
An official website of the United States government
The .gov means it’s official. Federal government websites often end in .gov or .mil. Before sharing sensitive information, make sure you’re on a federal government site.
The site is secure. The https:// ensures that you are connecting to the official website and that any information you provide is encrypted and transmitted securely.
- Publications
- Account settings
Preview improvements coming to the PMC website in October 2024. Learn More or Try it out now .
- Advanced Search
- Journal List
- Food Technol Biotechnol
- v.55(1); 2017 Mar

General Characteristics and Treatment Possibilities of Dairy Wastewater – A Review
The milk processing industry is one of the world’s staple industries, thus the treatment possibilities of dairy effluents have been attracting more and more attention. The purpose of the paper is to review contemporary research on dairy wastewater. The origin, categories, as well as liquid by-products and general indicators of real dairy wastewater are described. Different procedures applied for dairy wastewater management are summarised. Attention is focused on in-factory treatment technologies with the emphasis on biological processes. Aerobic and anaerobic methods with both their advantages and disadvantages are discussed in detail. Consecutive anaerobic and aerobic systems are analysed, too. Finally, future research niches are identified.
Introduction
The dairy industry includes the transformation of raw milk into pasteurised and sour milk, yoghurt, hard, soft and cottage cheese, cream and butter products, ice cream, milk and whey powders, lactose, condensed milk, as well as various types of desserts ( 1 – 6 ). The general distinctions among these foods are due to the reuse of non- -fat milk and whey (a by-product in cheese manufacturing) and the evaporation of the free water from the coagulum as well as from milk and whey powders ( 5 ). With the rapid industrialisation observed in the last century ( 4 ) and the growing rate of milk production (around 2.8% per annum), dairy processing is usually considered the largest industrial food wastewater source, especially in Europe ( 1 – 3 , 7 ). Moreover, in around 50% of the world’s whey production, especially concerning acid whey, it is untreated prior to disposal ( 8 – 10 ). The effluents originating from various production technologies are not discharged simultaneously, thus forming a stream with wide qualitative and quantitative variations ( 4 ). Notwithstanding the differences in composition, attributable to the manufactured product and technological operations ( 11 , 12 ), dairy effluents are distinguished by their relatively increased temperature, high organic content ( 13 – 15 ) and a wide pH range, which requires special purification in order to eliminate or reduce environmental damage ( 1 ). Treatments of dairy wastewaters include the application of mechanical, physicochemical and biological methods. Mechanical treatment is necessary to equalise volumetric and mass flow changes. It also reduces parts of the suspended solids. Physicochemical processes are effective in the removal of emulsified compounds, but reagent addition increases water treatment costs. Another disadvantage is the very low elimination of soluble chemical oxygen demand (COD). Therefore, biological wastewater treatment systems are preferred due to the highly biodegradable contaminants ( 7 , 11 , 16 , 17 ).
The purpose of the paper is to review the data on the basic composition and treatment possibilities of milk- -processing effluents. Their origin and major characteristics are summarised. Various methods for wastewater utilisation are discussed. Finally, suggestions for future research are made.
Dairy Wastewater Characteristics
Wastewater volume.
Water plays a key role in milk processing. It is used in every step of the technological lines, including cleaning and washing, disinfection, heating and cooling. Water requirements are huge ( 14 ).
The bulk of wastewater comes from manufacturing processes ( 6 ). Contaminated water, including sanitary activities, reaches 50–80% of the total water consumed in the dairy factory, whereas the remaining 20–50% is conditionally clean ( 6 , 18 ). It has been estimated that the amount of wastewater is approx. 2.5 times higher than that of processed milk in units of volume. The amount and characteristics of the wastewater depend largely on the factory size, applied technology, effectiveness and complexity of clean-in-place (CIP) methods, good manufacture practices (GMP), etc . ( 3 , 4 ). However, the introduction of GMP can reduce the world’s wastewater mean volume from 0.5–37 to 0.5–2 m 3 of effluent per m 3 of processed milk ( 3 , 19 ). Nowadays, the designed volumetric load is 1 m 3 of effluent per tonne of manufactured milk ( 4 ).
In dairy plants, the great fluctuations in wastewater quality and quantity are very problematic because each milk product needs a separate technological line ( 1 , 5 ). This results in the change of dairy effluent composition with the start of a new cycle in the manufacturing process, which impedes the work of in-factory wastewater treatment plants. Furthermore, intensive effluent volumetric variations in time are commonly observed. Daily and hourly changes are the consequence of washing the equipment and floors as the final step in every process cycle. Seasonal variations can be attributed to a higher dairy plant load in summer than in winter ( 20 ). One way of explaining hourly homogeneity is by coefficients in the range of 1.4–2.0 ( 6 ). The diurnal inequality coefficient depends on the seasonal character of dairy processing, varying from 1.5 for 2- and 3-shift work in summer to 2.6 for winter shifts. The actual concentration of polluting dairy effluents varies widely depending on the profile and capacity of the company, the production technology, the type of equipment used, the degree of wastewater reuse, the loss of raw materials, waste management, etc . ( 1 , 18 ). A major factor in the volumetric loading of dairy wastewater treatment plants are the immediate discharges produced in the cleaning of tank trucks, pipelines or equipment at the end of each cycle. In such cases, the effluent volumes are higher than those of manufactured milk ( 4 , 21 ). On average, wastewater discharge is 70% of the amount of the fresh water used at the plant ( 6 ).
Dairy processing effluents mostly include milk or milk products lost in the technological cycles (spilled milk, spoiled milk, skimmed milk and curd pieces); starter cultures used in manufacturing; by-products of processing operations (whey, milk and whey permeates); contaminants from the washing of milk trucks, tanks, cans, equipment, bottles and floors; reagents applied in CIP procedures, cooling of milk and milk products, for sanitary needs, in equipment damage or operational problems; and various additives introduced in manufacturing ( 13 , 18 , 22 , 23 ). Milk loss in wastewater is around 0.5–2.5% of processed milk, but it can increase to 3–4% ( 20 ).
Wastewater categories
The wide variety of dairy products presupposes the existence of many wastewater types. However, three major categories can be outlined according to their origin and composition ( 1 ), explained in the following chapters.
Processing water
Processing water is formed in the cooling of milk in special coolers and condensers, as well as condensates from the evaporation of milk or whey. Milk and whey drying produces vapours which form the cleanest effluent after condensation although it may contain volatile substances as well as milk or whey droplets from evaporators ( 6 ). In general, processing waters lack pollutants and, after minimal pretreatment, they can be reused or discharged together with stormwater ( 1 ). Water reusage is possible for installations that are not in direct contact with derived products. Typical applications include hot water and steam production as well as membrane cleaning. The water from the cooling of products during pasteurisation after the last rinse of bottles and condensates generated in vacuum installations from secondary vapours can be utilised for room cleaning, lawn irrigation, etc . ( 6 , 18 ).
Cleaning wastewater
Cleaning wastewater usually comes from washing equipment which is in direct contact with milk or dairy products. It also includes milk and product spillage, whey, pressing and brine, CIP effluents or equipment malfunction and even operational errors. Over 90% of organic solids in effluents come from milk and manufacturing residues: cheese pieces, whey, cream, water from separation and clarification, starter cultures, yoghurt, fruit concentrates or stabilisers. These effluents are in large quantities and are highly polluted, thus requiring further treatment.
Sanitary wastewater
Sanitary wastewater is found in lavatories, shower rooms, etc . Sanitary wastewater is similar in composition to municipal wastewater and is generally piped directly to sewage works ( 1 , 6 , 21 ). It can be used as nitrogen source for unbalanced dairy effluents before a secondary aerobic treatment ( 1 , 12 ).
Additionally, the by-products of manufacturing processes, such as whey, milk and whey permeates, can also be grouped separately if they are collected individually from other wastewater streams ( 12 , 24 , 25 ).
The main pollutant in milk processing wastewater is whey due to its high organic and volumetric load. It represents about 85–95% of the milk volume and 55% of the milk components. Whey consists of carbohydrates (4–5%), mostly lactose. Proteins and lactic acid amount to less than 1%, fats to around 0.4–0.5%, while salts vary from 1 to 3% ( 2 , 15 , 26 ). Whey is produced mainly in cheese manufacturing, and its volume depends on the productivity of cheese and the type of processed milk – bovine, goat, sheep, etc . ( 2 , 9 , 27 ). On the basis of milk casein coagulation procedures, whey can be categorised as cheese whey and second cheese whey. Cheese whey is a by-product in the production of hard, semi-hard and soft cheese, after the addition of rennin to milk. Mild enzyme action produces sweet whey with a pH=6–7 ( 2 , 28 ). Second cheese whey is a by-product in cottage cheese production after milk has been fermented, or curdled, with organic or mineral acids. Due to strong acid conditions, whey develops an acidic taste, while the average pH value rarely exceeds 5. Scientific literature also discusses casein whey whose composition is very close to that of second cheese whey ( 2 ). Sweet and acid whey also differ in mineral and protein content ( 9 , 29 ).
During cheese manufacturing, cheese whey wastewater is produced as well. Its volume and composition change with respect to the type of produced cheese, the applied technology, the milk type and the environment. Cheese whey wastewater originates in the addition of surplus cheese whey and second cheese whey to washing effluents. Nevertheless, its contamination level is lower than that of cheese whey ( 2 , 30 ).
Cheese whey waste streams are valuable sources of different compounds (protein, lactose, mineral elements) and are utilised in the manufacture of various products, such as lactic acid, single-cell protein, baker’s yeast, starter cultures, fermented whey drinks, enzymes, antibiotics, organic acids, vitamins, food gums, etc . Nevertheless, it should be taken into account that whey or whey product recovery results in new waste streams which also need to be treated although such effluents are less polluted than whey and their organic loading is comparable to other dairy wastewater ( 4 , 9 , 31 , 32 ).
