is reintroduced to environment, or they’re, you know, they’re in a better environment where they can get out of this tun. How long have you seen some of these water bears in this state, before they return back to, you know, kind of frolicking through, and eating, and reproducing and all that?
Thomas Boothby: Yeah, so, tardigrades are able to enter this ametabolic state, and many species are extremely stable and viable in that, in that state. So, kind of an average would be about a decade or so in this, in this ametabolic state, but there are reports that tardigrades have been shown to survive, you know, for like over a hundred years; these were experiments going into herbariums, where they had preserved plant material, and people gathered tardigrades off that preserved plant material, which, you know, was documented and cataloged, when it was gathered and preserved. And they’ve been able to purportedly revive tardigrades that are, you know, hundreds of years old.
Host: Unbelievable. Now, I mean, it’s, this is a very unique trait for an animal. You know not everyone, not every—every one —every animal can do this. So, what is it about the tardigrade? What unique quality do they possess to be able to do this sort of thing?
Thomas Boothby: Well, that’s a really excellent question, and that’s something that my lab and other labs are trying to uncover. We’ve found some hints and clues, but certainly there’s a lot more to learn. But one of the really interesting features of tardigrades that we found was, that when they start to dry out or enter these sorts of extreme environments, they start to produce a very special class of protein. And this is actually a type of protein that is unique to tardigrades. So, so no other organisms that we’ve, that we’ve looked at possess similar, similar proteins. And what these proteins do is something very interesting: they build up in their concentration. So, the tardigrades just start making more and more and more of these things. And what these proteins seem to do is they make the environment inside the tardigrade, so like inside the tardigrade cells, really, really viscous. So, imagine, you know, as opposed to water, which is very liquid, imagine it more like becoming like honey, where it’s very sort of gooey and viscous. And what this sort of increased viscosity does is it slows down all the bad things that are happening. So, you know, parts of cells start to break down, or unfold, or fuse together, normally, when a cell is drying out, but in this sort of super-viscous environment all those things are still happening, they’re just happening very, very slowly. And when this super, sort of super-viscous environment gets even drier, it, what it does is it forms a glass, so like glass in a windowpane, and this is really important because glass has a very different molecular makeup than say something like a crystal. So, if tardigrades made something that filled their cells with crystals, that would be really bad because crystals are very sharp and pointy, they can puncture cells or crush, you know, sensitive material inside of the tardigrade cells, but these glasses are much smoother and sort of more amorphous, and they’re actually able to encapsulate these sensitive molecules inside of tardigrade cells, and actually preserve them within this sort of glass-like matrix or structure. And what’s really amazing is, when water returns to the system, when you rehydrate a tardigrade, that glassy material just kind of melts away, and it goes back into solution, it dissolves into the, into the water, and it releases all those sensitive molecules that were stabilized inside of it back into the tardigrade cell, where they can perform their normal biological functions.
Host: Thomas, this is, this is amazing. I mean, I, my next question I feel like is a genuine one, but I feel like just everything you’ve just described sort of answers it for me, just how interesting this is, but what got you interested in this fascinating world of researching tardigrades?
Thomas Boothby: Yeah, that’s a great question. So, so besides tardigrades just being, you know, at least to me, like really fascinating, you know, wanting to understand kind of the, the outliers in biology, right, like, you know, tardigrade biology is quite unique, and in my opinion, understudied, and so, you know, just from a purely, from a place of pure intellectual curiosity, understanding how these little creatures are able to do something that, you know, for us, would be so sort of mind-bogglingly impossible to achieve is, was really, really of interest to me. And then, beyond sort of the fundamental biology of tardigrades, I was really attracted to studying them because of some of the potential applications, you know: what we could do in terms of taking the fundamental biological findings that we made studying tardigrades, and sort of the promise of applying that knowledge to trying to solve real world problems was really, really sort of attractive to me.
Host: So, tell me about, you’re, you’re at the University of Wyoming, right? So, you sort of went and described a little bit more about this protein, and you mentioned that you’re still doing a lot more research to figure out exactly what’s going on to allow the tardigrade to have this sort of unique process. So, tell me about some of your research that you’re doing over there.
Thomas Boothby: Absolutely. So, we’ve got, we’ve got quite a bit of sort of diverse research going on here. On sort of the fundamental biological side, we’re really interested in understanding how these tardigrade proteins are working. So, like, what are the building blocks that make up these proteins that make them so special and so protective? We’re also really interested in understanding whether or not these proteins and other tricks that tardigrades use to, say, survive when they dry out, we’re really interested to know if those are the same tricks that tardigrades use when they’re faced with other extremes, like freezing, for example. So, do tardigrades have sort of one, one way of surviving many different extremes or do they have many different tricks for surviving all these different extremes? And then on the more applied side, we’re really interested in how we can take that knowledge and adapt it to addressing real world problems, like stabilizing pharmaceuticals, or developing crops that are more resistant to extreme environments, so that’s kind of our research, in a nutshell.
Host: So I imagine, you mentioned there are tardigrades all over the world and you want to understand more about the, some of these different processes, or at least when they hibernate, or I guess, go into this, you said, I forget the exact state, something about metabolism, but essentially, into this state, and in this tun, do you get to travel to some of those locations as part of your research, like to, you know, volcanoes, or to whatever, deep sea, and understand, like pressure, or are you bringing them to the lab and doing everything in the, at your university?
Thomas Boothby: Yeah, so, so a little bit of both. So, a couple years ago, as part of a NSF (National Science Foundation) training, training grant, I was able to go down to Antarctica, and we were finding tardigrades down there, along with doing some experiments. One of the reasons that our lab located to Wyoming was to be closer to some of these extreme environments that we study organisms from. So, Wyoming has a number of really diverse extreme environments. You know, people typically think of, you know, sort of the hot springs out in Yellowstone, but then there’s also Wyoming’s Red Desert, which is an immense, high-elevation desert, so, so very cold and very dry, with sort of Martian-like environments. And then, of course, you have the Bighorn Mountains, the Snowy Range Mountains, so, you know, we kind of have all different types of environments out here in Wyoming where we go in and collect organisms from.
Host: That’s pretty cool. Yeah, you got to enjoy those kinds of trips then, you got to enjoy the harsh environments.
Thomas Boothby: Absolutely. Yeah, it helps, it helps to be a little bit tough if you want to go and study these little critters out there.
Host: Well, look, the space station is only 250 miles from Wyoming, you just got to go straight up. So, how did it happen where you were looking at all these different extreme environments and you thought, ah, you know, where we should go is the space station?
Thomas Boothby: Yeah, so, you know, how that kind of came about was, I was just really curious in this observation that tardigrades actually survive a number of extremes that they would never have been exposed to, so it’s kind of this perplexing question of, you know, how could an organism evolve to tolerate a condition that it, it didn’t evolve in? And spaceflight and space environments are probably some of the sort of most foreign or alien environments that you can think of for an organism that evolved on Earth. And so, there have been some space studies using tardigrades before. In particular there was a, there was a Russian capsule that went up in 2007, which actually exposed tardigrades to the vacuum of space, and they were left out there for about ten days in low-Earth orbit, and they were shown to be viable after that exposure. There was another mission involving some Italian scientists, which showed that tardigrades could survive and reproduce without any negative effects during spaceflight. And so, yeah, I got really interested in trying to understand how, right, not just, can they do this, but how are they able to do this? And so, that’s really, kind of the, kind of main driving scientific question for Cell Science-04 mission, is understanding how tardigrades adapt to being exposed to outer space, or to space conditions, rather. And then under those prolonged spaceflight conditions, how do they change and adapt after that initial exposure, you know, say over multiple generations?
Host: So, let’s get into it, let’s get into the experiment that’s going on the International Space Station, you called it Cell Science-04. So, what’s the, what’s this experiment that’s going up?
Thomas Boothby: Yeah, so what we’re really interested in doing is looking at what the initial changes in gene expression, so, so how tardigrades are adapting to spaceflight environments, is initially, and then how that changes over multiple generations. So, essentially, what we’re going to be doing is sending tardigrades up from the Kennedy Space Center to the ISS, and we’re going to basically have two different pools of tardigrades. One pool is going to be our sort of founding generation, where after a week of being in space, we’re going to preserve them in a special chemical preservative, but then the second pool we’re going to let culture, and grow, and reproduce for two months, and that’ll represent about four generations of tardigrades. So, they’ll have time to reproduce, and their offspring will reproduce, and so on, and so forth, for four generations. And then we’re going to preserve those multigenerational tardigrades. And when we get these preserved tardigrades back to our lab here in Wyoming, what we do is we extract a certain molecule called RNA, and this is kind of an intermediate molecule between the tardigrade’s DNA, their genes, and the final products that those genes are sort of the blueprint to make. And so, by looking at these molecules that we can extract, we can tell what changes in gene expression tardigrades are inducing when they’re exposed to space initially, and when they’re exposed to spaceflight conditions over the long term. And our hope is that by understanding how these tough little organisms are able to survive spaceflight conditions, that this will give us hints and clues into, you know, how we might safeguard astronauts during prolonged space missions.
Host: See, that’s going to be a big deal, especially for NASA’s plans to go to the Moon and Mars, just one extra step to help out in that process, very, very fascinating stuff.
Host: I’m curious to hear about how you’ve been preparing to get this experiment going. You know, you, I guess had to start with the initial process of figuring out how to get the tardigrades into space, but what’s been the process from the initial concept, to getting everything packed, and basically, ready to go on a rocket?
Thomas Boothby: Yeah. So, so initially, kind of the biggest consideration was just trying to figure out how we’re going to grow these little animals in space. So, in the lab, we normally grow them in these sorts of big glass petri dishes filled with a liquid medium, but in space that wouldn’t work so much because the, in microgravity the liquid media that the tardigrades grow in would just sort of float away. So, yeah, initially, it was validating a bioculture system that had been developed by some NASA engineers and adapted to this project. And then, it was really just going through a number of sort of dry runs and seeing, you know, in ground-based experiments, how our experimental plan for the actual flight experiment went. You know, it was a lot of optimizing things that don’t sound very exciting, like how fast a pump moves water through the system, or how much oxygen we need to deliver to the media. But, you know, all that sort of nitty-gritty detail has been worked out and we’re now, actually, this, just this week, in the process of prepping our samples that will go up to the ISS, so that basically involves loading the tardigrades into syringes that will be frozen and can be stored frozen and delivered to the ISS in this sort of inactive state. And then along with that we’re packaging up a lot of the food that the tardigrades eat because, over multiple generations, they’re going to need to be fed a couple times to stay healthy. So, the species of tardigrade that we use, they eat unicellular algae, so little, little algal cells, so we’re also getting those loaded into syringes, and ready to be sent up to the space station.
Host: So, actually, running the experiment on station, it sounds like this, whatever setup you have is going to be installed on a facility on space station, and every once in a while, is it going to require astronaut interaction to go ahead and use this syringe and feed the water bears, over generations?
Thomas Boothby: Absolutely. So, yup, when the, when our samples get up to the space station, they’ll be in syringes, and the astronauts will need to thaw out the tardigrades to sort of reactivate them, and then inject them into this bioculture system. Once they’re in the bioculture system, it’s pretty hands-off. We have telemetry, so we can sort of monitor the temperature and the flow rates and everything, inside the bioculture system. But then, yeah, you’re exactly right, at sort of two-week intervals an astronaut is going to need to attach an algal syringe to the bioculture system and inject fresh algae into it for the, for the tardigrades to eat, but, and then, and then at the end of the experiment they’re going to need to essentially sort of dismantle a portion of that bioculture system, which will be frozen and stored until we can get it back at our lab in Wyoming. So, there will definitely be some astronaut interaction with this, with this experiment, but there are also sort of large portions that are, that are automated.
Host: Yeah. Honestly, it sounds pretty easy in terms of the astronaut crew time needed, just, you know, feed it, it sounds like not even that often. You said once every two weeks was the feeding schedule?
Thomas Boothby: Yup.
Host: Yeah, see, it’s not even that bad. When it comes to measuring though, you said you’re going to, it is going to return, that’s part of the plan is it returns back to Earth, you go to the lab, and you have a number of things that you’re going to be looking at. Is there anything on orbit that will be, that you have in terms of measuring tools? It sounds like you have the, in the facility, you have the ability to control climate and watch all data coming in, but are you going to be doing any data gathering from the facility while it’s in orbit?
Thomas Boothby: So, the only sort of measurements that we’re making on orbit are environmental measurements. So, you know, what the, what the environment that the bioculture system is in. And we’re going to be using that telemetry, so, you know, the temperature, and whatnot, to replicate those experiments back here on Earth, and so we call those our near-synchronous ground controls. So we’re basically going to be doing the exact same experiment, but here on Earth, almost in real time with the, with the flight mission. But yeah, all the, all the sort of biological data that will be gathered is going to be done once we get the samples back from the space station, then we’ll process them here, here in the lab in Wyoming.
Host: So, you said — you said we, right? So, it’s not just, it’s not just you sending the water bears up and looking at everything. It sounds like you got, you got a decent support team that’s helping to bring all of this together.