Milk and whey permeates are by-products in cheese manufacturing; they are produced during milk and whey ultrafiltration, respectively. Their solid content is lower; they are rich in soluble compounds, over 80% of which is lactose ( 24 , 25 , 33 ).
Dairy wastewater consists of complex constituents ( 11 , 34 ). Knowing the composition of milk and milk products, we can estimate better the wastewater contaminant loading ( Table 1 ) ( 22 ). Although milk manufacturing produces waste streams analogous to milk and dairy product loss, every process gives an effluent unique in volume and composition ( 4 ).
COD=chemical oxygen demand, BOD u =ultimate biological oxygen demand
Dairy wastewater volumetric and flow rates (depending on the production capacity and work shifts), as well as pH and total suspended solids (TSS) content (as a consequence of applied CIP methods) affect the efficiency of wastewater treatment management ( 1 ). It is important to know the quantity of the milk to be pasteurised, how much milk is processed into cheese and whether the entire obtained whey is discharged in wastewater or part of it is processed and reused ( 6 ). Contaminant concentrations in wastewater can be determined by using Eq. 1:

where C is contaminant concentration in wastewater (g/m 3 ), L is the loss of milk and milk products in different technological production cycles expressed in proportional units (m 3 or t), C 1 , C 2 and C n are contaminant concentration per unit of milk or milk product loss (g/t), and N 1 , N 2 and N n are wastewater discharge per unit of milk or generated milk products (m 3 /t) ( 18 ).
Dairy wastewater composition
Milk processing effluents have an increased temperature and large variations in pH, TSS, biological oxygen demand (BOD), COD, total nitrogen (TN), total phosphorus (TP) and fat, oil and grease (FOG) ( 1 , 3 , 7 , 13 , 15 , 35 ). There is little information on industrial-scale dairy effluent composition ( 1 , 11 ). The information on the general characteristics of dairy wastewater is shown in Table 2 .
BOD 5 =biological oxygen demand for 5 days, COD=chemical oxygen demand, FOG=fat, oil and grease, TS=total solids, TSS=total suspended solids, TN=total nitrogen, TP=total phosphorus
Typically, dairy wastewater is white in colour (whey is yellowish-green) and has an unpleasant odour and turbid character ( 2 , 12 , 36 ).
With annual temperatures of 17–25 °C, dairy waste streams are warmer than municipal wastewater (10–20 °C), which results in faster biological degradation compared to sewage treatment plants ( 37 ). The average temperatures of industrial dairy effluents range from 17–18 °C in winter and 22–25 °C in summer ( 6 ). Using the Arrhenius equation, the biodegradation rates and oxygen consumption can be predicted to be 1.5 times higher in summer than in winter ( 37 ). The design winter temperature of 15 °C is adopted for this type of wastewater due to the utilisation of hot water for washing and cleaning of equipment ( 6 , 18 ).
A crucial requirement for biological treatment of dairy wastewater is their pH value between 6 and 9 ( 37 ). Milk and butter factories have effluents with active reaction close to neutral (pH=6.8–7.4). In plants where a certain amount of whey is discharged, the pH of the effluent is reduced to below 6.2. In cheese manufacturing, sweet whey is slightly acidic, with pH=5.9–6.6, while mineral acid coagulation gives an acidic whey with pH=4.3–4.6 ( 6 , 27 ). The sharp increase in the short-term pH of the total flow of up to 10–10.5 is attributable to the discharge of alkaline cleaning solutions. The prolonged exposure of wastewater to anaerobic conditions (in the sewer network with sumps) causes liquid acidification by lactic acid fermentation that leads to a decrease in pH ( 18 ).
Although dairy wastewaters have low concentrations of settleable solids, they may clog sewage pipes. Most of the suspension enters the initial stage of equipment cleaning. The bulk of the sediment (90%) of organic matter is usually of protein origin, namely particles of solid milk processing (pieces of cheese, coagulated milk, cheese, curd fines, milk film or flavouring agents, etc .) and other impurities (soil or sand) that get into the sewage system during equipment washing or packaging ( 12 , 18 ). Formation of protein and fat deposits on the inside of the pipes requires periodic cleaning with appropriate chemical or bacterial preparations. The main advantage in the application of such bacteria is that they continue acting in the next stages of wastewater treatment, increasing the purification effect ( 38 ). The highest amount of total solids (TS) has been reported in whey, with negligible amount of volatiles ( 3 ). Fats in dairy industry effluents are found in trace amounts in the form of emulsions with a droplet diameter of 1–10 µm ( 6 ). During homogenisation, the size of milk fat globules is reduced to 1–2 µm. The obtained stable emulsion, when passing into dairy effluents, affects the mechanical wastewater treatment system due to its difficult separation ( 4 ). Thus, fats remaining in cheese whey wastewater can produce an undesired flotation, which results in the washout of active sludge during biological processes ( 2 ). In the production of high-fat products (cream, sour cream and butter), larger fat globules are extracted from the milk, due to their coalescence and enlargement, as well as the degradation of the protein shell. That is why fat impurities in the wastewater from these productions are significantly different in type and concentration and their elimination by settling is more efficient than in other dairy effluents. The FOG concentration in the wastewater from dairy plants specialised in the production of high-fat products is 0.2–0.4 g/L although higher values (up to 2.88 g/L in a butter factory have been reported) ( 18 ). In the wastewater from other dairy plants, it usually does not exceed 0.1 g/L ( 18 ).
Due to their high organic content, represented mainly by rapidly assimilable carbohydrates and slowly degradable proteins ( 20 ) and lipids, dairy wastewater is characterised by high BOD and COD values varying from 0.1 to 100 g/L ( 3 , 11 , 27 , 39 ). It is known that there is a direct relationship between the ultimate 20-day BOD (BOD u ) and COD values in dairy wastewater, as shown in Eq. 2:

It should be taken into account that such a logical connection cannot be made between a 5-day BOD (BOD 5 ) and BOD u , and between BOD 5 and COD. Therefore, BOD 5 value of dairy waste streams is not an objective indicator of organic pollution ( 18 ). Nevertheless, many authors use the BOD 5 value of dairy wastewater in the BOD 5 /COD ratio. For dairy effluents this ratio varies between 0.4 and 0.8 ( 10 , 12 , 20 , 24 ). However, it should be determined separately in every particular case ( 40 ) and, since dairy wastewater is industrial, the BOD analysis should be conducted with selected microbial consortia, instead of traditional seeding material in order to achieve reliable results ( 41 ).
The highest whey COD and BOD 5 concentrations have been reported to be between 60–80 and 30–50 g/L, respectively. About 90% of BOD and COD loading is caused by lactose, while protein removal contributes to only around 12% of the whey COD reduction. High lactose solubility increases soluble COD part, which is removed mostly by biological units. Like whey, milk and whey permeates have high COD load because they are rich in lactose, which excludes the possibility for a direct discharge in water bodies ( 15 , 24 ). Cheese whey wastewater also has increased concentrations of organic matter, the values varying significantly: 0.8–77 g/L of COD and 0.6–16 g/L of BOD 5 . The lower lactose concentration reported is due to the fermentation in anaerobic conditions that leads to a lower initial pH and casein precipitation and odour production from the obtained butyric acid ( 2 ).
The time-consuming BOD analysis requires the application of faster methods that determine aerobically digestible organic matter in dairy wastewater. Many authors show that COD fractionation combined with the calculation of respirometric oxygen uptake rate is a good alternative method for the determination of wastewater biodegradability ( 42 – 44 ). However, results were obtained only for mixed dairy wastewater ( 45 – 47 ), while the information on single manufacturing processes is insufficient ( 48 ). Total organic carbon (TOC) calculation also includes organic carbonaceous fractions. It gives immediate results and can be used for online measurements. However, the TOC-BOD relationship should be estimated first ( 49 ). There is no available scientific data for the online TOC application or the TOC-BOD relationship in dairy wastewater treatment.
Every milk effluent has notably different TN and TP concentrations ( 3 ). Nitrogen exists mainly in the form of amino groups from milk proteins. Other nitrogenous compounds are also detected: urea, uric acids, and NH 4 + , NO 2 ¯ and NO 3 ¯ ions ( 11 , 27 ). Small quantities of nitrogen ammonium salts originating from ammonia compressors can also be found ( 18 ). Phosphorus compounds are mostly inorganic, phosphate (PO 4 3– ) and diphosphate (P 2 O 7 4– ), but they can also be present in organic form ( 11 ). Total nitrogen content in the wastewater from urban dairies, dairy and butter plants is 4.2–6% and that from cheese factories 3.7% of the BOD 5 . The phosphorus concentration is in the 0.6–0.7% range of the BOD 5 . The reported TN and TP values demonstrate an increased eutrophication risk in water receivers. Their concentrations are sufficient for normal biological treatment processes and the respective growth of bacteria involved in the oxidation of dairy wastewater impurities. However, cheese effluents lack in nitrogen for proper aerobic biological treatment due to the following C/N/P ratio of approx. 200:3.5:1 but can easily be treated anaerobically ( 50 ). During biological treatment of cheese factory wastewater, nitrification is less intense than in other dairy industry wastewater treatment facilities because of the lower BOD 5 /N ratio ( 2 , 12 , 18 ).
Dairy effluents are characterised by very low alkalinity (approx. 2.5 g/L expressed as CaCO 3 in milk permeate), thus bringing about a potential for rapid acidification and increased reagent costs for pH maintenance during purification ( 15 , 24 ).