Thomas Boothby: Yeah, absolutely. And yeah, it’s really great that you bring that up because, you know, I’m just one person in a team of really amazing folks. So, here, in the lab, in Wyoming, specifically, we have Cherie Hesgrove and Ryan Bettcher are working on this project. But then, on the NASA and the KBR side of things, we have a lot of people who really, sort of deserve some credit. So, specifically, Medaya Torres is our CS-04 mission scientist, Natayla Dvorochkin is our contract support scientist, and then on the KBR side there’s a bunch of people that I’d just like to acknowledge really quickly: Daniel Nolan, Kevin Sims, Oscar Roque, Christina Lim, Crystal Kumar, Kris Vogelsong, Brandon Schmitt, and Jamie Bales, Susan Markey, Meghan Feldman. And then on the NASA engineering side, Peter Zell and David Pletcher. And I’m sure I’m forgetting some other folks, but it’s been a, it’s been a huge team and group effort to get to this point, and yeah, I think it’s worth taking a couple minutes just to acknowledge all these folks.
Host: Absolutely. Yeah, and that’s part of the whole deal, right, is it’s not just, you know, it’s not just, “hey, Thomas, let’s get your experiment on the International Space Station,” it does really take a team, not only to get it up there, but to do all the work, to monitor it, make sure it’s working fine, and then of course, what you, what you’re all anticipating is when you get the water bears back from space into the lab in Wyoming and you get to conduct some fascinating research from that, from that group of water bears that went up there.
Host: Yeah. Now, one of the things I’m thinking of, Thomas, is, you know, there’s, well, I think what we’re all anticipating is when you’re starting that research, you know, what are the potential applications that you are thinking of in terms of maybe something we can learn, that we can bring back to benefit us here on Earth, or something that we can use to further space exploration. What are some of the things that you’re looking at that might have potential benefits to this experiment?
Thomas Boothby: Yeah. Well, definitely, part of the sort of stated goal of this mission is, you know, to start to build a foundation for developing therapies or countermeasures that might better safeguard astronauts in the future during prolonged space missions. So, you know, as I sort of mentioned before, spaceflight can be a really challenging sort of environment for organisms, including humans, who have evolved to the conditions on Earth. So, in space, you have much less gravity, you’re in microgravity, and you’re also exposed to a lot more radiation. So, for humans who spend a lot of time in space, you know, there can be detrimental effects to being in these environments. And so, one of the things we’re really keen to do is understand, you know, how are tardigrades surviving and reproducing in these environments, and can we learn anything about the tricks that they’re using that might be adapted to safeguarding astronauts. So, for example, if we see that tardigrades, when exposed to sort of this increased radiation in space, which produces a lot of reactive oxygen species, which are these sort of damaging chemical moieties that are really bad for cells, if tardigrades are producing a lot of reactive oxygen species scavengers, which basically kind of negate those negative effects, then that might be something that we would consider either through, you know, like a dietary supplement, or something like that, providing astronauts with increased antioxidants or reactive oxygen species scavengers. That would just help them stay healthier in space for longer.
Host: See, this makes me think about this experiment and this, like you said, you want to, you want to set a foundation, right, that’s what you were talking about whenever you were thinking of potential application. And I think that’s a very exciting thing to say because what makes me, it makes me think that this is scalable, right? You can continue the research, maybe, maybe bringing next cell science investigations up to the International Space Station. And you were just mentioning the radiation environment, which in low-Earth orbit is a little bit different from say, the Moon. And with the Artemis program, with NASA returning to the Moon, there are potential, there are potential options to have investigations like this, where you can study water bears in an even higher radiation environment and gather even more unique data. So, it, to me it sounds like this is something that you can continue for a while.
Thomas Boothby: Absolutely, we hope so. I think, you know, there’s a lot more to learn about tardigrades and a lot of, you know, continuing potential benefits to society.
Host: And that’s a, that’s such a big deal and it’s all happening on board the International Space Station coming here real soon. So, Dr. Thomas Boothby, thank you again for coming on Houston We Have a Podcast. And really, best of luck to you and your team as you gear up for this launch of a, on a SpaceX Cargo Dragon to the International Space Station. Best of luck to you as your, as your journey just begins for Cell Science-04.
Thomas Boothby: Great. Thanks very much.
Host: Hey, thanks for sticking around. I hope you learned something about water bears and you’re as excited as I am for this launch of CRS-22. You can watch these water bears launch from Florida, travel to the International Space Station. Just check out our website NASA.gov/ntv has the latest on our TV schedule when you can see the launch live. If you like this podcast, we are one of several NASA podcasts across the entire agency; you can check all of them out at NASA.gov/podcasts . We, Houston We Have a Podcast, are on the Johnson Space Center pages of Facebook, and Twitter, and Instagram. So, if you want to talk to us, just use the hashtag #AskNASA on your favorite platform, you can submit an idea or ask us a question, just make sure to mention it’s for us at Houston We Have a Podcast. This episode was recorded on May 6, 2021. Thanks to Alex Perryman, Pat Ryan, Norah Moran, Belinda Pulido, Jennifer Hernandez, Rachel Barry, and the International Space Station Program Research Office for helping to set us up with Thomas. And, of course, thanks again to Dr. Thomas Boothby for taking the time to come on the show. Give us a rating and feedback on whatever platform you’re listening to us on and tell us what you think of our podcast. We’ll be back next week.

Tardigrades (Water Bears)
Created by Sarah Bordenstein, Marine Biological Laboratory
Strange is this little animal, because of its exceptional and strange morphology and because it closely resembles a bear en miniature. That is the reason why I decided to call it little water bear.
- J.A.E. Goeze (Pastor at St. Blasii, Quedlinburg, Germany), 1773
What is a Tardigrade - Where to Find - How to Find - Tardigrades in Extreme Environments - Resources
What is a Tardigrade?
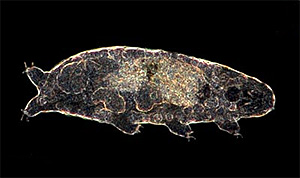
Tardigrades (Tardigrada), also known as water bears or moss piglets , are a phylum of small invertebrates. They were first described by the German pastor J.A.E. Goeze in 1773 and given the name Tardigrada, meaning "slow stepper," three years later by the Italian biologist Lazzaro Spallanzani. Tardigrades are short (0.05mm - 1.2mm in body length), plump, bilaterally symmetrical, segmented organisms. They have four pairs of legs, each of which ends in four to eight claws. Tardigrades reproduce via asexual ( parthenogenesis ) or sexual reproduction and feed on the fluids of plant cells, animal cells, and bacteria. They are prey to amoebas, nematodes, and other tardigrades. Some species are entirely carnivorous! Tardigrades are likely related to Arthropoda (which includes insects, spiders, and crustaceans) and Onychophora (velvet worms), and are often referred to as a "lesser known taxa" of invertebrates . Despite their peculiar morphology and amazing diversity of habitats, relatively little is known about these tiny animals. This makes them ideal research subjects for which students and amateur microscopists may contribute novel data to the field.
Back to Top
Where to Find Tardigrades
Tardigrades can be found in almost every habitat on Earth! With over 900 ( more info ) described species, the phylum has been sighted from mountaintops to the deep sea, from tropical rain forests to the Antarctic. Most species live in freshwater or semiaquatic terrestrial environments, while about 150 marine species have been recorded. All Tardigrades are considered aquatic because they need water around their bodies to permit gas exchange as well as to prevent uncontrolled desiccation. They can most easily be found living in a film of water on lichens and mosses, as well as in sand dunes, soil, sediments, and leaf litter.
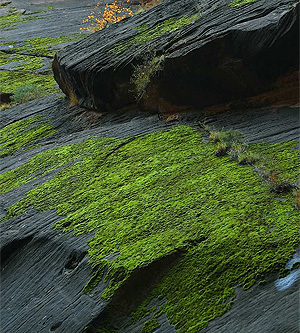
How to Find Terrestrial Tardigrades
- Collect a clump of moss or lichen (dry or wet) and place in a shallow dish, such as a Petri dish.
- Soak in water (preferably rainwater or distilled water) for 3-24 hours.
- Remove and discard excess water from the dish.
- Shake or squeeze the moss/lichen clumps over another transparent dish to collect trapped water.
- Starting on a low obejctive lens, examine the water using a stereo microscope.
- The Water Bear web base
- Hunting for Bears in the Backyard
Tardigrades in Extreme Environments
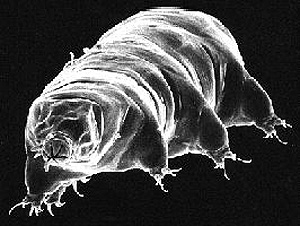
- temperatures as low as -200 ° C (-328 ° F) and as high as 151 ° C (304 ° F);
- freezing and/or thawing processes;
- changes in salinity;
- lack of oxygen;
- lack of water;
- levels of X-ray radiation 1000x the lethal human dose;
- some noxious chemicals;
- boiling alcohol;
- low pressure of a vacuum;
- high pressure (up to 6x the pressure of the deepest part of the ocean).
How do they do it?
Tardigrades have adapted to environmental stress by undergoing a process known as cryptobiosis . Cryptobiosis is defined as a state in which metabolic activities come to a reversible standstill. It is truly a death-like state; most organisms die by a cessation of metabolism. Several types of cryptobiosis exist, the most common include:
- anhydrobiosis (lack of water);
- cryobiosis (low temperature);
- osmobiosis (increased solute concentration, such as salt water);
- anoxybiosis (lack of oxygen).
The most common type of cryptobiosis studied in tardigrades is anhydrobiosis. Anton van Leeuwenhoek first documented cryptobiosis in 1702, when he observed tiny animalcules in sediment collected from house roofs. He dried them out, added water, and found that the animals began moving around again. The animalcules were likely nematodes or rotifers, other types of cryptobiotic animals. Tardigrades can survive dry periods by curling up into a little ball called a tun. Tun formation requires metabolism and synthesis of a protective sugar known as trehalose, which moves into the cells and replaces lost water. While in a tun, their metabolism can lower to less than 0.01% of normal. Revival typically takes a few hours, depending on how long the tardigrade has been in the cryptobiotic state.
Live tardigrades have been regenerated from dried moss kept in a museum for over 100 years! Once the moss was moistened, they successfully recovered from their tuns. While tardigrades can survive in extreme environments, they are not considered extremophiles because they are not adapted to live in these conditions. Their chances of dying increase the longer they are exposed to the extreme environment.
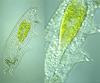
Learn more about Tardigrades with this collection of resources including informational websites, primary literature, and educational activities.
Additional Resources
For additional resources about Tardigrades, search the Microbial Life collection.
Back to Top

- Stay Connected

Home / Science & Nature / Animals /
Study determines microscopic water bears will be Earth’s last survivors
In Animals , Marine Science , Research News , Science & Nature , Space , Spotlight / 19 July 2017
By Megan Watzke
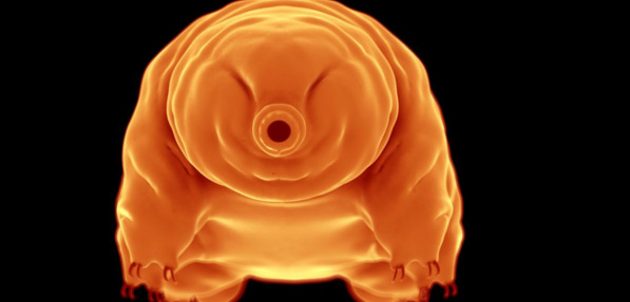
The world’s most indestructible species, the tardigrade, an eight-legged micro-animal, also known as the water bear, will survive until the Sun dies, according to a new Harvard-Smithsonian Center for Astrophysics and Oxford University collaboration.
The world’s most indestructible species, the tardigrade, an eight-legged micro-animal, also known as the water bear, will survive until the Sun dies, according to a new Harvard-Smithsonian Center for Astrophysics and Oxford University collaboration.
The new study published in Scientific Reports, has shown that the tiny creatures will survive the risk of extinction from all astrophysical catastrophes, and be around for at least 10 billion years–far longer than the human race.
Although much attention has been given to the cataclysmic impact that an astrophysical event would have on human life, very little has been published around what it would take to kill the tardigrade, and wipe out life on this planet. The research implies that life on Earth in general, will extend as long as the Sun keeps shining. It also reveals that once life emerges, it is surprisingly resilient and difficult to destroy, opening the possibility of life on other planets.
Tardigrades are the toughest, most resilient form of life on earth, able to survive for up to 30 years without food or water, and endure temperature extremes of up to 150 degrees Celsius, the deep sea and even the frozen vacuum of space. The water-dwelling micro animal can live for up to 60 years, and grow to a maximum size of 0.5 mm, best seen under a microscope.
Researchers from the Universities of Oxford and the Harvard-Smithsonian Center for Astrophysics, have found that these life forms will likely survive all astrophysical calamities, such as an asteroid, since they will never be strong enough to boil off the world’s oceans.
Three potential events were considered as part of the research, including; large asteroid impact, and exploding stars in the form of supernovas or gamma-ray bursts.
Asteroids: There are only a dozen known asteroids and dwarf planets with enough mass to boil the oceans, these include Vesta and Pluto, however none of these objects will intersect the Earth’s orbit and pose no threat to tardigrades.
Supernova: In order to boil the oceans an exploding star would need to be 0.14 light-years away. The closest star to the Sun is four light years away and the probability of a massive star exploding close enough to Earth to kill all forms of life on it, within the Sun’s lifetime, is negligible.
Gamma-Ray bursts: Gamma-ray bursts are brighter and rarer than supernovae. Much like supernovas, gamma-ray bursts are too far away from earth to be considered a viable threat. To be able to boil the world’s oceans the burst would need to be no more than 40 light-years away, and the likelihood of a burst occurring so close is again, minor.
“Without our technology protecting us, humans are a very sensitive species. Subtle changes in our environment impact us dramatically. There are many more resilient species’ on earth. Life on this planet can continue long after humans are gone,” says Rafael Alves Batista, co-author and post-doctoral research associate in the Department of Physics at Oxford University. “Tardigrades are as close to indestructible as it gets on Earth, but it is possible that there are other resilient species examples elsewhere in the universe. In this context there is a real case for looking for life on Mars and in other areas of the solar system in general. If tardigrades are Earth’s most resilient species, who knows what else is out there.”
David Sloan, co-author and post-doctoral research associate in the Department of Physics at Oxford University, said: “To our surprise we found that although nearby supernovae or large asteroid impacts would be catastrophic for people, tardigrades could be unaffected. Therefore it seems that life, once it gets going, is hard to wipe out entirely. Huge numbers of species, or even entire genera may become extinct, but life as a whole will go on.”
In highlighting the resilience of life in general, the research broadens the scope of life beyond Earth, within and outside of this solar system. Abraham Loeb, co-author and chair of the Astronomy Department at Harvard University, said: “It is difficult to eliminate all forms of life from a habitable planet. Organisms with similar tolerances to radiation and temperature as tardigrades could survive long-term below the surface in these conditions. The subsurface oceans that are believed to exist on Europa and Enceladus, would have conditions similar to the deep oceans of Earth where tardigrades are found, volcanic vents providing heat in an environment devoid of light. The discovery of extremophiles in such locations would be a significant step forward in bracketing the range of conditions for life to exist on planets around other stars.”
A paper detailing this work appeared on July 14, 2017 in Scientific Reports , an open, online journal from the publishers of Nature.
Related posts:
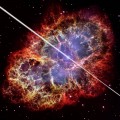
Tags: asteroids , astronomy , astrophysics , Center for Astrophysics | Harvard & Smithsonian , extinction , Smithsonian Astrophysical Observatory

See the U.S.A. on Paik’s “Electronic Superhighway”
Water Bears: The Biology of Tardigrades
- © 2018
- Ralph O. Schill 0

Institute of Biological Materials and Biomolecular Systems, University of Stuttgart, Stuttgart, Germany
You can also search for this editor in PubMed Google Scholar
- Exhaustively reviews virtually every conceivable aspect of tardigrade biology
- Includes field and laboratory methods for studying water bears
- Provides a timely summary on the discovery and history of these animals
Part of the book series: Zoological Monographs (ZM, volume 2)
33k Accesses
222 Citations
278 Altmetric
This is a preview of subscription content, log in via an institution to check access.
Access this book
- Available as EPUB and PDF
- Read on any device
- Instant download
- Own it forever
- Durable hardcover edition
- Dispatched in 3 to 5 business days
- Free shipping worldwide - see info
Tax calculation will be finalised at checkout
Other ways to access
Licence this eBook for your library
Institutional subscriptions
Table of contents (15 chapters)
Front matter, from johann august ephraim goeze to ernst marcus: a ramble through the history of early tardigrade research (1773 until 1929).
- Hartmut Greven
Morphology and Functional Anatomy
- Nadja Møbjerg, Aslak Jørgensen, Reinhardt Møbjerg Kristensen, Ricardo C. Neves
Phylogeny and Integrative Taxonomy of Tardigrada
- Aslak Jørgensen, Reinhardt M. Kristensen, Nadja Møbjerg
Tardigrade Biogeography
- S. J. McInnes, P. J. A. Pugh
Paleontology and Molecular Dating
- Roberto Guidetti, Roberto Bertolani
Cytology and Cytogenetics
- Roberto Bertolani, Lorena Rebecchi
Tardigrade Ecology
- Diane R. Nelson, Paul J. Bartels, Noemi Guil
Reproduction, Development and Life Cycles
- Tiziana Altiero, Atsushi C. Suzuki, Lorena Rebecchi
Environmental Adaptations: Encystment and Cyclomorphosis
- Roberto Guidetti, Nadja Møbjerg
Environmental Adaptations: Desiccation Tolerance
- Ralph O. Schill, Steffen Hengherr
Environmental Adaptations: Cryobiosis
- Steffen Hengherr, Ralph O. Schill
Environmental Adaptations: Radiation Tolerance
- K. Ingemar Jönsson, Eliana B. Levine, Andrzej Wojcik, Siamak Haghdoost, Mats Harms-Ringdahl
Molecular Biology in Tardigrades
- Thomas C. Boothby
Field and Laboratory Methods
- Peter Degma
Tardigrade Taxa
- Peter Degma, Roberto Guidetti
Back Matter
- Tardigrada biodiversity
- anhydrobiosis
- cryptobiosis
- dormant state
- eutardigrades
- heat tolerance
- heterotardigrades
- extreme environments
- parthenogentic
About this book
Offering extensive information on tardigrades, this volume begins with a chapter on the history of tardigrades, from the first description by Goeze in 1773, until 1929, when the most comprehensive monographic approach by E. Marcus was published. Tardigrades’ organ systems, including their integument, body cavity, digestive, muscular, nervous and reproductive systems, as well as their overall external morphology, are summarized in the second chapter. Subsequent chapters present the current state of knowledge on tardigrade phylogeny, biogeography, paleontology, cytology and cytogenetics. In addition, the book provides insights into the ecology of tardigrades in marine, freshwater and terrestrial habitats. The reproduction, development and life cycles are summarized and the extraordinary environmental adaptations of encystment and cyclomorphosis, desiccation tolerance, freezing tolerance and radiation tolerance are discussed in detail. Further chapters provide an overview of key approaches in molecular tardigrade studies and describe techniques for sampling and sample processing. The book closes with a list of tardigrade taxa up to a sub-generic level, including the type species of each genus, the numbers of lower taxa in each taxon, and the main environments in which the taxa were found. Given its depth of coverage, the volume offers an invaluable resource for scientists from various disciplines who plan to research tardigrades, and for all others who are interested in these fascinating animals.
Editors and Affiliations
Ralph O. Schill
About the editor
Bibliographic information.
Book Title : Water Bears: The Biology of Tardigrades
Editors : Ralph O. Schill
Series Title : Zoological Monographs
DOI : https://doi.org/10.1007/978-3-319-95702-9
Publisher : Springer Cham
eBook Packages : Biomedical and Life Sciences , Biomedical and Life Sciences (R0)
Copyright Information : Springer Nature Switzerland AG 2018
Hardcover ISBN : 978-3-319-95701-2 Published: 04 March 2019
eBook ISBN : 978-3-319-95702-9 Published: 14 February 2019
Series ISSN : 2523-3904
Series E-ISSN : 2523-3912
Edition Number : 1
Number of Pages : VIII, 419
Number of Illustrations : 61 b/w illustrations, 57 illustrations in colour
Topics : Zoology , Evolutionary Biology , Animal Ecology
- Publish with us
Policies and ethics
- Find a journal
- Track your research
Academia.edu no longer supports Internet Explorer.
To browse Academia.edu and the wider internet faster and more securely, please take a few seconds to upgrade your browser .
Enter the email address you signed up with and we'll email you a reset link.
- We're Hiring!
- Help Center
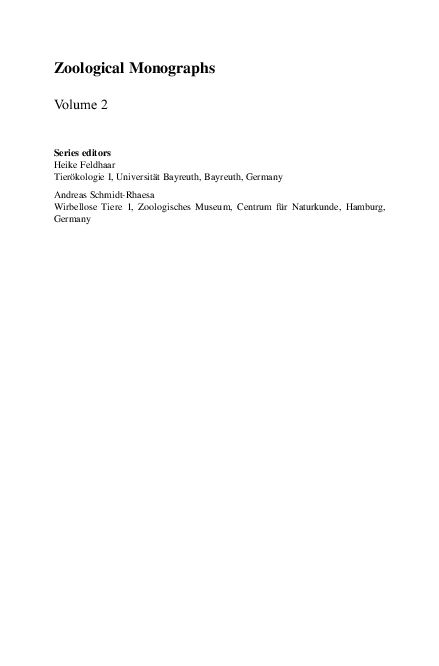
Water Bears: The Biology of Tardigrades

2018, Zoological Monographs
Related Papers
ROBERTO BERTOLANI
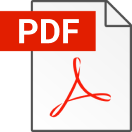
International Journal of Latest Technology in Engineering, Management & Applied Science -IJLTEMAS (www.ijltemas.in)
Tardigrades popularly known as water bears are micrometazoans with four pairs of lobopod legs. They are the organisms which can live in extreme conditions and are known to survive in vacuum and space without protection. Tardigardes survive in lichens and mosses, usually associated with water film on mosses, liverworts, and lichens. More species are found in milder environments such as meadows, ponds and lakes. They are the first known species to survive in outer space. Tardigrades are closely related to Arthropoda and nematodes based on their morphological and molecular analysis. The cryptobiosis of Tardigrades have helped scientists to develop dry vaccines. They have been applied as research subjects in transplantology. Future research would help in more applications of tardigrades in the field of science.
Ingrida Satkauskiene
Journal of Insect Physiology
Roberto Guidetti
Acta Physiologica
Hans Ramløv , Nadja Møbjerg , K. Halberg , D. Persson
Limnological Review
Michaela Czerneková
Tardigrades (phylum Tardigrada) are aquatic microecdysozoans that have adapted to survive extreme conditions through the formation of cysts or ametabolic tuns. Their body is covered by a cuticle that plays an important role in their life cycle, including their response and adaptation to environmental challenges. Cuticular characteristics are a critical component of tardigrade taxonomy. Therefore, research has often been focused on the relationship between cuticular morphology and ultrastructure and the evolutionary and phylogenetic positioning of the phylum and individual species herein. However, a deeper insight into the ultrastructural characteristics and chemical composition of the tardigrade cuticle is needed. This knowledge is important not only for a better understanding of tardigrade physiology and ecology but also for the development of efficient microinjection and/or electroporation techniques that would allow for genetic manipulation, opening new avenues in tardigrade rese...
The tardigrade fauna of Australian marine caves: With descriptions of nine new species of Arthrotardigrada
Reinhardt Møbjerg Kristensen
BMC Evolutionary Biology
Background Tardigrada is a group of microscopic invertebrates distributed worldwide in permanent and temporal aquatic habitats. Famous for their extreme stress tolerance, tardigrades are also of interest due to their close relationship with Arthropoda and Cycloneuralia. Despite recent efforts in analyzing the musculature of a number of tardigrade species, data on the class Heterotardigrada remain scarce. Aiming to expand the current morphological framework, and to promote the use of muscular body plans in elucidating tardigrade phylogeny, the myoanatomy of two heterotardigrades, Actinarctus doryphorus and Echiniscoides sigismundi, was analyzed by cytochemistry, scanning electron and confocal laser scanning microscopy and 3D imaging. We discuss our findings with reference to other tardigrades and internal phylogenetic relationships of the phylum. Results We focus our analyses on the somatic musculature, which in tardigrades includes muscle groups spanning dorsal, ventral, and lateral...
Journal of Zoological Systematics and Evolutionary Research
Nadja Møbjerg
RELATED PAPERS
Molecular Phylogenetics and Evolution
R. Bertolani
Invertebrate Systematics
Frontiers in physiology
Morten Schiøtt
Laura Castillo
Zoological Journal of the Linnean Society
Journal of Limnology
Helgol Mar Res
Kasper Andersen
Martina Montanari
Syed Atif Iqrar
Weronika Erdmann
Marine Biology Research
Kenneth Halberg
European Journal of Taxonomy
Zoological Letters
Annales Zoologici
Dorota Lachowska-cierlik
Sunghyun KANG
Contributions to Zoology
Omar Rota-Stabelli
Journal of Experimental Biology
Kenneth Halberg , Dennis Persson , Nadja Møbjerg , Hans Ramløv
Journal of Morphology
Italian Journal of Zoology
Michele Cesari
Planetary and Space Science
Polar Biology
The European Zoological Journal
Ivan Parnikoza
Invertebrate Zoology
Sandra Mcinnes , Chester Sands , William Goodall-copestake , Peter Convey
Sandra Mcinnes , Chester Sands
Nigel Marley , Sandra Mcinnes
Michele Cesari , Roberto Guidetti
Nigel Marley
- We're Hiring!
- Help Center
- Find new research papers in:
- Health Sciences
- Earth Sciences
- Cognitive Science
- Mathematics
- Computer Science
- Academia ©2024
A Laser Zapped the Rocks on Mars and Revealed a Long-Lost Water World
Evidence suggests the Red Planet once mirrored Earth’s blue oceans.