The high salinity of industrial dairy effluents causes a non-volatile suspended solid content increase in the primary and secondary sludge. Inorganic impurities in dairy wastewater are represented by Na + , K + , Ca 2+ and Cl¯ ions, with their highest amounts in cheese and cottage cheese production (0.46–10%), mostly NaCl and KCl (>50%) as well as Ca 3 (PO 4 ) 2 , where salt is added in advance. Increased Na + amounts indicate the application of alkaline cleaning agents in milk factories. The amount of Ca 2+ in acidic whey is twice as high as that in sweet whey ( 2 , 12 ). The presence of chlorides in dairy wastewater is due to the addition of salt in the production of brine and cooling liquors, and the Cl¯ concentration in fresh water and milk. Cl¯ concentration in dairy wastewater reaches 0.8–1 g/L but the average value range is 0.15–0.2 g/L.
The additional wastewater pollution due to the used cleaning solutions, additives and other products which enter the drainage pipes should be taken into account ( 4 , 18 ). CIP methods produce wastewater streams at 12- or 24-hour time intervals, while sanitisers are used if the dairy factory has been shut down for more than 96 h. Thus, wastewater pH will change widely depending on the cleaning program applied ( 23 ). Different chemical solutions can be used in accordance with the installation type, water hardness, etc . ( 4 ). The cleaning agents applied in CIP procedures affect principally the effluent pH (mineral and organic acids), contributing less than 10% to BOD 5 and COD loading and increasing amounts of water for cleaning and disinfection (up to 30% of total water flow rate). Most of the applied chemicals are very toxic to microorganisms in secondary treatment units. NaOH and HCl increase the mineral scale ( 1 ), while HNO 3 , quaternary ammonium surfactants, and detergents containing H 3 PO 4 and P influence TN and TP loading, which leads to an accelerated eutrophication of the environment if not treated properly ( 6 ). Due to the above-mentioned environmental problems, the trend is to apply more HNO 3 instead of the less desirable H 3 PO 4 although the latter is a better cleaner the application of which will not be reduced in the future. The cleaning solutions utilised in CIP procedures are hot (64–82 °C), which causes a temperature increase in the resulting effluents ( 4 ). Strong oxidants or bleaches (NaOCl and ClO 2 ) are applied for sanitising installations. Cl-containing bleaching agents can produce dangerous organochlorides which pollute dairy effluents. Enzymes as well as surfactants are the chemicals preferred for cool surface cleaning and cause fewer negative environmental problems ( 6 ). In minor doses, the following substances can also be found: NH 3 , Na 3 PO 4 , HCl, HOCH 2 COOH, Na 2 SiO 3 , hydraulic oil, propylene glycol, emulsifiers, antifoaming agents, sodium azide and chloramphenicol ( 4 , 51 ).
Dairy Wastewater Treatment
Dairy manufacturing has a strong impact on the environment, producing large volumes of wastewater with high organic and nutrient loading and extreme pH variations. This requires the application of effective and cheap wastewater treatment procedures which ensure fresh water preservation ( 1 , 4 ). There are various dairy effluent treatment strategies ( Fig. 1 ), which are described in the following paragraphs.

Dairy wastewater treatment options, adapted from ( 19 )
Discharge in nature without treatment
It is not recommended that raw dairy wastewater be discharged directly into water bodies because this would lead to different pollution problems, including rapid dissolved O 2 depletion due to the high organic loading, which results in anaerobic conditions, the release of volatile toxic substances, aquatic life destruction and subsequent environmental damage. Higher water temperatures decrease O 2 solubility and increase biota sensitivity ( 1 ).
Treatment in wetlands
Wetland systems use natural processes that include self-supported microbial communities to improve wastewater treatment ( 52 ). The simple construction and the lack of sludge recycling make them preferable for dairy effluent utilisation in developing communities ( 52 , 53 ). The main drawbacks of their application include the need for a large surface area, the potential risks for surface and groundwater pollution, the presence of dangerous volatile substances and the presence of insects. The easy exploitation of the systems counteracts with the complexity of the biological processes, which exceeds that of other treatment systems applied in wastewater purification. Also problematic is the generation of Fe 3+ , Mn 3+ and Ca 2+ ions. They precipitate and reduce bed permeability with time. As a result, anaerobic conditions prevail and the NH 3 removal is limited ( 1 ).
Generally, dairy wastewater is treated in wetlands under aerobic conditions. Five days are enough for an 85% BOD 5 reduction in aerobic ponds with milk wastes at 20 °C, while high-load dairy wastewater is treated mostly in facultative wetlands ( 1 ). In a butyl-covered lagoon, processing effluent was biodegraded at 35 °C, with the organic loading rate (OLR), expressed as COD, of 1.5 kg/(m 3 ·day), neutral pH and a hydraulic retention time (HRT) of 1–2 days. However, a polishing step in aerated pond is necessary to achieve 99% total COD reduction ( 1 , 4 ). A surface-flow wetland was applied to utilise 2.65 m 3 /day of milkhouse wastewater with OLR, expressed as BOD 5 , of 7.3 g/(m 2 ·day) ( 54 ). The results showed high TSS, BOD 5 , TP and total Kjeldahl nitrogen (TKN) biodegradation with respective values of 94, 85, 68 and 53%. Despite the fact that the lagoon produced NH 3 , its outflow concentrations gradually declined over time. Most of the nitrogen was stored in biomass, while denitrification had a minor role (<1%) ( 54 ). Clarified effluents needed more BOD 5 reduction to meet water discharge standards ( 55 ). Cheese wastewater with OLR, expressed as COD, of 5.5 kg/(m 3 ·day) was consistently treated in a grease trap, an upflow anaerobic sludge blanket (UASB) reactor-type pond, aerobic pond and a final wetland with water hyacinth ( 56 ). A high quality effluent was obtained: BOD 5 , COD, TSS, FOG, organic N and total coliforms were reduced by more than 90%, except for the phosphorus from PO 4 3– (with a decrease of only 62%).
Purification in urban or in-factory wastewater treatment plant
In-plant effluent treatment is the most common strategy for dairy wastewater purification ( 1 ). Typically, it includes mechanical, physicochemical, chemical and biological methods.
Mechanical treatment
Mechanical treatment removes suspended solids from wastewater. Conventional mechanical procedures reduce insufficiently the organic load because of the low settleable solid concentration in dairy wastewater ( 5 ). Nevertheless, the faster the wastewater is screened, the better, due to less TSS biodegradation and a low soluble COD increase ( 1 ).
High variations of dairy effluents can bring about an instability of the subsequent treatment facilities. Adequate equalisation will smooth the fluctuations in the flow, organic loading, pH and temperature, neutralise residual cleaning agents and completely destroy excess oxidisers. In practice, a 24-hour flow pattern at the highest load can be effectively handled by effluent equalisation for at least 6–12 h with a basin dimension from 25 to 50% of the total effluent volume ( 1 , 6 ).
Physicochemical treatment
Physicochemical treatment destroys and reduces milk fat and protein colloids in the dairy wastewater ( 4 ). FOG removal is a major problem in the plants producing unskimmed milk, in milk and whey separation, cheese and butter production, as well as milk bottling. Skimmed milk production rarely creates such problems.
Animal fat is solid at room temperature due to the high levels of saturated fatty acids in its composition. Milk fat is no exception. This physical state, combined with the low density of the fat allows its easy removal from the surface of wastewater ( 6 , 57 ). If the equalisation unit precedes the FOG trap, a temperature drop will heighten the risk of high fat accumulation on the top of the liquid. Otherwise, the equalisation unit must have a sufficient volume to collect the peak effluent flow. In general, flow balancing is followed by FOG removal. Increased wastewater temperatures can reduce fat separation ability ( 1 , 5 ). Dissolved air flotation is more effective because it reduces organic loading via protein and fat colloid destabilisation with coagulants (Al 2 (SO 4 ) 3 , FeCl 3 and FeSO 4 ) and flocculants. Nevertheless, this method requires expensive, synthetic chemicals which causes environmental problems and removes soluble matter to a lesser extent ( 58 ). The resulting scum is very hard to dewater and it is not recommended to mix it with activated sludge. Scum must be treated properly before disposal ( 1 , 4 ). If inorganic and synthetic chemicals are replaced by biopolymers (carboxymethyl cellulose (CMC) or chitosan), processed sludge can be used as an animal food ingredient ( 59 , 60 ).
According to some authors ( 60 – 62 ) natural coagulation in dairy wastewater can be achieved with the application of certain lactic acid bacteria. These bacteria ferment soluble lactose to lactic acid, which denatures milk proteins in the wastewater. In combination with CMC, the total COD was reduced by 65–78%, while reduction of 49–82% was obtained when chitosan was used ( 60 ). At an initial 5 g/L of COD, over 0.01 g/L of proteins and 0.7–0.8 g/L of sugars, 75, >90 and 10–25% of COD were removed, respectively ( 61 ).
Chemical treatment
Chemical treatment removes mostly colloids and soluble contaminants from milk processing effluents. It includes reagent oxidation or pH correction. During cheese wastewater reaction with FeSO 4 and H 2 O 2 , up to 80% of fat (initial concentration of 1.93 g/L) is removed ( 63 ). Extreme pH values of dairy wastewater below 6.5 and above 10 can increase the corrosion of pipes and be highly detrimental to microbiological assemblages in biological processes. Therefore, they should be corrected to reduce side effects. If a dissolved air flotation (DAF) unit is used, then the pH control is a necessary step to achieve optimal coagulant conditions ( 64 ). However, coagulants work best at an acidic pH, which requires a second pH adjustment to a neutral value before biological treatment ( 65 ). It is very suitable to collect independently used CIP solutions and outflow them constantly during the whole wastewater plant exploitation ( 1 , 4 ).
Biological treatment
One of the most reliable methods for dairy effluent purification is biological removal. Such methods can assimilate all dairy wastewater components but they mostly utilise soluble compounds and small colloids. These processes have not been fully studied. Moreover, because of their unlimited adaptation potential, they can be jointly used in various sequences to meet certain component biodegradation requirements ( 1 , 7 ). Biological treatment has two main branches depending on oxygen requirements: aerobic and anaerobic processes ( 66 ).
Aerobic processes. Nowadays, most dairy wastewater treatment plants are aerobic although they have been less efficient, mainly due to filamentous growth and rapid acidification caused by high lactose levels and low water buffer capacity, respectively ( 4 , 12 , 67 ). Problems generally encountered with activated sludge processes are bulking and foaming, which diminish sludge settling, Fe 3+ and CO 3 2– precipitation, additional biomass production as well as poor activity at low temperatures. It takes a few months for the sludge adaptation before full operational capacity is reached. Nitrogen from NH 3 is easily degraded. Phosphorus removal is less effective and relies on environmental conditions. Aerobic bacteria are less useful in colloid utilisation when compared to anaerobic bacteria. The heightened O 2 depletion (>3 kg of O 2 per kg of BOD 5 ) requires large energy demands during the aerobic treatment of concentrated dairy wastewater (>2 g of COD per L) ( 1 , 4 ). Plug flow systems are better than complete-mix processes since they are less sensitive to high organic load problems like bulking sludge, etc . ( 21 ). Commonly, dairy effluent OLR, expressed as BOD 5 , should be less than 0.28–0.30 kg/m 3 . To enhance biological removal, a proper pretreatment or adequate wastewater dilution should be applied ( 1 , 68 ).
Aerobic biological systems give a very positive response during synthetic dairy wastewater treatment with 4 g/L of COD and 1 g/L of TKN at pH=11.5, with over 96% of degradation being achieved in a continuous mode ( 69 ). An artificial effluent similar to milk powder and butter processing wastewater was treated in an anaerobic- -anoxic-oxic system at HRT of 7 days and a nominal sludge age of 20 days ( 70 ). The process was characterised by sludge bulking due to the growth of filamentous bacteria ( Sphaerotilus natans , Type 0411 and Haliscomenobacter hydrossis ). TN removal remained unchanged at 66% without the improvement in the sludge volume index. TP depended on the anoxic selector relative dimensions (from 49 to 20%) and a respective nitrate rise in the effluent. Nevertheless, more than 90% of COD reduction was achieved.
Aerobic filters are applied to a lesser extent in the treatment of high-strength dairy effluents rich in FOG. High fat and heavy biofilm blockage are possible, which results in biomass loss, filter fouling and corresponding reduction in productivity ( 1 ).
The sequencing batch reactor (SBR) is preferred in dairy wastewater treatment because of its various loading capabilities and effluent flexibility. A traditional technology with free sludge flocs is mostly applied. The purification of milk effluents is given by Britz et al . ( 1 ). COD was reduced by 91–97, TS by 63, volatile solids (VS) by 66, TKN by 75, and TN by 38%. However, mechanical treatment had to be applied first. Another study shows the aerobic SBR as an excellent example of the combination of activated sludge granulation with dairy effluent treatment ( 71 ). Granulation stability is limited by nutrient concentration in the wastewater, while effluent quality depends on the need for preliminary sludge settling, usually 0.25–0.5 HRT. Up to 90% of total COD, 80% of TN and 67% of TP were reached in an 8-hour cycle and 50% volume exchange ratio. The results were obtained after fully activated sludge granulation and consecutive biomass sedimentation. The soluble effluent COD was reduced to 125 mg/L. Industrial effluents are more difficult to treat than synthetic ones. The lower maximum OLRs also reduced the SBR granular sludge efficiency ( 17 ). In a bench-scale SBR, raw industrial dairy wastewater was treated with Lactobacillus casei TISTR 1500 ( 62 ). Microaerobic conditions maintained in the SBR allow for biomass accumulation in large amounts, leading to 85% lactose reduction via rapid fermentation and subsequent protein coagulation by 90%. As a consequence, 70% of COD degradation can be achieved. Around 2.67 times higher OLR was achieved in two laboratory aerobic SBRs treated with a mixed landfill and dairy effluent than in traditional SBR processes ( 71 ). The best BOD 5 removal mode was reached at OLR, expressed as BOD 5 , of 0.8 kg/(m 3 ·day) per a 10- -day HRT. The application of flexible fibre as an activated sludge carrier increases the laboratory SBR reliability and it is possible to treat dairy effluents at very high OLRs. At OLR, expressed as COD, of 0.4 kg/(m 3 ·day), COD was degraded by more than 89% and up to 97% at OLR, expressed as COD, of 2.74 kg/(m 3 ·day) ( 72 ). Membrane technologies are successfully applied in the treatment of low-load dairy effluents in an SBR. A high BOD removal (over 97%) and TSS-free wastewater are obtained. Due to low influent loading, TN removal reaches 96% by means of assimilation only. TP elimination reaches only 80% after system optimisation due to the limited excess sludge disposal ( 73 ).
Moving bed biofilm reactor (MBBR) shows very high performance when applied to dairy wastewaters: OLR increases dozens of times compared to conventional activated sludge systems. A milk processing effluent was treated in a MBBR with biomass developed on FLOCOR- -RMP ® particles (Henderson Plastics Ltd, Norfolk, UK) ( 74 ). At OLR, expressed as COD, of 5 kg/(m 3 ·day), more than 80% of total COD degradation was achieved in almost half-order kinetics with partial substrate penetration. TN was decreased by 13.3–96.2%. The small reactor volume and the high OLR encompass process applications including plant renovation and the introduction of new, limited-space treatment facilities ( 74 ). A novel MBBR with free-floating plastic elements (with a density slightly less than 1.0 kg/m 3 ) may give 85 and 60% COD reduction at OLRs of 12 and 21.6 kg/(m 3 ·day), respectively. On the basis of test results, we can say that the MBBR should be very suitable for the treatment of dairy industry effluents ( 75 ).
Good results can be reached in a membrane bioreactor during the treatment of an ice-cream factory effluent with 13.3 kg/m 3 of COD, 6.5 kg/m 3 of BOD 5 at a temperature of 25 °C. Both indicators are reduced by over 95%, while TKN is decreased by more than 96 and TP by 80%. Under aerobic conditions, the indigenous microflora composed of lactic acid bacteria may reach over 10 9 CFU/mL, which will downgrade CIP-induced alkaline pH variations ( 76 ).
Various alternatives for aerobic treatment of dairy effluents are also used. Pure oxygen is another possibility in the biodegradation of milk wastewater. Oxygen can be applied directly in the homogenisation tank during a traditional physicochemical treatment and stable operation is achieved under a broad initial COD and TSS range. This modification improves effluent quality and reduces process costs. Such oxygen injection systems can replace the expensive anaerobic treatment and are naturally safer ( 77 ). Cheese whey can also be successfully utilised as a cheap medium for edible mushroom cultivation. Some authors report the growth of Ganoderma lucidum on protein-free cheese whey. The best soluble COD utilisation was achieved at pH=4.6 and 27.1 °C, while the maximum mycelial yield of 0.35 mg per mg of soluble COD removed was obtained at pH=4.2 and 28.5 °C ( 78 ). Although there is information on edible fungal growth, dairy wastewater utilisation has not been studied from a COD point of view ( 79 – 82 ).
Cheese whey effluents can be treated successfully in municipal wastewater treatment plants. Factories with onsite treatment technologies should collect sanitary wastewater independently from processing effluents and discharge them directly into municipal wastewater treatment plants. Nevertheless, such a treatment option can lead to operational problems with secondary treatment units ( 1 , 12 ). Periodic sludge bulking is possible and is caused by intermittent high soluble COD levels in the receiving sewage plant.
Anaerobic processes. Anaerobic systems are more suitable for the direct utilisation of high-strength dairy wastewater and are more cost-effective than aerobic processes. If properly operated, these systems do not produce unpleasant odours ( 1 , 4 ). The major problems of anaerobic dairy wastewater treatment include long start-up periods due to complex substrate degradation, preliminary biomass adaptation prior to protein and fat utilisation, fast drop in pH and a resultant inhibition of methane production (as a consequence of the high concentration of easily fermentable lactose and low substrate alkalinity), sludge disintegration by fats in the form of triglyceride emulsions and subsequent biomass flotation, presence of inhibitory compounds (long-chain fatty acids, K + and Na + ions), inability of ammonia biodegradation and phosphorus removal, careful management, increased sensitivity to various OLRs and shock loadings, etc . Notwithstanding the little information on industrial-scale anaerobic plants utilising cheese whey, more than 75% COD removal and around 10 kg / (m 3 ·day) of OLRs, expressed as COD, are achieved. The degree of biodegradation depends on the HRT applied ( 4 , 12 , 22 , 83 – 85 ).