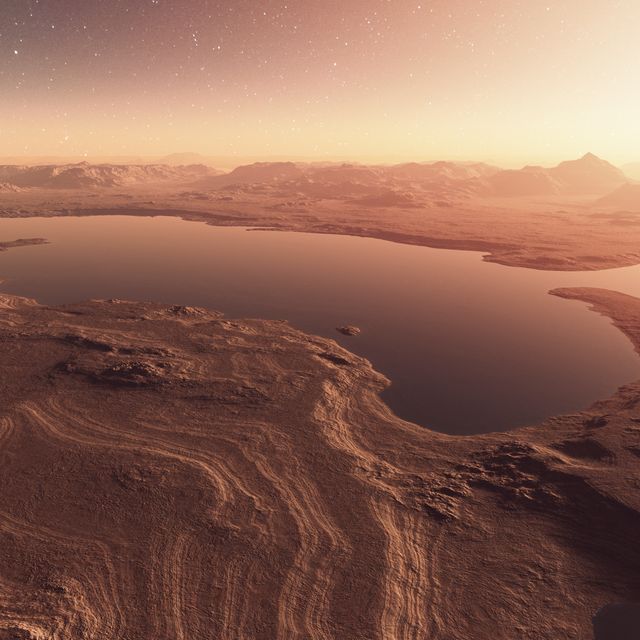
- Around four billion years ago, during its Noarchian geologic period, Mars was likely filled with oceans, rivers, and lakes.
- A new study, analyzing data from a 2017 soil analysis from Gale crater, discovered high levels of manganese, a surprising find as manganese enrichment on Earth often requires oxygen and microbes.
- The study theorizes that water percolation into soils along ancient waterways could’ve created these deposits, and they might’ve even supported ancient microbial life, much like oxidated states of manganese due on Earth today.
Water-filled oceans, meandering river deltas, varying seasons, and an insulating atmosphere are usually descriptors for Earth—the only known rock to support life in the solar system. But during the Noarchian, a period in Mars’ history some 4.1 to 3.5 billion years ago, these descriptions perfectly matched the fourth rock from the Sun. Back then, the not-so-red planet could’ve even supported life. In fact, Mars boasts the oldest known prebiotic, life-supporting conditions.
Of course, the Mars of today isn’t so hospitable—it’s atmosphere is nearly non-existent, riverbeds are dried up, and any water is now locked under Mars iron oxide-rich soil or CO2-filled ice caps. In other words, it isn’t a great place for humans, so luckily we’ve sent robots in our place to glimpse into our planetary neighbor’s geologic past.
“The Gale lake environment, as revealed by these ancient rocks, gives us a window into a habitable environment that looks surprisingly similar to places on Earth today,” ChemCam’s principal investigator Nina Lanza, said in a press statement . “Manganese minerals are common in the shallow, oxic waters found on lake shores on Earth, and it’s remarkable to find such recognizable features on ancient Mars.”
The piece of tech that’s central to the ChemCam is a high-powered laser that can deliver a dizzying 1 million watts of power into the area the side of a pin-head. While only providing this burst of energy for five-billionths of a second, it’s enough to excite electrons in the soil sample and the spectrometer “reads” the light, detailing the atomic makeup of the sample.
The puzzling thing about manganese is that, at least on Earth the enrichment process is sped up by microbes and oxygen—not exactly modern Mars has in spades.
“On Mars, we don’t have evidence for life, and the mechanism to produce oxygen in Mars’s ancient atmosphere is unclear, so how the manganese oxide was formed and concentrated here is really puzzling,” Los Alamos National Laboratory’s Patrick Gasda, lead author on the study, said in a press statement. “These findings point to larger processes occurring in the Martian atmosphere or surface water and shows that more work needs to be done to understand oxidation on Mars.”
It’s possible that manganese became enriched in these deposits as water percolate through soil adjacent to some ancient river or lake. Because oxidation states of manganese can be used by terrestrial microbes for energy, it’s possible that Martian microbial life similarly fed on these deposits.
Today, Earth stands alone in the Solar System for its remarkable ability to support life, but look back four billion years in the past, and Mars would’ve been the clear favorite for finding life.
Darren lives in Portland, has a cat, and writes/edits about sci-fi and how our world works. You can find his previous stuff at Gizmodo and Paste if you look hard enough.
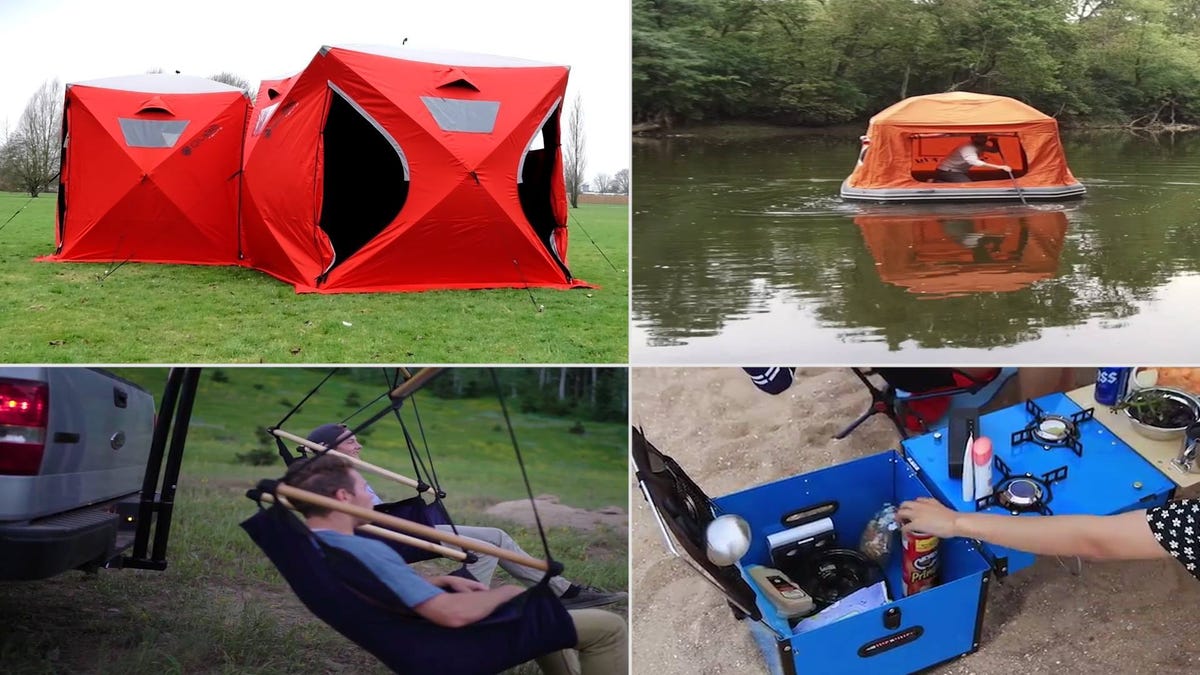
.css-cuqpxl:before{padding-right:0.3125rem;content:'//';display:inline;} Moon and Mars .css-xtujxj:before{padding-left:0.3125rem;content:'//';display:inline;}
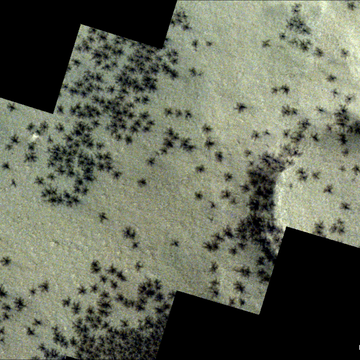
Human-Made Space Debris Could Crash on Mars
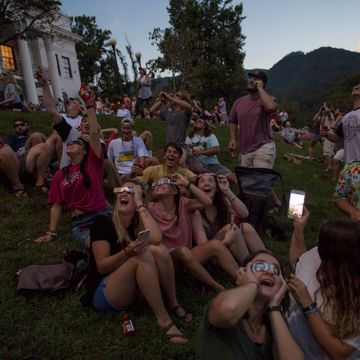
How to View the Solar Eclipse
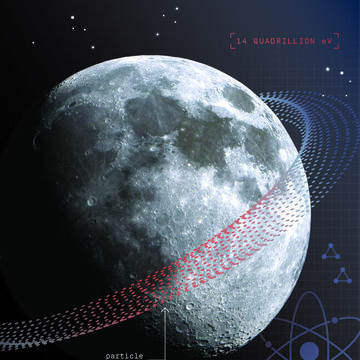
How a Lunar Supercollider Could Upend Physics
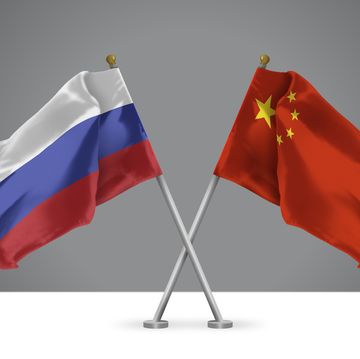
The Moon Is Going Nuclear
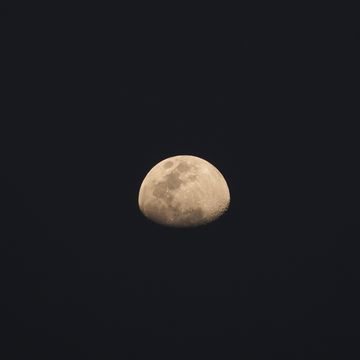
Private Company to Mine Helium-3 from the Moon
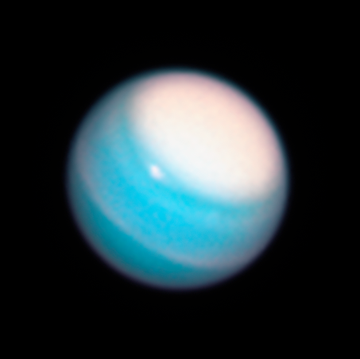
3 Moons Emerged From Around Neptune and Uranus
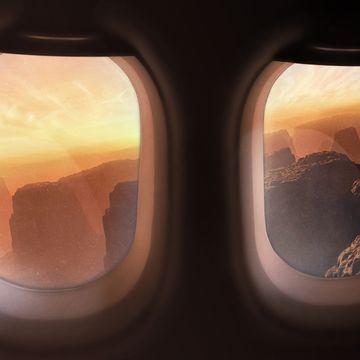
4 People Are Spending a Year In a Martian World.
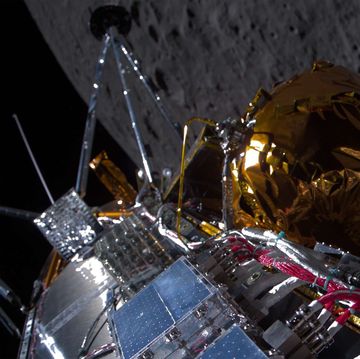
America Has Planted Its Feet on the Moon Once More
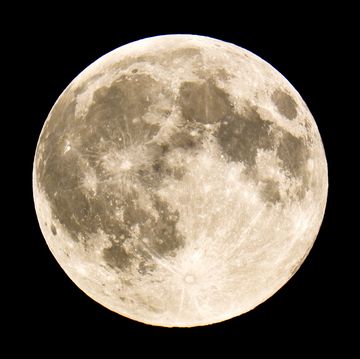
The Strange Origin of the Hollow Moon Conspiracy
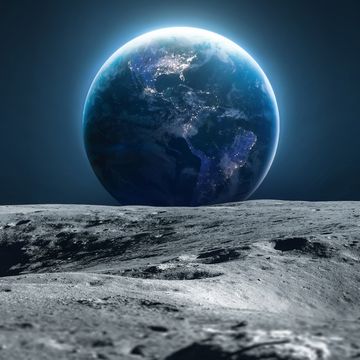
The Moon Is Shrinking Where We're Trying to Land
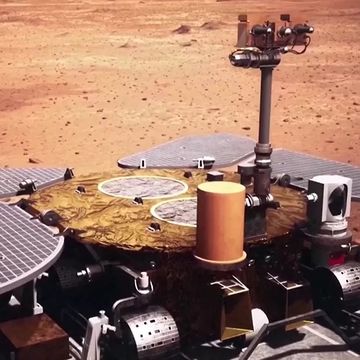
Rover Reveals Bizarre Shapes on Martian Surface
Thank you for visiting nature.com. You are using a browser version with limited support for CSS. To obtain the best experience, we recommend you use a more up to date browser (or turn off compatibility mode in Internet Explorer). In the meantime, to ensure continued support, we are displaying the site without styles and JavaScript.
- View all journals
- Explore content
- About the journal
- Publish with us
- Sign up for alerts
- Published: 06 May 2024
Venus water loss is dominated by HCO + dissociative recombination
- M. S. Chaffin ORCID: orcid.org/0000-0002-1939-4797 1 na1 ,
- E. M. Cangi 1 na1 ,
- B. S. Gregory 1 ,
- R. V. Yelle 2 ,
- J. Deighan ORCID: orcid.org/0000-0003-3667-902X 1 ,
- R. D. Elliott 1 &
- H. Gröller 2
Nature volume 629 , pages 307–310 ( 2024 ) Cite this article
724 Accesses
915 Altmetric
Metrics details
- Atmospheric chemistry
- Inner planets
Despite its Earth-like size and source material 1 , 2 , Venus is extremely dry 3 , 4 , indicating near-total water loss to space by means of hydrogen outflow from an ancient, steam-dominated atmosphere 5 , 6 . Such hydrodynamic escape likely removed most of an initial Earth-like 3-km global equivalent layer (GEL) of water but cannot deplete the atmosphere to the observed 3-cm GEL because it shuts down below about 10–100 m GEL 5 , 7 . To complete Venus water loss, and to produce the observed bulk atmospheric enrichment in deuterium of about 120 times Earth 8 , 9 , nonthermal H escape mechanisms still operating today are required 10 , 11 . Early studies identified these as resonant charge exchange 12 , 13 , 14 , hot oxygen impact 15 , 16 and ion outflow 17 , 18 , establishing a consensus view of H escape 10 , 19 that has since received only minimal updates 20 . Here we show that this consensus omits the most important present-day H loss process, HCO + dissociative recombination. This process nearly doubles the Venus H escape rate and, consequently, doubles the amount of present-day volcanic water outgassing and/or impactor infall required to maintain a steady-state atmospheric water abundance. These higher loss rates resolve long-standing difficulties in simultaneously explaining the measured abundance and isotope ratio of Venusian water 21 , 22 and would enable faster desiccation in the wake of speculative late ocean scenarios 23 . Design limitations prevented past Venus missions from measuring both HCO + and the escaping hydrogen produced by its recombination; future spacecraft measurements are imperative.
This is a preview of subscription content, access via your institution
Access options
Access Nature and 54 other Nature Portfolio journals
Get Nature+, our best-value online-access subscription
24,99 € / 30 days
cancel any time
Subscribe to this journal
Receive 51 print issues and online access
185,98 € per year
only 3,65 € per issue
Buy this article
- Purchase on Springer Link
- Instant access to full article PDF
Prices may be subject to local taxes which are calculated during checkout
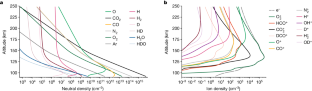
Similar content being viewed by others
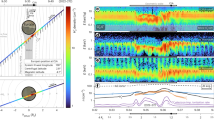
Oxygen production from dissociation of Europa’s water-ice surface
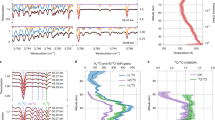
Isotopic fractionation of water and its photolytic products in the atmosphere of Mars
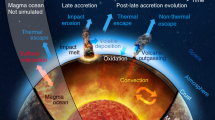
Dry late accretion inferred from Venus’s coupled atmosphere and internal evolution
Data availability.
Tables containing all reactions used in the model, including their adopted rate coefficients and computed column rates, are provided in a supplementary PDF file accessible on the journal website. These rates are also accessible in the archived code repository listed below, which also includes our adopted photo cross-sections and all other source data used in our model. Model densities for all species, computed rates for reactions shown in Fig. 2 , assumed temperature and escape probabilities and computed photo rates are provided in Excel format in the online version of the paper; this file also includes data for our illustrative water-inventory timelines. Source data are provided with this paper.
Code availability
All model code is available at github.com/emcangi/VenusPhotochemistry . The version of the model used to prepare the manuscript is archived on Zenodo at https://doi.org/10.5281/zenodo.10460004 .
Izidoro, A. et al. Planetesimal rings as the cause of the Solar System’s planetary architecture. Nat. Astron. 6 , 357–366 (2022).
Article ADS Google Scholar
Salvador, A. et al. Magma ocean, water, and the early atmosphere of Venus. Space Sci. Rev. 219 , 51 (2023).
Article ADS CAS Google Scholar
Moroz, V. I. et al. Spectrum of the Venus day sky. Nature 284 , 243–244 (1980).
Marcq, E., Mills, F. P., Parkinson, C. D. & Vandaele, A. C. Composition and chemistry of the neutral atmosphere of Venus. Space Sci. Rev. 214 , 10 (2018).
Kasting, J. F. & Pollack, J. B. Loss of water from Venus. I. Hydrodynamic escape of hydrogen. Icarus 53 , 479–508 (1983).
Turbet, M. et al. Day–night cloud asymmetry prevents early oceans on Venus but not on Earth. Nature 598 , 276–280 (2021).
Article ADS CAS PubMed Google Scholar
Johnstone, C. P. Hydrodynamic escape of water vapor atmospheres near very active stars. Astrophys. J. 890 , 79 (2020).
Donahue, T. M., Hoffman, J. H., Hodges, R. R. & Watson, A. J. Venus was wet: a measurement of the ratio of deuterium to hydrogen. Science 216 , 630–633 (1982).
De Bergh, C. et al. Deuterium on Venus: observations from Earth. Science 251 , 547–549 (1991).
Article ADS PubMed Google Scholar
Kumar, S., Hunten, D. M. & Pollack, J. B. Nonthermal escape of hydrogen and deuterium from Venus and implications for loss of water. Icarus 55 , 369–389 (1983).
Donahue, T. M. New analysis of hydrogen and deuterium escape from Venus. Icarus 141 , 226–235 (1999).
Stewart, A. I. F. in Second Arizona Conference on Planetary Atmospheres (1968).
Hodges, R. R. An exospheric perspective of isotopic fractionation of hydrogen on Venus. J. Geophys. Res. Planets 104 , 8463–8471 (1999).
Chaufray, J.-Y., Bertaux, J.-L., Quémerais, E., Villard, E. & Leblanc, F. Hydrogen density in the dayside Venusian exosphere derived from Lyman-α observations by SPICAV on Venus Express. Icarus 217 , 767–778 (2012).
McElroy, M. B., Prather, M. J. & Rodriguez, J. M. Escape of hydrogen from Venus. Science 215 , 1614–1615 (1982).
Gu, H., Cui, J., Niu, D. & Yu, J. Hydrogen and helium escape on Venus via energy transfer from hot oxygen atoms. Mon. Not. R. Astron. Soc. 501 , 2394–2402 (2021).
Hartle, R. E. & Grebowsky, J. M. Light ion flow in the nightside ionosphere of Venus. J. Geophys. Res. Planets 98 , 7437–7445 (1993).
Persson, M. et al. H + /O + escape rate ratio in the Venus magnetotail and its dependence on the solar cycle. Geophys. Res. Lett. 45 , 10805–10811 (2018).
Lammer, H. et al. Loss of hydrogen and oxygen from the upper atmosphere of Venus. Planet. Space Sci. 54 , 1445–1456 (2006).
Gillmann, C. et al. The long-term evolution of the atmosphere of Venus: processes and feedback mechanisms. Space Sci. Rev. 218 , 56 (2022).
Grinspoon, D. H. Implications of the high D/H ratio for the sources of water in Venus’ atmosphere. Nature 363 , 428–431 (1993).
Avice, G. et al. Noble gases and stable isotopes track the origin and early evolution of the Venus atmosphere. Space Sci. Rev. 218 , 60 (2022).
Way, M. J. & Del Genio, A. D. Venusian habitable climate scenarios: modeling Venus through time and applications to slowly rotating Venus-like exoplanets. J. Geophys. Res. Planets 125 , e06276 (2020).
Article Google Scholar
Chaffin, M. S., Deighan, J., Schneider, N. M. & Stewart, A. I. F. Elevated atmospheric escape of atomic hydrogen from Mars induced by high-altitude water. Nat. Geosci. 10 , 174–178 (2017).
Cangi, E. M., Chaffin, M. S. & Deighan, J. Higher Martian atmospheric temperatures at all altitudes increase the D/H fractionation factor and water loss. J. Geophys. Res. Planets 125 , e06626 (2020).
Cangi, E., Chaffin, M., Yelle, R., Gregory, B. & Deighan, J. Fully coupled photochemistry of the deuterated ionosphere of Mars and its effects on escape of H and D. J. Geophys. Res. Planets 128 , e2022JE007713 (2023).
Yung, Y. L. & Demore, W. B. Photochemistry of the stratosphere of Venus: implications for atmospheric evolution. Icarus 51 , 199–247 (1982).
Fox, J. L. & Sung, K. Y. Solar activity variations of the Venus thermosphere/ionosphere. J. Geophys. Res. Space Phys. 106 , 21305–21335 (2001).
Krasnopolsky, V. A. A photochemical model for the Venus atmosphere at 47–112 km. Icarus 218 , 230–246 (2012).
Fedorova, A. et al. HDO and H 2 O vertical distributions and isotopic ratio in the Venus mesosphere by Solar Occultation at Infrared spectrometer on board Venus Express. J. Geophys. Res. Planets 113 , E00B22 (2008).
Paxton, L. J., Anderson Jr, D. E. & Stewart, A. I. F. Analysis of Pioneer Venus Orbiter ultraviolet spectrometer Lyman α data from near the subsolar region. J. Geophys. Res. Space Phys. 93 , 1766–1772 (1988).
Fox, J. L. The post-terminator ionosphere of Venus. Icarus 216 , 625–639 (2011).
Brinton, H. C. et al. Venus nighttime hydrogen bulge. Geophys. Res. Lett. 7 , 865–868 (1980).
Martinez, A. et al. Exploring the variability of the Venusian thermosphere with the IPSL Venus GCM. Icarus 389 , 115272 (2023).
Article CAS Google Scholar
Navarro, T. et al. Venus’ upper atmosphere revealed by a GCM: I. Structure and variability of the circulation. Icarus 366 , 114400 (2021).
Fox, J. L. The chemistry of protonated species in the Martian ionosphere. Icarus 252 , 366–392 (2015).
Taylor, H. A., Brinton, H. C., Wagner, T. C. G., Blackwell, B. H. & Cordier, G. R. Bennett ion mass spectrometers on the Pioneer Venus Bus and Orbiter. IEEE Tran. Geosci. Remote Sens. 18 , 44–49 (1980).
Miller, K. L., Knudsen, W. C. & Spenner, K. The dayside Venus ionosphere: I. Pioneer-Venus retarding potential analyzer experimental observations. Icarus 57 , 386–409 (1984).
Barabash, S. et al. The Analyser of Space Plasmas and Energetic Atoms (ASPERA-4) for the Venus Express mission. Planet. Space Sci. 55 , 1772–1792 (2007).
Bertaux, J. L. & Clarke, J. T. Deuterium content of the Venus atmosphere. Nature 338 , 567–568 (1989).
Donahue, T. M. Deuterium on Venus. Nature 340 , 513–514 (1989).
Liang, M.-C. & Yung, Y. L. Modeling the distribution of H 2 O and HDO in the upper atmosphere of Venus. J. Geophys. Res. Planets 114 , E00B28 (2009).
Parkinson, C. D. et al. Photochemical control of the distribution of Venusian water. Planet. Space Sci. 113 , 226–236 (2015).
Widemann, T. et al. Venus evolution through time: key science questions, selected mission concepts and future investigations. Space Sci. Rev. 219 , 56 (2023).
McClintock, W. E. et al. The Imaging Ultraviolet Spectrograph (IUVS) for the MAVEN Mission. Space Sci. Rev. 195 , 75–124 (2015).
Bertaux, J. L., Goutail, F., Dimarellis, E., Kockarts, G. & van Ransbeeck, E. First optical detection of atomic deuterium in the upper atmosphere from Spacelab 1. Nature 309 , 771–773 (1984).
Gregory, B. S., Elliott, R. D., Deighan, J., Gröller, H. & Chaffin, M. S. HCO + dissociative recombination: a significant driver of nonthermal hydrogen loss at Mars. J. Geophys. Res. Planets 128 , e2022JE007576 (2023).
Barth, C. A., Pearce, J. B., Kelly, K. K., Wallace, L. & Fastie, W. G. Ultraviolet emissions observed near Venus from Mariner V. Science 158 , 1675–1678 (1967).
Anderson, D. E. The Mariner 5 ultraviolet photometer experiment: analysis of hydrogen Lyman alpha data. J. Geophys. Res. 81 , 1213–1216 (1976).
Takacs, P., Broadfoot, A., Smith, G. & Kumar, S. Mariner 10 observations of hydrogen Lyman alpha emission from the Venus exosphere: evidence of complex structure. Planet. Space Sci. 28 , 687–701 (1980).
von Zahn, U., Kumar, S., Niemann, H. & Prinn, R. in Venus (eds Hunten D. M., Colin, L., Donahue, T. M. & Moroz, V. I.) 299–430 (Univ. Arizona Press, 1983).
Hunten, D. M. The escape of light gases from planetary atmospheres. J. Atmos. Sci. 30 , 1481–1494 (1973).
Krissansen-Totton, J., Fortney, J. J. & Nimmo, F. Was Venus ever habitable? Constraints from a coupled interior–atmosphere–redox evolution model. Planet. Sci. J. 2 , 216 (2021).
Warren, A. O. & Kite, E. S. Narrow range of early habitable Venus scenarios permitted by modeling of oxygen loss and radiogenic argon degassing. Proc. Natl Acad. Sci. 120 , e2209751120 (2023).
Article CAS PubMed PubMed Central Google Scholar
Chassefière, E. Hydrodynamic escape of hydrogen from a hot water-rich atmosphere: the case of Venus. J. Geophys. Res. 101 , 26039–26056 (1996).
Chassefière, E. Loss of water on the young Venus: the effect of a strong primitive solar wind. Icarus 126 , 229–232 (1997).
Way, M. J. et al. Was Venus the first habitable world of our solar system? Geophys. Res. Lett. 43 , 8376–8383 (2016).
Article ADS CAS PubMed PubMed Central Google Scholar
Gillmann, C. et al. Dry late accretion inferred from Venus’s coupled atmosphere and internal evolution. Nat. Geosci. 13 , 265–269 (2020).
Selsis, F., Leconte, J., Turbet, M., Chaverot, G. & Bolmont, É. A cool runaway greenhouse without surface magma ocean. Nature 620 , 287–291 (2023).
Fox, J. L. & Bougher, S. W. Structure, luminosity, and dynamics of the Venus thermosphere. Space Sci. Rev. 55 , 357–489 (1991).