Milk processing effluents are predominantly treated in conventional one-phase systems: upflow anaerobic sludge blanket (UASB) reactor and anaerobic filter (AF) are most commonly applied ( 4 ). UASB reactors have been used in industrial dairy wastewater treatment for more than 20 years. They are suitable for treatment of overloaded effluents with COD higher than 42 g/L ( 86 ). Laboratory scale UASB reactors utilising whey permeates in a continuous regime have been designed ( 87 ). Kinetic coefficients using the Monod equation are determined per HRT of 0.4–5 days and an initial wastewater COD of 10.4–0.2 g/L ( 87 ). It was shown by a comparative study of the possibility of using flocculent sludge and the effect of different HRTs (6–16 h) on the anaerobic UASB reactor behaviour applied to dairy wastewater treatment that nearly 80% of protein mineralisation, soluble COD and volatile fatty acid degradation as well as over 60% fat removal can be reached at an HRT of at least 12 h and an OLR, expressed as COD, of less than 2.5 g/(L·day) ( 88 ). Biomass granulation was also achieved in the UASB reactor within 60–70 days. Of all the elements studied, only Ca 2+ ions had any significant effect ( 89 ). When treating a synthetic ice-cream effluent in the UASB reactor, TOC was reduced by 86% at an HRT of 18.4 h, with the highest OLR, expressed as TOC, reaching 3.06 kg/(m 3 ·day) ( 1 ). High FOG degradation is also possible in an UASB reactor. A couple of bench-scale UASB reactors were successfully employed during the utilisation of a synthetic milk effluent rich in FOG (0.2, 0.6 and 1 g/L) ( 90 ). Enzymatic pre-hydrolysis contributed to 8% more COD removal at the highest FOG concentration ( 90 ). Cheese effluents are degraded in UASB reactors in laboratory tests and on an industrial scale. A laboratory-scale UASB reactor utilising a cheese factory effluent eliminates around 90% of effluents at an OLR, expressed as COD, of 31 g/(L·day) ( 91 ). Organic loads, expressed as COD, over 45 g/(L·day) perform worse (70–80% only). Moreover, chemicals are needed to support a constant pH. Short-shock OLR during operation increases sludge granulation, improving stability in reactor performance. The results of the laboratory tests on an industrial level have been confirmed ( 1 ), improving them by 6% per 10% higher load. A full-plant UASB reactor can be applied in cheese factory wastewater treatment. With an initial COD of 33 g/L, HRT of 16 h and OLR, expressed as COD, of 49.5 kg/(m 3 ·day), 86% degradation can be reached. During the utilisation of an industrial effluent from Edam cheese, butter and milk production, a full-scale UASB reactor can be applied, the COD being decreased by 70% ( 1 ).
Dairy effluents with a low TSS can be successfully utilised in AFs in an all-scale range. The COD decreased by between 60 and 98% at a HRT of 12–48 h and an OLR, expressed as COD, of 1.7–20 kg/(m 3 ·day) ( 1 ). A large specific surface of the filter media creates a precondition for higher biomass accumulation which is less affected by shear stress. A five-time higher load than with the non- -porous filler under the same conditions is achieved. It has been reported that with a couple of mesophilic upflow AFs utilising a milk bottling effluent, the reactor with the porous packing performed better (OLR, expressed as COD, of 21 kg/(m 3 ·day)) than the same reactor with non-porous packing (OLR, expressed as COD, of 4 kg/(m 3 ·day)), which is influenced by shear stress to a greater extent ( 92 ). Different temperature regimes can be analysed during the treatment of dairy wastewater in laboratory upflow AFs. At 12.5, 21 and 30 °C and HRT of 4 days on average, the COD removal in each reactor amounted to 92, 85 and 78%, respectively ( 93 ). An AF was used to treat ice-cream wastewater in a comparative study with contact process, UASB reactor and fluidised bed bioreactor (FBB) ( 94 ). The data showed a COD removal of 67, 80 and 50% at OLR, expressed as COD, of 6, 1 and 2 kg/(m 3 ·day) and 60% of total COD removal, at OLR, expressed as COD, of 2–4 kg/(m 3 ·day). All reactors had a poor biomass retention resulting from FOG loading. An upflow AF performed better, which allowed its full-scale installation in the manufacturing process ( 94 ). An upflow AF has been claimed to be unsuitable for the anaerobic digestion of very dilute dairy wastewaters ( 95 ). In fact, continuous stirred-tank (CSTR), UASB and baffled reactors also cause problems although experimental data show that the baffled reactor performs better with an OLR, expressed as VS, of 0.117–1.303 g/(L·day) and HRTs between 18.8 and 2 days.
Although a CSTR is a good option for scientific research of complete-mix systems ( 96 ), it is difficult to use it on an industrial scale because of HRT restrictions. Such reactors were studied with a cheese effluent consisting of wash water/whey ratio of 4:1 with 17 g/L of COD. However, problems with sludge loss arise if the HRT drops to below 9 days ( 1 ).
Milk processing effluents can be treated in hybrid systems too ( 4 ). An anaerobic contact digester may reach a COD degradation of over 80–95% under mesophilic conditions. The main disadvantage is the difficult sludge settlement. However, the technology is applied worldwide in dairy plants although it is quite old ( 1 ). A laboratory-scale experiment analysed the kinetic performance of anaerobic synthetic ice-cream effluent at 37 °C applying the Monod and Contois equations at an HRT range between 2.99 and 7.45 days. A better explanation of the kinetic coefficients can be achieved in the final pilot-scale plant since it allows variations in the initial substrate concentration ( 97 ).
Anaerobic packed-bed bioreactor (PBB) can be successfully applied for dairy wastewater treatment of various organic loads. A downflow PBB was used for treating deproteinised cheese whey with 59 g/L of COD ( 1 ). At OLR, expressed as COD, of 12.5 kg/(m 3 ·day), the system decreased the COD to 90–95% at HRT of 2–2.5 days. The influent pH was around 2.9, while the pH in the reactor was almost neutral. Good results were obtained in a pilot--scale plant with an up-flow anaerobic PBB ( 98 ). The initial cheese whey COD was 59.4 g/L. A 16-hour HRT was enough to reach 99.4% of lactose conversion. Whey wastewater was degraded to 89% in an anaerobic MBBR at (35±2) °C per 1-day HRT and an OLR, expressed as COD, of 11.6 kg/(m 3 ·day) ( 99 ). The cheese whey was decomposed in a laboratory PBB with a polyethylene carrier. The highest COD reduction was achieved at a 3.5-day HRT with OLR, expressed as COD, of 3.8 kg/(m 3 ·day) and biogas production of 0.42 m 3 per kg of COD per day ( 1 ). The mesophilic anaerobic fluidized-bed bioreactor system degraded 5.2 g/L of COD in the ice-cream wastewater to 94.4% at 35 °C, OLR, expressed as COD, of 15.6 kg/(m 3 ·day) and HRT of 8 h. Under shock loading, the return to steady-state conditions was possible within 6–16 h ( 100 ). The fluidized-bed bioreactor was used to treat a low-load milk effluent with 0.2–0.5 g/L of COD. At an 8-hour HRT, 80% of COD was removed ( 1 ).
Membrane applications in anaerobic systems are good options for improved effluent filtration combined with a higher concentration and an effective differentiation between HRT and solids retention time. A completely mixed anaerobic microfiltration membrane reactor system was used on cheese whey high in COD (63 g/L) ( 1 ). More than 99% of organic matter was utilised when HRT was 7.5 days, which allowed authors to upgrade the studies from the pilot plant to a full-scale demonstration. The application of the ultrafiltration system made it possible to achieve a higher biomass retention for more efficient wastewater treatment.
Different temperature conditions have been tested in order to reach a higher COD anaerobic removal. The psychrophilic anaerobic operation in some laboratory hybrid reactors, utilising whey effluents with low (COD of 1 kg/m 3 ) and high (COD of 10 kg/m 3 ) load, showed a better COD performance when the OLR reached 70–80% in the first reactor (at OLRs, expressed as COD, of 0.5–1.3 kg/(m 3 ·day), in a 20–12 °C range) and more than 90% in the second (at OLRs, expressed as COD, up to 13.3 kg/(m 3 ·day), in a 20–14 °C range) ( 101 ). If the high-load reactor was operated at 12 °C, COD removal decreased to 50–60% and biogranule decomposition started. These side effects could be eliminated via an OLR reduction down to 6.6 kg/(m 3 ·day). However, dairy wastewater has higher average temperature, which makes it possible to apply high-load wastewater treatment technologies ( 6 , 18 ). Another study showed that mesophilic conditions ((36±1) °C) generate more H 2 compared to thermophilic ones ((55±1) °C) during the treatment of cheese whey wastewater, with 9.2 and 8.1 mmol of H 2 per g of COD, respectively. The specific H 2 production was 4.6 times higher at 36 than at 55 °C ( 102 ).
Separated-phase systems are preferred from technological point of view. They have the highest organic loading and shortest HRT compared to other anaerobic digesters. The consecutive acidogenic-methanogenic phase division of anaerobic digestion is suitable for the treatment of dairy wastewater with an unbalanced composition (high C:N ratios which acidify very quickly). In such separated-phase systems, the acidogenic reactor has a major role as it supplies short-chain volatile fatty acids which can be easily fermented to CH 4 in the methanogenic reactor. The easily utilisable lactose requires a shorter HRT and a smaller volume of the acidogenic reactor than the methanogenic digester ( 1 , 4 , 103 ). Such a system was used to treat a dairy effluent with 50 kg/m 3 of COD and pH=4.5. The COD was decreased by 72% at 35 °C and the following operating conditions: OLR, expressed as COD, of 50 and 9 kg/(m 3 ·day), when HRT was only 1 and 3.3 days in the acidogenic and the methanogenic reactors, respectively ( 1 ). The CSTR was the preferable model for the acidogenic phase. In a 9-month operation study, a two- -phase anaerobic reactor comprising an acidogenic-phase CSTR and a methanogenic-phase upflow AF was used to treat dairy waste streams ( 104 ). The effluent COD was reduced by 90% and the BOD 5 by 95%, while an OLR, expressed as COD, of 5 kg/(m 3 ·day) and a 2-day HRT were obtained. The H 2 and subsequent CH 4 production from fresh cheese whey were achieved in a CSTR, at 35 °C and HRT of 1 day. The mixed liquor was consequently fermented to CH 4 in a baffled bioreactor, operated at HRTs of 20, 10 and 4.4 days. At the lowest HRT, the COD reduction reached 94% ( 105 ). An acidogenic CSTR and a final methanogenic upflow AF were used to utilise cheese whey. The results showed that a maximum acidogenesis of up to 50%, with the same OLR (expressed as COD) range (0.5–2 g per mixed liquor suspended solids per day) could be achieved at an HRT of 24 h. The effluent was fed subsequently to the upflow AF where the initial soluble COD was decreased by 90% during HRT of 4 days ( 106 ). A two-stage hybrid UASB reactor, filled respectively with polyurethane foam and polyvinyl chloride rings in each phase, was supposed to exceed other anaerobic methods in the treatment of dairy effluents. The combined COD removal in the reactor in a stable equilibrium (10.7 to 19.2 kg/(m 3 ·day)) changed from 97 to 99% ( 39 ). Anaerobic rotating biological contact reactors are also discussed in the literature for anaerobic separate phase treatment ( 1 ). Carrier incorporation into anaerobic reactors for biomass support greatly increases their specific activity. Depending on the operating temperature, dairy wastewater can be treated in a two-phase separation. The basic configuration presupposes that thermophilic acidogenesis is followed by mesophilic methanogenesis. The information on these processes in the literature is scarce ( 107 – 109 ). An experiment compared two couples of anaerobic SBRs working at the following temperatures: the first couple (thermophilic-mesophilic system) at 55–35 °C and the second (mesophilic-mesophilic system) at 35–35 °C. At an OLR, expressed as VS, varying between 2–4 g/(L·day), the thermophilic-mesophilic system performs better (VS removal rate of 43.8–44.1% when HRT is 3 days and 37.1–38.9% when HRT is 6 days) than the mesophilic-mesophilic system (VS removal rate of 29.3– 30.2% when HRT is 3 days and 26.1–29.1% when HRT is 6 days). The overall improved performance showed that the thermophilic-mesophilic system with respect to total coliform reduction, TSS removal and biogas production, is preferable to the mesophilic-mesophilic SBR couple. Despite that, higher energy consumption during the thermophilic phase should be taken into account from an economical point of view ( 84 ). During a set of experiments, a high-temperature-based technology including acetic and butyric acid fermentation followed by CH 4 production achieved 116% COD reduction and 43% CH 4 biosynthesis, thus performing better than single- -phased processes ( 110 ).