Hodges Jr, R. R. Collision cross sections and diffusion parameters for H and D in atomic oxygen. J. Geophys. Res. 98 , 3799–3805 (1993).
Shizgal, B. D. Escape of H and D from Mars and Venus by energization with hot oxygen. J. Geophys. Res. 104 , 14833–14846 (1999).
Yang, J., Boué, G., Fabrycky, D. C. & Abbot, D. S. Strong dependence of the inner edge of the habitable zone on planetary rotation rate. Astrophys. J. 787 , L2 (2014).
Herrick, R. R. & Hensley, S. Surface changes observed on a Venusian volcano during the Magellan mission. Science 379 , 1205–1208 (2023).
Rolf, T. et al. Dynamics and evolution of Venus’ mantle through time. Space Sci. Rev. 218 , 70 (2022).
Hedin, A. E., Niemann, H. B., Kasprzak, W. T. & Seiff, A. Global empirical model of the Venus thermosphere. J. Geophys. Res. 88 , 73–84 (1983).
Bertaux, J.-L. et al. SPICAV on Venus Express: three spectrometers to study the global structure and composition of the Venus atmosphere. Planet. Space Sci. 55 , 1673–1700 (2007).
Hagemann, R., Nief, G. & Roth, E. Absolute isotopic scale for deuterium analysis of natural waters. Absolute D/H ratio for SMOW. Tellus 22 , 712–715 (1970).
ADS CAS Google Scholar
Lillis, R. et al. Photochemical escape of oxygen from Mars: first results from MAVEN in situ data. J. Geophys. Res. Space Phys. 122 , 3815–3836 (2017).
Rosati, R. E., Skrzypkowski, M. P., Johnsen, R. & Golde, M. F. Yield of excited CO molecules from dissociative recombination of HCO + and HOC + ions with electrons. J. Chem. Phys. 126 , 154302–154302 (2007).
Miller, K. L., Knudsen, C. W., Spenner, K., Whitten, R. C. & Novak, V. Solar zenith angle dependence of ionospheric ion and electron temperatures and density on Venus. J. Geophys. Res. Space Phys. 85 , 7759–7764 (1980).
Brace, L. H. et al. The dynamic behavior of the Venus ionosphere in response to solar wind interactions. J. Geophys. Res. 85 , 7663–7678 (1980).
Kasprzak, W. T. et al. in Venus II: Geology, Geophysics, Atmosphere, and Solar Wind Environment (eds Bougher, S. W. et al.) 225–258 (Univ. Arizona Press, 1997).
Niemann, H. B., Kasprzak, W. T., Hedin, A. E., Hunten, D. M. & Spencer, N. W. Mass spectrometric measurements of the neutral gas composition of the thermosphere and exosphere of Venus. J. Geophys. Res. Space Res. 85 , 7817–7827 (1980).
Fox, J. L. & Kliore, A. J. in Venus II: Geology, Geophysics, Atmosphere, and Solar Wind Environment (eds Bougher, S. W. et al.) 161–188 (Univ. Arizona Press, 1997).
Grebowsky, J. M., Kasprzak, W. T., Hartle, R. E. & Donahue, T. M. A new look at Venus’ thermosphere H distribution. Adv. Space Res. 17 , 191–195 (1996).
Donahue, T. M., Grinspoon, D. H., Hartle, R. E. & Hodges, R. R. Jr. in Venus II: Geology, Geophysics, Atmosphere, and Solar Wind Environment (eds Bougher, S. W. et al.) 385–414 (Univ. Arizona Press, 1997).
Stolzenbach, A., Lefèvre, F., Lebonnois, S. & Määttänen, A. Three-dimensional modeling of Venus photochemistry and clouds. Icarus 395 , 115447 (2023).
Dickinson, R. E. & Ridley, E. C. Venus mesosphere and thermosphere temperature structure: II. Day-night variations. Icarus 30 , 163–178 (1977).
Seiff, A. Dynamical implications of the observed thermal contrasts in Venus’ upper atmosphere. Icarus 51 , 574–592 (1982).
Garvin, J. B. et al. Revealing the mysteries of Venus: the DAVINCI mission. Planet. Sci. J. 3 , 117 (2022).
Smrekar, S. E. et al. VERITAS (Venus Emissivity, Radio Science, InSAR, Topography, and Spectroscopy): a selected discovery mission in 53rd Lunar and Planetary Science Conference. LPI contribution no. 2678, id. 1122 (2022).
Helbert, J. et al. The VenSpec suite on the ESA Envision mission – a holistic investigation of the coupled surface atmosphere system of Venus in 16th Europlanet Science Congress, id. EPSC2022-374 (2022).
Download references
Acknowledgements
M.S.C., E.M.C., B.S.G. and R.D.E. were supported by NASA Solar System Workings grant 80NSSC19K0164 and Planetary Science Early Career Award grant 80NSSC20K1081. E.M.C. was also supported by NASA FINESST award 80NSSC22K1326. M.S.C. and E.M.C. thank M. Landis for helpful discussions about water delivery.
Author information
These authors contributed equally: M. S. Chaffin, E. M. Cangi
Authors and Affiliations
Laboratory for Atmospheric and Space Physics, University of Colorado Boulder, Boulder, CO, USA
M. S. Chaffin, E. M. Cangi, B. S. Gregory, J. Deighan & R. D. Elliott
Lunar and Planetary Laboratory, University of Arizona, Tucson, AZ, USA
R. V. Yelle & H. Gröller
You can also search for this author in PubMed Google Scholar
Contributions
M.S.C. oversaw the study, performed final model calculations and the photochemical equilibrium calculation and wrote the initial text of the paper. E.M.C. developed the H-bearing and D-bearing photochemical model and nonthermal escape calculation originally used at Mars with a reaction network provided by R.V.Y. and performed initial model calculations for Venus. B.S.G. developed and ran the Monte Carlo model to generate escape probability curves. R.D.E. initially developed the Monte Carlo escape model with support from J.D. and H.G. H.G. performed pilot studies of HCO + -driven loss in the Mars atmosphere. All authors contributed to the interpretation and presentation of model results.
Corresponding author
Correspondence to M. S. Chaffin .
Ethics declarations
Competing interests.
The authors declare no competing interests.
Peer review
Peer review information.
Nature thanks David Grinspoon and the other, anonymous, reviewer(s) for their contribution to the peer review of this work. Peer reviewer reports are available.
Additional information
Publisher’s note Springer Nature remains neutral with regard to jurisdictional claims in published maps and institutional affiliations.
Extended data figures and tables
Extended data fig. 1 model densities for all species..
The six panels function only to separate species for clarity.
Extended Data Fig. 2 Key photochemical model inputs.
a , Temperature profiles for neutrals, ions and electrons adapted from the inputs in ref. 28 . b , Adopted eddy diffusion profile and molecular diffusion coefficients for H and O atoms.
Extended Data Fig. 3 Implications of HCO + -driven loss for Venus ocean scenarios.
a , Escaping H production rates for the two most important processes in our model. b , Schematic water loss history of Venus.
Supplementary information
Supplementary information.
This file contains Supplementary Methods and Supplementary Tables. Merged PDF containing tables of reactions used in the model, assumed reaction rate coefficients and computed equilibrium model column rates.
Peer Review File
Source data, source data figs. 1–3, rights and permissions.
Springer Nature or its licensor (e.g. a society or other partner) holds exclusive rights to this article under a publishing agreement with the author(s) or other rightsholder(s); author self-archiving of the accepted manuscript version of this article is solely governed by the terms of such publishing agreement and applicable law.
Reprints and permissions
About this article
Cite this article.
Chaffin, M.S., Cangi, E.M., Gregory, B.S. et al. Venus water loss is dominated by HCO + dissociative recombination. Nature 629 , 307–310 (2024). https://doi.org/10.1038/s41586-024-07261-y
Download citation
Received : 03 October 2023
Accepted : 29 February 2024
Published : 06 May 2024
Issue Date : 09 May 2024
DOI : https://doi.org/10.1038/s41586-024-07261-y
Share this article
Anyone you share the following link with will be able to read this content:
Sorry, a shareable link is not currently available for this article.
Provided by the Springer Nature SharedIt content-sharing initiative
By submitting a comment you agree to abide by our Terms and Community Guidelines . If you find something abusive or that does not comply with our terms or guidelines please flag it as inappropriate.
Quick links
- Explore articles by subject
- Guide to authors
- Editorial policies
Sign up for the Nature Briefing newsletter — what matters in science, free to your inbox daily.

- Election 2024
- Entertainment
- Newsletters
- Photography
- Personal Finance
- AP Investigations
- AP Buyline Personal Finance
- AP Buyline Shopping
- Press Releases
- Israel-Hamas War
- Russia-Ukraine War
- Global elections
- Asia Pacific
- Latin America
- Middle East
- Election Results
- Delegate Tracker
- AP & Elections
- Auto Racing
- 2024 Paris Olympic Games
- Movie reviews
- Book reviews
- Personal finance
- Financial Markets
- Business Highlights
- Financial wellness
- Artificial Intelligence
- Social Media
Less alcohol, or none at all, is one path to better health
Moderate drinking was once thought to have benefits for the heart. But better research methods have thrown cold water on that idea. A growing number of public health experts say if you choose to drink alcohol, you should drink as little as possible. (AP Video: Laura Bargfeld)
FILE - Bottles of alcohol sit on shelves at a bar in Houston on June 23, 2020. Moderate drinking was once thought to have benefits for the heart, but better research methods starting in the 2010s have thrown cold water on that. (AP Photo/David J. Phillip, File)
- Copy Link copied
It’s wine time. Beer Thirty. Happy hour. Five o’clock somewhere.
Maybe it’s also time to rethink drinking ?
Moderate drinking was once thought to have benefits for the heart, but better research methods have thrown cold water on that.
“Drinking less is a great way to be healthier,” said Dr. Timothy Naimi, who directs the Canadian Institute for Substance Use Research at the University of Victoria in British Columbia.
AP AUDIO: Less alcohol, or none at all, is one path to better health.
AP correspondent Haya Panjwani reports.
ARE DRINKING GUIDELINES CHANGING?
Guidelines vary a lot from country to country but the overall trend is toward drinking less.
The United Kingdom, France, Denmark, Holland and Australia recently reviewed new evidence and lowered their alcohol consumption recommendations. Ireland will require cancer warning labels on alcohol starting in 2026.
“The scientific consensus has shifted due to the overwhelming evidence linking alcohol to over 200 health conditions, including cancers, cardiovascular diseases and injuries,” said Carina Ferreira-Borges, regional adviser for alcohol at the World Health Organization regional office for Europe.
From Dry January to Sober October to bartenders getting creative with non-alcoholic cocktails , there’s a cultural vibe that supports cutting back.
“People my age are way more accepting of it,” said Tessa Weber, 28, of Austin, Texas. She stopped drinking for Dry January this year because she’d noticed alcohol was increasing her anxiety. She liked the results — better sleep, more energy — and has stuck with it.
“It’s good to reevaluate your relationship with alcohol,” Weber said.
WAIT, MODERATE DRINKING DOESN’T HAVE HEALTH BENEFITS?
That idea came from imperfect studies comparing groups of people by how much they drink. Usually, consumption was measured at one point in time. And none of the studies randomly assigned people to drink or not drink, so they couldn’t prove cause and effect.
People who report drinking moderately tend to have higher levels of education, higher incomes and better access to health care, Naimi said.
“It turns out that when you adjust for those things, the benefits tend to disappear,” he said.
Another problem: Most studies didn’t include younger people. Almost half of the people who die from alcohol-related causes die before the age of 50.
“If you’re studying people who survived into middle age, didn’t quit drinking because of a problem and didn’t become a heavy drinker, that’s a very select group,” Naimi said. “It creates an appearance of a benefit for moderate drinkers that is actually a statistical illusion.”
Other studies challenge the idea that alcohol has benefits. These studies compare people with a gene variant that makes it unpleasant to drink to people without the gene variant. People with the variant tend to drink very little or not at all. One of these studies found people with the gene variant have a lower risk of heart disease — another blow to the idea that alcohol protects people from heart problems.
HOW MANY DRINKS CAN I HAVE PER DAY?
That depends.
Drinking raises the risk of several types of cancer , including colon, liver, breast and mouth and throat. Alcohol breaks down in the body into a substance called acetaldehyde, which can damage your cells and stop them from repairing themselves. That creates the conditions for cancer to grow.
Thousands of U.S. deaths per year could be prevented if people followed the government’s dietary guidelines, which advise men to limit themselves to two drinks or fewer per day and women to one drink or fewer per day, Naimi said.
One drink is the equivalent of about one 12-ounce can of beer, a 5-ounce glass of wine or a shot of liquor.
Naimi served on an advisory committee that wanted to lower the recommendation for men to one drink per day . That advice was considered and rejected when the federal recommendations came out in 2020.
“The simple message that’s best supported by the evidence is that, if you drink, less is better when it comes to health,” Naimi said.
The Associated Press Health and Science Department receives support from the Howard Hughes Medical Institute’s Science and Educational Media Group. The AP is solely responsible for all content.
Suggestions or feedback?