Combined (anaerobic-aerobic) processes. Since an anaerobic technology reduces mostly C-containing contaminants and has a weaker effect on nutrient removal, it needs to be considered as only a preliminary step which must be polished. This can be achieved by incorporating a local aerobic step or, occasionally, by directly discharging anaerobic effluent into the municipal wastewater treatment plants ( 4 ).
A mixed dairy wastewater was purified on a full- -scale level in consecutive UASB reactor and aerobic denitrification steps. When 95% COD removal was achieved, the produced CH 4 was sufficient to cover the plant energy requirements ( 1 ).
SBR great flexibility makes it an adequate post-aerobic step in combined dairy wastewater treatment. A new downflow-upflow hybrid reactor containing downflow pre-acidification and upflow methanation chambers was designed to treat high-load cheese wastewater at an average OLR, expressed as COD, of 10 g/(L·day). COD (98%) was converted into biogas, while the discharged soluble COD reached 1 g/L. The process was maintained at stable pH values without chemical addition. After treatment in the SBR, more than 90% of COD, nitrogen from NH 3 and TP were removed ( 32 ). Wastewaters from raw milk quality laboratories, containing milk preservatives (sodium azide or chloramphenicol), were utilised in an industrial-scale plant with an AF and SBR. Influent FOG were completely treated in the anaerobic step without biomass washout for more than 2 years of operation, the COD decrease being more than 90% at an OLR, expressed as COD, of 5–6 kg/(m 3 ·day). However, alkali had to be added to reduce the critical pH drop. The outgoing stream from the anaerobic process was polished in SBR until the final COD dropped to 200 mg/L and the TN to less than 10 mg/L ( 52 ).
The consecutive anaerobic-aerobic technology was used to purify reconstituted whey wastewater in a single reactor at low oxygen concentration and 20 °C. Maximum COD removal of (98±2) % was reached at total cycle time of 4 days and OLR, expressed as COD, of 0.78 g/(L·day). In accordance with specific biomass activity, trophic differentiation can be seen in the system: methanogens predominantly live at the bottom of the bulk liquid, while acidogens inhabit suspended flocs. When the soluble O 2 rose to 0.5 mg/L during the aerobic phase, the COD was reduced to (88±3) % in a 2-day total cycle time at 1.55 kg/(m 3 ·day) ( 111 ).
Conclusions
The discontinuous manufacturing process and high production heterogeneity in milk processing make it hard to outline the general dairy wastewater characteristics. Nevertheless, it can be concluded that dairy factories are large water consumers and therefore produce unstable waste streams with increased temperatures, variable pH values, high COD, BOD, FOG, N and P concentrations in combination with inhibiting cleaning agents and strong fluctuations in all factors. However, there is little information on the composition of wastewater streams from certain dairy industry branches, such as the production of yoghurt and whey products, which require more attention in future research.
Conventional aerobic activated sludge systems and percolating filters are not appropriate for dairy wastewater treatment. The high soluble COD values in wastewater account for the vast filamentous growth, which obstructs proper treatment and plant management. The application of immobilised biofilm technologies offers the opportunity to treat concentrated wastewater. MBBR are promising systems. However, many studies should be performed on other dairy wastewater streams, such as high FOG effluents, acid whey, etc .
High organic contamination levels create conditions for the preference of anaerobic digestion over aerobic processes in dairy wastewater utilisation although anaerobic treatment rarely produces clear streams. This necessitates the development of novel, more effective fermentation technologies to deal with high-strength dairy effluents. Insufficient information on temperature-phased anaerobic biodegradation paves the way for new research on dairy wastewater management. A major problem in the anaerobic fermentation of dairy wastewater is ammonia, known for its toxicity if generated in high concentrations. Research can contribute a lot to the anaerobic ammonium oxidation application in the treatment of anaerobic effluents from dairy manufacturing for an improved nitrogen removal.
The consecutive combination of fermentative and oxygen processes may be a solution for appropriate milk processing wastewater treatment. However, innovative and more compact equipment should be designed to meet the challenges associated with wastewater treatment limitations and water-quality requirements. Moreover, the replacement of outdated equipment with new machines needs to be supported by more, real-case studies, which will help us understand better dairy wastewater treatment.
Open Access is an initiative that aims to make scientific research freely available to all. To date our community has made over 100 million downloads. It’s based on principles of collaboration, unobstructed discovery, and, most importantly, scientific progression. As PhD students, we found it difficult to access the research we needed, so we decided to create a new Open Access publisher that levels the playing field for scientists across the world. How? By making research easy to access, and puts the academic needs of the researchers before the business interests of publishers.
We are a community of more than 103,000 authors and editors from 3,291 institutions spanning 160 countries, including Nobel Prize winners and some of the world’s most-cited researchers. Publishing on IntechOpen allows authors to earn citations and find new collaborators, meaning more people see your work not only from your own field of study, but from other related fields too.
Brief introduction to this section that descibes Open Access especially from an IntechOpen perspective
Want to get in touch? Contact our London head office or media team here
Our team is growing all the time, so we’re always on the lookout for smart people who want to help us reshape the world of scientific publishing.
Home > Books > Technological Approaches for Novel Applications in Dairy Processing
Physico-Chemical Treatment of Dairy Industry Wastewaters: A Review
Submitted: 06 September 2017 Reviewed: 10 April 2018 Published: 05 November 2018
DOI: 10.5772/intechopen.77110
Cite this chapter
There are two ways to cite this chapter:
From the Edited Volume
Technological Approaches for Novel Applications in Dairy Processing
Edited by Nurcan Koca
To purchase hard copies of this book, please contact the representative in India: CBS Publishers & Distributors Pvt. Ltd. www.cbspd.com | [email protected]
Chapter metrics overview
2,968 Chapter Downloads
Impact of this chapter
Total Chapter Downloads on intechopen.com

Total Chapter Views on intechopen.com
Overall attention for this chapters
Dairy industries have grown in most countries because of the demand in milk and milk products. This rise has led to the growth of dairy industries. The wastewaters discharged from this industry contain high concentrations of nutrients, chemical oxygen demand (COD), biological oxygen demand (BOD), total suspended solids (TSS) and organic and inorganic contents, which can cause serious environmental problems if not properly treated. The conventional biological treatment methods are suitable for dairy wastewaters due to its high biodegradability. However, long chain fatty acids formed during the hydrolysis of lipids show the inhibitory action during anaerobic treatment. Sequencing batch reactor (SBR) and up flow anaerobic sludge blanket (UASB) systems seem to be the most promising technology for the biological treatment of dairy wastewaters. Several research papers have been published on the application of aerobic and anaerobic treatment technologies for dairy industry wastewater, but both treatment methods still have some disadvantages. The most important challenge is to find cost-efficient and environmentally sustainable approaches to enable water reuse and waste management. Therefore, alternative treatment technologies against biological treatment methods such as coagulation, adsorption, membrane and electrolysis processes are under investigation. This chapter provides a critical review focusing on physicochemical treatment technologies of dairy wastewater.
- wastewater treatment
- physicochemical
Author Information
Taner yonar *.
- Faculty of Engineering, Department of Environmental Engineering, Uludag University, Bursa, Turkey
Özge Sivrioğlu
Nihan özengin.
*Address all correspondence to: [email protected]
1. Introduction
Industrialisation has a big role for development of a country which causes serious pollution problems throughout the earth [ 1 ]. With increase in demand for milk and milk products, dairy industries have shown enormous growth in number and size in many countries all around the world [ 2 ]. The total milk production was estimated 818 million tonnes according to the İnternational Dairy Federation’s World Dairy Report 2016, approximately 2% more than 2014 [ 3 ].
Dairy industry is the major source of food processing which has one of the highest consumption of water which used through every steps of dairy industry [ 4 , 5 , 6 ]. Therewith the amount of wastewater discharged from dairy industry has also raised [ 2 ]. For this reason, treatment of dairy wastes becomes very important before disposal [ 7 ]. Therefore, it is necessary to know how the processes take place in dairy industry.