MIT News | Massachusetts Institute of Technology
- Machine learning
- Social justice
- Black holes
- Classes and programs
Departments
- Aeronautics and Astronautics
- Brain and Cognitive Sciences
- Architecture
- Political Science
- Mechanical Engineering
Centers, Labs, & Programs
- Abdul Latif Jameel Poverty Action Lab (J-PAL)
- Picower Institute for Learning and Memory
- Lincoln Laboratory
- School of Architecture + Planning
- School of Engineering
- School of Humanities, Arts, and Social Sciences
- Sloan School of Management
- School of Science
- MIT Schwarzman College of Computing
Two MIT PhD students awarded J-WAFS fellowships for their research on water
Press contact :.
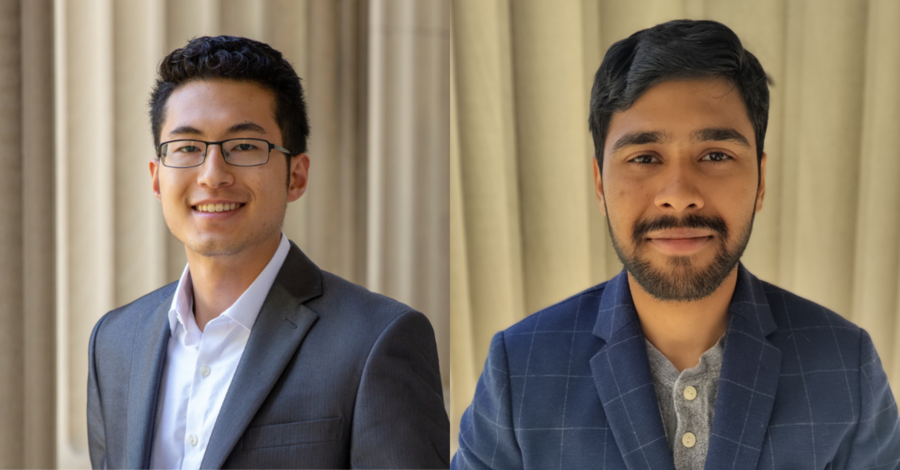
Previous image Next image
Since 2014, the Abdul Latif Jameel Water and Food Systems Lab (J-WAFS) has advanced interdisciplinary research aimed at solving the world's most pressing water and food security challenges to meet human needs. In 2017, J-WAFS established the Rasikbhai L. Meswani Water Solutions Fellowship and the J-WAFS Graduate Student Fellowship. These fellowships provide support to outstanding MIT graduate students who are pursuing research that has the potential to improve water and food systems around the world.
Recently, J-WAFS awarded the 2024-25 fellowships to Jonathan Bessette and Akash Ball, two MIT PhD students dedicated to addressing water scarcity by enhancing desalination and purification processes. This work is of important relevance since the world's freshwater supply has been steadily depleting due to the effects of climate change. In fact, one-third of the global population lacks access to safe drinking water. Bessette and Ball are focused on designing innovative solutions to enhance the resilience and sustainability of global water systems. To support their endeavors, J-WAFS will provide each recipient with funding for one academic semester for continued research and related activities.
“This year, we received many strong fellowship applications,” says J-WAFS executive director Renee J. Robins. “Bessette and Ball both stood out, even in a very competitive pool of candidates. The award of the J-WAFS fellowships to these two students underscores our confidence in their potential to bring transformative solutions to global water challenges.”
2024-25 Rasikbhai L. Meswani Fellowship for Water Solutions
The Rasikbhai L. Meswani Fellowship for Water Solutions is a doctoral fellowship for students pursuing research related to water and water supply at MIT. The fellowship is made possible by Elina and Nikhil Meswani and family.
Jonathan Bessette is a doctoral student in the Global Engineering and Research (GEAR) Center within the Department of Mechanical Engineering at MIT, advised by Professor Amos Winter. His research is focused on water treatment systems for the developing world, mainly desalination, or the process in which salts are removed from water. Currently, Bessette is working on designing and constructing a low-cost, deployable, community-scale desalination system for humanitarian crises.
In arid and semi-arid regions, groundwater often serves as the sole water source, despite its common salinity issues. Many remote and developing areas lack reliable centralized power and water systems, making brackish groundwater desalination a vital, sustainable solution for global water scarcity.
“An overlooked need for desalination is inland groundwater aquifers, rather than in coastal areas,” says Bessette. “This is because much of the population lives far enough from a coast that seawater desalination could never reach them. My work involves designing low-cost, sustainable, renewable-powered desalination technologies for highly constrained situations, such as drinking water for remote communities,” he adds.
To achieve this goal, Bessette developed a batteryless, renewable electrodialysis desalination system. The technology is energy-efficient, conserves water, and is particularly suited for challenging environments, as it is decentralized and sustainable. The system offers significant advantages over the conventional reverse osmosis method, especially in terms of reduced energy consumption for treating brackish water. Highlighting Bessette’s capacity for engineering insight, his advisor noted the “simple and elegant solution” that Bessette and a staff engineer, Shane Pratt, devised that negated the need for the system to have large batteries. Bessette is now focusing on simplifying the system’s architecture to make it more reliable and cost-effective for deployment in remote areas.
Growing up in upstate New York, Bessette completed a bachelor's degree at the State University of New York at Buffalo. As an undergrad, he taught middle and high school students in low-income areas of Buffalo about engineering and sustainability. However, he cited his junior-year travel to India and his experience there measuring water contaminants in rural sites as cementing his dedication to a career addressing food, water, and sanitation challenges. In addition to his doctoral research, his commitment to these goals is further evidenced by another project he is pursuing, funded by a J-WAFS India grant, that uses low-cost, remote sensors to better understand water fetching practices. Bessette is conducting this work with fellow MIT student Gokul Sampath in order to help families in rural India gain access to safe drinking water.
2024-25 J-WAFS Graduate Student Fellowship for Water and Food Solutions
The J-WAFS Graduate Student Fellowship is supported by the J-WAFS Research Affiliate Program , which offers companies the opportunity to engage with MIT on water and food research. Current fellowship support was provided by two J-WAFS Research Affiliates: Xylem , a leading U.S.-based provider of water treatment and infrastructure solutions, and GoAigua , a Spanish company at the forefront of digital transformation in the water industry through innovative solutions.
Akash Ball is a doctoral candidate in the Department of Chemical Engineering, advised by Professor Heather Kulik. His research focuses on the computational discovery of novel functional materials for energy-efficient ion separation membranes with high selectivity. Advanced membranes like these are increasingly needed for applications such as water desalination, battery recycling, and removal of heavy metals from industrial wastewater.
“Climate change, water pollution, and scarce freshwater reserves cause severe water distress for about 4 billion people annually, with 2 billion in India and China’s semiarid regions,” Ball notes. “One potential solution to this global water predicament is the desalination of seawater, since seawater accounts for 97 percent of all water on Earth.”
Although several commercial reverse osmosis membranes are currently available, these membranes suffer several problems, like slow water permeation, permeability-selectivity trade-off, and high fabrication costs. Metal-organic frameworks (MOFs) are porous crystalline materials that are promising candidates for highly selective ion separation with fast water transport due to high surface area, the presence of different pore windows, and the tunability of chemical functionality. In the Kulik lab, Ball is developing a systematic understanding of how MOF chemistry and pore geometry affect water transport and ion rejection rates. By the end of his PhD, Ball plans to identify existing, best-performing MOFs with unparalleled water uptake using machine learning models, propose novel hypothetical MOFs tailored to specific ion separations from water, and discover experimental design rules that enable the synthesis of next-generation membranes.
Ball’s advisor praised the creativity he brings to his research, and his leadership skills that benefit her whole lab. Before coming to MIT, Ball obtained a master’s degree in chemical engineering from the Indian Institute of Technology (IIT) Bombay and a bachelor’s degree in chemical engineering from Jadavpur University in India. During a research internship at IIT Bombay in 2018, he worked on developing a technology for in situ arsenic detection in water. Like Bessette, he noted the impact of this prior research experience on his interest in global water challenges, along with his personal experience growing up in an area in India where access to safe drinking water was not guaranteed.
Share this news article on:
Related links.
- Kulik Research Group
- Abdul Latif Jameel Water and Food Systems Lab (J-WAFS)
- K. Lisa Yang Global Engineering and Research (GEAR) Center
- Department of Chemical Engineering
- Department of Mechanical Engineering
Related Topics
- Awards, honors and fellowships
- Graduate, postdoctoral
- Chemical engineering
- Mechanical engineering
- Desalination
- Climate change
- Sustainability
- Environment
- International development
- Computer modeling
Related Articles
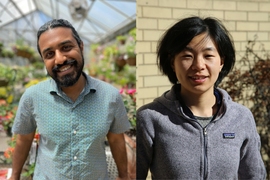
MIT PhD students honored for their work to solve critical issues in water and food
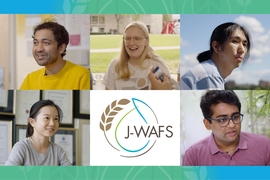
MIT PhD students shed light on important water and food research
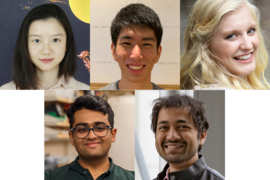
Five MIT PhD students awarded 2022 J-WAFS fellowships for water and food solutions
Previous item Next item
More MIT News
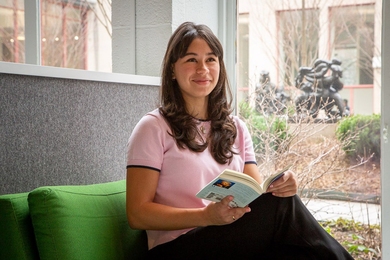
Discovering community and cultural connections
Read full story →
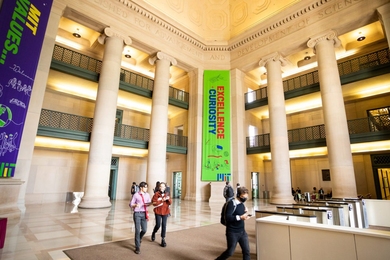
MIT Supply Chain Management Program earns top honors in three 2024 rankings
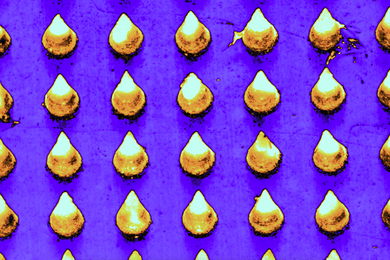
New treatment could reverse hair loss caused by an autoimmune skin disease
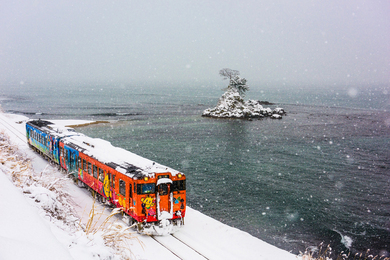
Study: Heavy snowfall and rain may contribute to some earthquakes
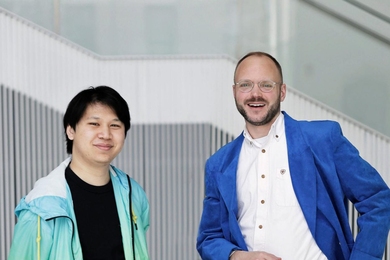
How AI might shape LGBTQIA+ advocacy
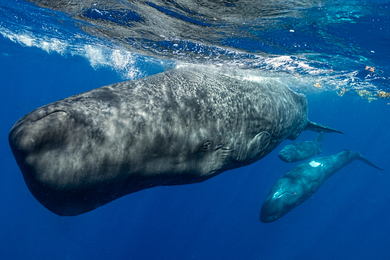
Exploring the mysterious alphabet of sperm whales
- More news on MIT News homepage →
Massachusetts Institute of Technology 77 Massachusetts Avenue, Cambridge, MA, USA
- Map (opens in new window)
- Events (opens in new window)
- People (opens in new window)
- Careers (opens in new window)
- Accessibility
- Social Media Hub
- MIT on Facebook
- MIT on YouTube
- MIT on Instagram
Opinion | J.B. Pritzker, et al.: Clean water is our…
Share this:.
- Click to share on Facebook (Opens in new window)
- Click to share on X (Opens in new window)
- Click to print (Opens in new window)
- Click to email a link to a friend (Opens in new window)
- Clarence Page
Opinion | J.B. Pritzker, et al.: Clean water is our moonshot moment for Midwest climate leadership

One-fifth of the planet’s surface freshwater sits in our Great Lakes. Demand for it will only grow, which gives us both an opportunity and a responsibility to speed the pace of water innovation. We must manage and conserve our finite fresh water as if it were a sea of diamonds.
Even our “waste” water is precious. Over the next 10 years, a bipartisan coalition called Great Lakes ReNEW will invest millions of dollars in new technologies to recover and recycle valuable minerals, such as nickel, cobalt and lithium, from our water, and remove toxic chemicals such as per- and polyfluorinated substances, known as PFAS. The goal is to destroy what’s toxic and reuse what’s valuable.
Led by Chicago-based nonprofit Current, which Alaina Harkness leads, scientists and entrepreneurs will join forces to ensure that this Great Lakes water innovation hub creates economic opportunity for all.
We do not have time to waste. Already, we waste too much of our water and the valuable materials it carries — materials we need to make batteries, electrify society and power the next wave of computing. Too much of our water is contaminated.
The first challenge is precision separation: figuring out how to get both the bad and the valuable out, en masse. The second challenge: doing it with less energy than current technology allows. We have to invent solutions that won’t contribute to the warming of our planet.