In the dairy industry, the products are very diverse, which are mainly pasteurised and sterilised milk, yogurt, ayran, cheese, cream, butter, ice cream, and milk powder. Wastewater is produced both from production of products and from packaging units.
In the milking process, raw milk is collected from the producers, samples are taken and sent to the factory. Wastewater arises from the water coming from milk cans, storage tanks, washing places and cooling systems.
In the packaging unit, wastewater occurs during the cleaning of bottles, jars, tanks and related equipment with packaging.
In the cream production unit, butter is made with sweet cream and sour cream. Milk is centrifuged to separate the cream from the milk. While the cream-free milk is sent to the needed processes, butter is produced by churning the remaining cream. Wastewater is formed during the washing of the places and the cleaning of the tools.
In cheese making process, there are many steps. These include coagulation of the milk, cutting of the curd, cooking, when draining, placing curd in cheese moulds, and pressing the moulds. The cheese in the moulds is shaped and packaged. The most important wastewater source in the state is whey. However, whey can be re-used by mostly drying. For this reason, it is used again in ready-made food production (biscuit, chocolate, etc.) from being given as wastewater [ 8 ].
In the ice cream production unit, milk, additives, sugar and thickeners are mixed. After being pasteurised and cooled, aromas are added and packaged afterwards. Detergents and disinfectant-containing wastewaters form during cleaning and disinfection at the unit.
In condensed milk production, heated milk is evaporated and homogenised to yield sugar free milk. Sweet condensed milk is also produced using this method.
In the production of milk powder, it is obtained by applying vacuum evaporation and then spray drying.
The sources of the dairy industry wastewater are given in Table 1 .

The sources of the dairy industry wastewater [ 15 ].
Operating methods, production program, type of product being processed, water management being applied and design of the processing plant are effecting the composition and concentration of dairy effluents. Processing waters, cleaning wastewaters and sanitary wastewater are the three major sources of dairy industry wastewaters [ 9 ]. Most of milk processing industries use clean in place (CIP) system which uses caustic, phosphoric/nitric, sodium hypochlorite solutions for cleaning, and these chemicals became a part of wastewater [ 1 ].
Dairy industry wastewaters contain suspended and dissolved solids, soluble and trace organics, nutrients, fats, chlorides, sulphate, lactose, and they are characterised by high chemical oxygen demand (COD) and biological oxygen demand (BOD) [ 2 , 12 , 13 , 14 ]. The wastewater may also contain germicides, detergents and other types of chemicals [ 10 ]. These all have significant impact on wastewater. The characteristics and standards for discharge of dairy effluents are given in Table 2 .
Characteristics of some dairy industry wastewaters and discharge standards of dairy effluents (adapted from [ 2 , 14 ]).
The characteristics of dairy wastewaters have shown variable effluent composition and differ from industry to industry. This makes it hard to use same methods for each wastewater for treatment.
Traditional approaches (aerobic and anaerobic processes) for the treatment of dairy wastewater have many disadvantages such as land cost, climatic conditions, need of sludge recycling, and so on [ 7 ]. The most preferred treatment method for dairy wastewater is a biological method including processes such as activated sludge, tricking filters, aerated lagoons, sequential batch reactor (SBR), upflow anaerobic sludge blanket (UASB), anaerobic filters, and so on. Aerobic processes are high energy intensive, but they have to be combined with anaerobic processes to achieve discharge standards [ 2 , 16 ]. On the other hand, physicochemical methods are promising and effective methods for wastewater treatment.
2. Assessment of physicochemical treatment processes on dairy wastewater
Wastewater characterisation plays an important role when the wastewater treatment system is designed. The COD concentration of dairy wastewater varies considerably [ 23 ]. Pollution load of a company wastewater producing yogurt in the sector and pollution load of a company wastewater producing cheese are very different. Since yogurt and ayran production plants have low oil-grease and COD parameters, they generally provide only physical + biological treatment and discharge standards. However, since the oil-grease and KOI parameters are high in the cheese producing plants, the physical + chemical + biological treatment units are generally preferred in the small-scale plants.
In many countries, the wastewater of dairy and dairy products is among the sources that cause significant pollution of natural aquatic environments. Numerous studies have been conducted to date to considerably reduce the adverse effects of these wastewaters [ 24 ].
Physicochemical processes are widely used for treatment of industrial wastewaters. Summarised literature of the dairy industry wastewater treated with physico-chemical processes are given in Table 3 .
Summarised literature of the dairy industry wastewater treated with physico-chemical processes.
2.1. Chemical precipitation and coagulation/flocculation processes
Some physical-chemical-biological processes are usually interacting such as chemical precipitation, colloids’ aggregation by coagulation-flocculation processes. In most processes, both precipitation and coagulation-flocculation happen simultaneously.
Chemical precipitation involves the addition of chemicals to separate the dissolved and suspended solids by sedimentation and used for primary settling facilities. In current practice, phosphorus and heavy metal removal can be realised. Many substances have been used as precipitants over the years such as alum, ferric sulphate, ferrous sulphate, and so on. They are used primarily for the treatment of metallic cations, anions, organic molecules, detergents and oily emulsions [ 44 ].
Coagulation/flocculation processes are used basically to separate suspended, colloidal and dissolved contents from wastewater and they applied directly to raw wastewater [ 45 ]. The process can be divided into two categories. The first one named coagulation is the process where chemicals (coagulant agents) such as iron or aluminium are used to overcome the factors which promote the stability of the system. The second process named flocculation makes destabilised particles come together and they can be separated easily through gravity settling [ 46 ]. A few studies have been studied in the literature for the coagulation of dairy wastewater. The literature studies are summarised at Table 3 .
2.2. Adsorption process
Adsorption has been found to be attractive for the removal of organic compounds from wastewater [ 47 ]. There are many types of adsorbents including activated carbon, synthetic polymeric and silica-based adsorbents. The most useful one is activated carbon because of cost efficiency and ability to adsorb wide range of organic compounds. Adsorption can be classified as physical and chemical adsorption. Van der Waals forces are used in physical adsorption and activated carbon is the best example of physical adsorption. A chemical reaction occurs between adsorbate and adsorbent, but it does not have a wide application in wastewater treatment [ 48 ].
Adsorption onto solid surfaces has various applications and used to remove organics, chemicals, heavy metals, and so on [ 49 ]. Fly ash, rice husk ash, and bagasse fly ash and activated carbon are some of low-cost adsorbents.
2.3. Membrane processes
Membrane processes such as microfiltration, ultrafiltration, nanofiltration, dialysis, electrodialysis and reverse osmosis are very promising methods [ 49 ]. Membrane filtration can be defined as removal or separation of particulate and colloidal substances from a liquid which work as selective barrier and are typically 0.0001–1.0 μm.
Several works focused on treatment of dairy wastewater by membrane operations. The use of membrane filtration technology offers a wide range of advantages for the consumer. The membrane technology is a novel nonthermal environmental friendly technology within future possibilities that minimises the adverse effect of temperature rise such as change in phase, denaturation of proteins and change in sensory attributes of the product.

2.4. Electrochemical process
Electrolysis is the degradation of organic or inorganic substances by using electrical charge. Oxidation and reduction reactions occur in electrolytic cell which contains an anode and cathode. When you apply electric to cell, negative ions will migrate to anode and positive ions will migrate to cathode and cations will be reduced and anions will be oxidised at both electrodes [ 48 ]. Electrocoagulation, electroflotation and anodic oxidation processes are some examples used for dairy treatment.
Electrocoagulation is an effective and promising treatment method subject of numerous publications. It has been shown that this method is particularly effective for a wide range of pollutants (heavy metals, organic compounds, microorganisms and various others). For this reason, it is considered as one of the more promising water remediation techniques.
EC is a primary wastewater treatment for inducing the controlled electrogeneration of flocculants/coagulants on site, usually under the application of a constant current. It is a complex process involving several chemical and physical phenomena with the formation of iron or aluminium cations from the dissolution of the corresponding sacrificial anode(s) and the simultaneous production of OH− anions by cathodic reduction of water. The polymeric metal hydroxides formed act as excellent coagulating agents to favour the removal of dissolved, colloidal, or suspended matter, eventually yielding great percentages of removal of colour and turbidity. Coagulation mainly occurs by destabilisation, once the metal cations combine with the negatively charged particles moving towards the anode by electrophoretic motion [ 49 ].
3. Conclusions
Milk and dairy products are among the sources of industrial wastewater that cause significant pollution of natural aquatic environments. Wastewater generally comes from the dilution of milk or dairy products. In addition, detergents, disinfectant materials, machine oils and cloth fibres used in cleaning take place in wastewater. Dairy effluent nature is slightly alkaline, high temperature, unpleasant rancid odours, bitter or medicinal taste, hard, scaly deposits, and so on when it is disposed without treatments, it may result in adverse effects in fish growth, reproduction and immunity in water bodies, harmful effect on beneficial microorganism’s and plant growth due to decrease micronutrients solubility, serious problems of health and hygiene, eutrophication.
In order to treat industrial wastewater of milk and dairy products, quite different systems have been developed in different countries of the world. Factors such as the initial investment and operating costs in the selection of treatment technologies, the presence of appropriate staff for the enterprise and the need for treatment to ensure the regulations are taken into account.
The use of membrane technology in wastewater treatment by biological treatment has a short history covering the last 20–30 years. It is in a rapid development process, since it removes many disadvantages of classical systems. Membrane processes are in their process of being an effective remedy for most wastewater treatment with their unique properties. They can be used alone or together with other wastewater treatment systems. Membrane bioreactors offer effective solid-liquid separation, high yields of effluent, smaller plant sizes and low sludge production.