And then, the third challenge is perhaps the hardest: getting industries and governments to adopt it. And the truth is, for that to happen, it has to be affordable or proved to save companies money or be publicly subsidized with tax incentives, similar to the way electric vehicle purchases have been subsidized.
Currently, the majority of these materials are obtained from foreign nations, introducing risks to our economic security.
Similarly, extracting nutrients such as phosphates and nitrates from agricultural runoff not only stops ocean-killing algae blooms in the Gulf of Mexico but also contributes to a circular economy for fertilizers. (Did you know that most fertilizer comes from phosphate rock, a finite resource?)
While this sounds like science fiction, it is not.
Breakthrough research at Northwestern University is making progress on PFAS removal, with a prototype absorption column already in use at the Metropolitan Water Reclamation District of Greater Chicago ’s O’Brien facility in Skokie.
At scale, this technology will be able to selectively separate these dangerous chemicals, which cause long-term adverse health effects, from our precious fresh water. Even better, this technology one day could be installed upstream at the source of the industrial pollution, where minerals and contaminants exist in higher concentrations and thus are easier to detect and capture.
ReNEW will also be helping nascent startups, such as Rapid Radicals, a spinout of Marquette University in Wisconsin, scale its wastewater separation technology. And in Ohio, we’ll be leveraging an industry-leading testbed in Lake Erie run by the Cleveland Water Alliance.
Achieving meaningful, measurable progress on these ambitious goals will require state leaders like us and our successors to collaborate. Protecting our water must be a consensus priority.
We like to say: Built in the Great Lakes, made for the world.
For all of human history, we’ve turned water into wealth. Turning waste into wealth is far more difficult. In this case, it’s our moonshot moment for the Great Lakes, and for climate leadership in the Midwest.
J.B. Pritzker is the governor of Illinois, Tony Evers is the governor of Wisconsin and Alaina Harkness is CEO of Current.
Submit a letter, of no more than 400 words, to the editor here or email [email protected] .
More in Opinion

Editorials | Editorial: Gambling on elections? Biden or Trump is a bet too far.

Opinion | Michael Schill: Here’s why I reached an agreement with Northwestern protesters

Opinion | Zaher Sahloul: President Joe Biden, bring a full stop to the catastrophic offensive on Rafah

Opinion | Micky Horstman: The key to CTA’s rebound is boosting business, not taxes
Trending nationally.
- New COVID ‘FLiRT’ variants are spreading nationwide. Chicago health experts urge up to date vaccination.
- Animation showing planned Key Bridge explosive demolition unveiled
- Steve Albini, legendary Chicago music producer for Nirvana and the Pixies, dies at 61
- After 114 years, Boy Scouts of America is changing name to Scouting America
- Three ‘Bernedoodles’ rescued from hot vehicle near Disney, adopted by firefighters
- Share full article
Advertisement
Supported by
Why You Can Hear the Temperature of Water
A science video maker in China couldn’t find a good explanation for why hot and cold water sound different, so he did his own research and published it.

By Sam Kean
Most people are quite good at distinguishing between the sound of a hot liquid and the sound of a cold one being poured, even if they don’t realize it.
“Every time I give a talk and I say, ‘Surprisingly, adults can tell the difference between hot and cold water,’ people just go like this,” said Tanushree Agrawal, a psychologist who, during a video call, mimicked audience members shaking their heads no. But research she completed at the University of California at San Diego demonstrated that three-fourths of the participants in her experiments could in fact detect the difference.
You can try it yourself. Put on your headphones or listen closely to your computer or phone’s speaker and hit play on this audio recording.
Can You Hear the Temperature?
Could you tell which sound was hot and which was cold?
If you said the first one was cold, congratulations: You’re in Dr. Agrawal’s majority.
In general, cold water sounds brighter and splashier, while hot water sounds duller and frothier. But until recently no one really had evidence to explain the difference.
However, Xiaotian Bi, who earned a Ph.D. in chemical engineering last year from Tsinghua University in Beijing, offers a new explanation in a paper he and colleagues published in March on the arXiv website. It’s all about the size of the bubbles that form during pouring, he says, and this insight may have implications for how we enjoy everyday food and drink.
Dr. Bi’s paper has not yet been through peer review, and he acknowledges that much more research is needed. But Joshua Reiss, a professor of audio engineering at Queen Mary University of London, who has also studied the acoustics of hot and cold water, said he was “on the right track, for sure.”
Discussions of the varying sounds of hot and cold liquids usually point to differences in viscosity as the culprit. But Dr. Bi wasn’t satisfied with that reasoning. He produces and stars in his own popular science videos , and decided that the sounds water makes at different temperatures was a good topic . He poked around looking for published research on the subject and came away disappointed.
“None of them gave a precise explanation,” he said, adding that it was “an unsolved mystery.”
So Dr. Bi decided to do his own scientific investigation, which would inform his video. He used his expertise in fluid dynamics to explore the role played by bubbles, which actually create most of the sound we hear in moving water. You can observe this in waves, which glide along silently until they break, at which point they fall and trap air that produces noise as the bubbles resonate briefly within the water.
Previous research showed that larger air bubbles in liquids produce lower-frequency sounds. Dr. Bi also found that the acoustical spectrum of hot water has more low-frequency sounds than the spectrum of cold water. He wondered, then, whether pouring hot water into a container would trap larger bubbles than pouring cold would, and whether that might explain the difference in sounds.
His hunch proved correct. Dr. Bi purchased a container with a spigot to dispense water in a controlled fashion, first at 50 degrees Fahrenheit, then at 194 degrees. High-resolution videos and photographs revealed that hot water consistently produced bubbles 5 to 10 millimeters in size, while cold water produced bubbles around 1 to 2 millimeters.
(That’s why the cold water is on the left side of your screen in video above, and the hot water on the right)
In addition to offering an explanation of something that people hear, the research also provides insight into how we enjoy food and drink in general. Consider coffee.
Coffee tastes delicious when hot, but gunky and bitter when cold. That’s because aromatic flavor molecules jump off the surface of hot beverages more readily. And that link between flavor and temperature can produce a Pavlovian response in coffee drinkers.
This is consistent with an observation by Charles Spence, a psychologist who heads the Crossmodal Research Laboratory at Oxford and has won an Ig-Nobel Prize for research on the links between sound and taste when potato chips are consumed. In a 2021 paper, he wrote that “the sound of temperature likely helps to subtly set people’s aromatic flavor expectations,” even if unconsciously.
“Very often we taste what we predict,” he said. It’s all part of what he calls the hidden “sonic seasoning” of food and drinks.

IMAGES
VIDEO
COMMENTS
The tiny animals nicknamed "water bears" can endure extreme conditions by entering a deep hibernation with a switch at the molecular level, a new study finds ... The research could one day inform ...
Tardigrades, or water bears, thrive in some of Earth's harshest environments. Now, researchers say they have unlocked the survival mechanism of the tiny creature.
New research finds that the microscopic "water bears" are remarkably good at repairing their DNA after a huge blast of radiation. By Carl Zimmer To introduce her children to the hidden marvels ...
These microscopic organisms, also known as water bears, boast a unique and sophisticated body plan. Discover the complexities of their body segments, each equipped with claws and specialized structures. Tardigrades exhibit a tubular stylet used for feeding and a distinctive cuticle that shields them from environmental challenges.
Tardigrades are microscopic eight-legged animals that have been to outer space and would likely survive the apocalypse. Bonus: They look like adorable miniature bears. Around 1,300 species of ...
New Research. Water Bears Are the Master DNA Thieves of the Animal World. Foreign genes from bacteria, fungi and plants may have bestowed these animals with their ability to tolerate boiling ...
Researchers found the bears could withstand an impact of up to 900 meters per second and shock pressures of up to 1.14 gigapascals. At higher speeds, the seemingly invincible water bears turned to ...
Confronted with drying, rapid temperature changes, changes in water salinity or other problems, tardigrades can curtail their metabolism to 0.01 percent of normal, entering a kind of suspended ...
NASA Is Blasting Water Bears, Bobtail Squid Into Space For Scientific Research The animals are being launched into the cosmos as NASA researchers attempt to learn more about how the conditions of ...
Water bears live in temporary ponds and droplets of water in soil and on moist plants. They are known to survive under conditions that would kill most organisms - they can withstand temperatures ranging from -272 deg C to +150 deg C, they can be without water for a period of 10 years, and they are extremely resistant to radiation.
But the Cell Science-04 water bears aren't the first tardigrades to visit space. In 2007, a European research team sent 3,000 living tardigrades into Earth orbit for 12 days on the outside of a ...
University of Tokyo. Summary: Some species of tardigrades, or water bears as the tiny aquatic creatures are also known, can survive in different environments often hostile or even fatal to most ...
Figure 2 - (A) Water bears can survive in extremely cold habitats, like the icy Himalaya mountains, and at temperatures as low as −150°C. (B) Water bears can survive in extremely hot habitats, like the hottest deserts, and at temperatures as high as 100°C. (C) Water bears can even survive in the vacuum of space!
The water bear's resilience unveils a microcosmic marvel, inviting us to explore the extraordinary mechanisms that enable them to triumph in the face of adversity. Cryptobiosis Mastery. Tardigrades, the microscopic marvels often referred to as water bears, wield an extraordinary survival strategy - cryptobiosis.
Welcome to the official podcast of the NASA Johnson Space Center, Episode197, "Water Bears in Space.". I'm Gary Jordan and I'll be your host today. On this podcast we bring in the experts, scientists, engineers, and astronauts, all to let you know, what's going on in the world of human spaceflight. Water bears are about to head to the ...
The name "water-bear" comes from the way they walk, reminiscent of a bear's gait. The name Tardigradum means "slow walker" and was given by Lazzaro Spallanzani in 1777. ... Courtship is an early step in mating and was first observed in tardigrades in 1895. Research shows that up to nine males aggregate around a female to mate.
Water bears are resistant to physical stressors as well as some chemical stressors such as hydrogen sulphide, carbon dioxide, ethanol (for ca. 10 min) and 1-hexanol (Baumann 1922; Ramlov and Westh 2001). ... The last space research project involving tardigrades was the Phobos Life Project. It was a part of the Phobos Ground Mission.
Tardigrades are short (0.05mm - 1.2mm in body length), plump, bilaterally symmetrical, segmented organisms. They have four pairs of legs, each of which ends in four to eight claws. Tardigrades reproduce via asexual ( parthenogenesis) or sexual reproduction and feed on the fluids of plant cells, animal cells, and bacteria.
1. Introduction. Recent climate changes and increasing warming of polar and high mountain ecosystems are the main threats to psychrophiles. Consequently, due to a rapid change in environmental conditions, these organisms might completely disappear or hide in cold refugia (Fountain et al. 2012; Milner et al. 2017; Zawierucha & Shain 2019).Considering that the world is being subjected to the so ...
The world's most indestructible species, the tardigrade, an eight-legged micro-animal, also known as the water bear, will survive until the Sun dies, according to a new Harvard-Smithsonian Center for Astrophysics and Oxford University collaboration. ... The research implies that life on Earth in general, will extend as long as the Sun keeps ...
Editors: Ralph O. Schill. Exhaustively reviews virtually every conceivable aspect of tardigrade biology. Includes field and laboratory methods for studying water bears. Provides a timely summary on the discovery and history of these animals. Part of the book series: Zoological Monographs (ZM, volume 2) 32k Accesses. 223 Citations.
Related Papers. Tardigrades popularly known as water bears are micrometazoans with four pairs of lobopod legs. They are the organisms which can live in extreme conditions and are known to survive in vacuum and space without protection. Tardigardes survive in lichens and mosses, usually associated with water film on mosses, liverworts, and lichens.
Water May Have Flowed on Mars 400,000 Years Ago This week a new study, published in the Journal of Geophysical Research: Planets , finds more compelling evidence of Mars' Earth-like beginnings.
The No. 3 California women's water polo team is set to host the NCAA Championship for the first time in program history this week at Spieker Aquatics Complex. The Bears have the No. 3 seed for the second straight year and will face No. 6 Fresno State (21-7) in the quarterfinal round on Friday at 6 p.m. PT.
Despite its Earth-like size and source material1,2, Venus is extremely dry3,4, indicating near-total water loss to space by means of hydrogen outflow from an ancient, steam-dominated atmosphere5,6.
Moderate drinking was once thought to have benefits for the heart, but better research methods have thrown cold water on that. "Drinking less is a great way to be healthier," said Dr. Timothy Naimi, who directs the Canadian Institute for Substance Use Research at the University of Victoria in British Columbia.
Since 2014, the Abdul Latif Jameel Water and Food Systems Lab (J-WAFS) has advanced interdisciplinary research aimed at solving the world's most pressing water and food security challenges to meet human needs. In 2017, J-WAFS established the Rasikbhai L. Meswani Water Solutions Fellowship and the J-WAFS Graduate Student Fellowship.
Breakthrough research at Northwestern University is making progress on PFAS removal, with a prototype absorption column already in use at the Metropolitan Water Reclamation District of Greater ...
High-resolution videos and photographs revealed that hot water consistently produced bubbles 5 to 10 millimeters in size, while cold water produced bubbles around 1 to 2 millimeters.
Earth's magnetic field was once 30 times weaker than it is today. This change may have played a pivotal role in the blossoming of complex life, new research found.