Treatment methods supported by chemical substances (coagulation-flocculation, oxidation-reduction, flotation, etc.) implemented for organic matter in water and wastewater treatment, solid material, turbidity, heavy metal, colour removal purposes. The treatment efficiency is affected by such factors such as the parameter to be eliminated, the chemical substance used, the duration of the detention, the intensity of the mixture; the amount of sludge formed can be more or less than the chemical substance. Compared to biological processes, advantages such as ease of operation, removal of the non-degradable part of the organic material, removal of the treatment efficiency from changes are caused to be particularly preferred.
- 1. Tikariha A, Omprakash S. Study of characteristics and treatments of dairy industry waste water. Journal of Applied & Environmental Microbiology. 2014; 2 (1):16-22. DOI: 10.12691/jaem-2-1-4
- 2. Kushwaha JP, Srivastana C, Mall ID. An overview of various technologies for the treatment of dairy wastewaters. Critical Reviews in Food Science and Nutrition. 2011; 51 :442-452. DOI: 10.1080/10408391003663879
- 3. The World Daıry Sıtuatıon. Bulletin of the International Dairy Federation 485/2016; 2016. ISSN 0250-5118
- 4. Sivrioğlu Ö, Yonar T. Determination of the acute toxicities of physicochemical pretreatment and advanced oxidation processes applied to dairy effluents on activated sludge. Journal of Dairy Science. 2015; 98 (4):2337-2344. DOI: 10.3168/jds.2014-8278
- 5. Singh NB, Singh R, Imam MM. Waste water management in dairy effluents: Pollution abatement and preventive attıtudes. International Journal of Science, Environment and Technology. 2014; 3 (2):672-683. ISSN: 2278-3687 (O)
- 6. Sarkar B, Chakrabari PP, Viyajkumar A, Kale V. Wastewater treatment in dairy industries-possibility of reuse. Desalination. 2006; 195 :141-152. DOI: 10.1016/j.desal.2005.11.015
- 7. Deshmukh DS. Wastewater generation and its treatment in dairy industries. International Journal of Application of Engineering and Technology. 2017; 2 (3):25-35. ISSN: 2321-8134
- 8. Kiliç A. Süt endüstrisi atıksularının arıtımında ardışık kesikli reaktörde (SBR) hareketli biofilm uygulaması [thesis]. Selçuk Üniversity; 2006
- 9. Britz TJ, Van Sckalkwyk C, Hung YT. Treatment of Dairy Processing Wastewater: Handbook of Industrial and Hazardous Waste Treatment. 2nd ed. New York: Marcel Dekker; 2004. pp. 616-646. DOI: 0-8493-7236-4
- 10. Shete BS, Shinkar NP. Dairy industry wastewater sources, characteristics & its effects on environment. International Journal of Current Engineering and Technology. 2013; 3 (5):1611-1615. ISSN: 2277-4106
- 11. Shivsharan VS, Kulkarni SW, Wani M. Physicochemical cahracterization of dairy effluents. International Journal of Life science and Pharma Research. 2013; 2 (2):182-191. ISSN: 2250-3137
- 12. Şengil A, Özacar M. Treatment of dairy wastewaters by electrocoagulation using mild steel electrodes. Journal of Hazardous Materials. 2006; B137 :1197-1205. ISSN: 2250-3137
- 13. Cristian O. Characteristics of the Untreated Wastewater Produced by Food Industry. Analele Universităţii din Oradea, Fascicula: Protecţia Mediului; 2010. p. XV
- 14. Deshannavar UB, Basavaraj RK, Naik NM. High rate digestion of dairy industry effluent by upflow anaerobic fixed-bed reactor. Journal of Chemical and Pharmaceutical Research. 2012; 4 (6):2895-2899. ISSN: 0975-7384
- 15. Tawfika A, Sobheyb M, Badawya M. Treatment of a combined dairy and domestic wastewater in an up-flow anaerobic sludge blanket (UASB) reactor followed by activated sludge (AS system). Desalination. 2008; 227 (1-3):167-177. DOI: 10.1016/j.desal.2007.06.023
- 16. Tawfika A, Sobheyb M, Badawya M. Treatment of a combined dairy and domestic wastewater in an up-flow anaerobic sludge blanket (UASB) reactor followed by activated sludge. Desalination. 2008; 227 (1-3):167-177. DOI: 10.1016/j.desal.2007.06.023
- 17. Qazi JI, Nadeem M, Baig SS, Baig S, Syed Q. Anaerobic fixed film biotreatment of dairy wastewater. Middle-East Journal of Scientific Research. 2011; 8 (3):590-593. ISSN: 1990-9233
- 18. Bulletin of the International Dairy Federation. The World Dairy Report No: 485/216; 2016
- 19. SKKY: Su Kirliligi Kontrol Yonetmeligi (Water Pollution and Control Regulation). T.C. Basbakanlik Mevzuati Gelistirme ve Yayin Genel Mudurlugu. 2004. p. 54. Available from: http://mevzuat.basbakanlik.gov.tr
- 20. Demirel B, Yenigün O, Onay TT. Anaerobic treatment of dairy wastewater: A review. Process Biochemistry. 2005; 40 :2583-2595. DOI: 10.1016/j.procbio.2004.12.015
- 21. Marshall KR, Harper WJ. Treatment of wastes from the dairy industry. In: Barnes D, Forster CF, Hrudey SE, editors. Surveys in Industrial Wastewater Treatment. Vol. 1. Boston: Pitman Advanced Publishing Program; 1984. pp. 296-376
- 22. Dabhi YM. Physicochemical treatment of dairy plant wastewater using ferrous sulphate and ferric chloride coagulants. International Journal of Basic and Applied Chemical Sciences. 2013; 3 (4):9-14
- 23. Karpati A, Bencze L, Boszeki J. New phisico-chemical pretreatment of dairy effluents. In: Proceedings of International Symposium on Waste Management Problems in Agro Industries; İstanbul. 1989. pp. 121-128
- 24. Lolei M, Alidadi H, Nekonam G, Kor Y. Study of the coagulation process in wastewater treatment of dairy industries. International Journal of Environmental Health Engineering. 2013; 2 (5):17-21. DOI: 10.4103/2277-9183.132684
- 25. Hamdani A, Chennaoui M, Assobhei O, Mountadar M. Dairy effluent characterization and treatment by coagulation decantation. Le Lait. 2004; 84 (3):317-328
- 26. Rusten B, Eikebrokk B, Thorvaldsen G. Coagulation as pretreatment of food industry wastewater. Water Science and Technology. 1990; 22 :1-8
- 27. Blanc F, Navia R. Treatment of dairy wastewater by chemical coagulation. In: Proceedings of the 45th Industrial Waste Conference; Pardue University, USA. pp. 681-689
- 28. Rao M, Bhole AG. Removal of organic matter from dairy industry wastewater using low-cost adsorbents. Journal of Indian Chemical Engineer. Section A. 2002; 44 (1):25-28
- 29. Kurzbaum E, Shalom OD. The potential of phosphate removal from dairy wastewater ad municipal wastewater effluents using a lanthanum modified bentonite. Applied Clay Science. 2016; 123 :182-186. DOI: 10.1016/j.clay.2016.01.038
- 30. Vourch M, Balannec B, Chaufer B, Dorange G. Treatment of dairy industry wastewater by reverse osmosis for water reuse. Desalination. 2008; 219 :190-202. DOI: 10.1016/j.desal.2007.05.013
- 31. Suarez A, Fidalgo T, Riera FA. Recovery of dairy wastewaters by reverse osmosis. Production of boiler water. Separation and Purification Technology. 2014; 133 :204-211. DOI: 10.1016/j.seppur.2014.06.041
- 32. Sarkar B, Chakrabarti PP, Vijaykumar A, Kale V. Wastewater treatment in dairy industries: Possibility of reuse. Desalination. 2005; 195 :141-152. DOI: 10.1016/j.desal.2005.11.015
- 33. Andrade LH, Mendes FDS, Espindola JC, Amaral MCS. Nanofiltration as tertiary treatment for the reuse of dairy wastewater treated by membrane bioreactor. Separation and Purification Technology. 2014; 126 :21-29. DOI: 10.1016/j.seppur.2014.01.056
- 34. Tchamango S, Njiki CPN, Ngameni E, Hadjiev D, Darchen A. Treatment of dairy effluents by electrocoagulation using aliminium electrodes. Science of the Total Environment. 2010; 408 :947-952. DOI: 10.1016/j.scitotenv.2009.10.026
- 35. Melchiors MS, Piovesan M, Becegato VR, Becegato VA, Tambourgi EB, Paulino AT. Treatment of wastewater from the dairy industry using electroflocculation and solid whey recovery. Journal of Environmental Management. 2016; 182 :574-580. DOI: 10.1016/j.jenvman.2016.08.022
- 36. Yavuz Y, Öcal E, Koparal AS, Öğütveren ÜB. Treatment of dairy industry wastewater by EC and EF processes using hybrid Fe-Al plate electrodes. Journal of Chemical Technology and Biotechnology. 2011; 86 (7):964-969. DOI: 10.1002/jctb.2607
- 37. Markou V, Kontogianni MC, Frontistis Z, Tekerlekopoulou AG, Katsonous A, Vayenas D. Electrochemical treatment of biologically pre-treated dairy wastewater using dimensionally stable anodes. Journal of Environmental Management. 2017; 202 :217-224. DOI: 10.1016/j.jenvman.2017.07.046
- 38. Yonar T, Sivrioğlu Ö. Electrochemical degradation of dairy effluent using novel Sn/Sb/Ni-Ti anodes. Journal of Physical Chemistry and Biophysics. 7 :2. In: 3rd International Conference on Electrochemistry; 10-11 July 2017; Berlin, Germany
- 39. Bazrafshan E, Moein H, Mostafapour FK, Nakhaie S. Application of electrocoagulation process for dairy wastewater treatment. Journal of Chemistry. 2013;8:Article ID 640139. DOI: 10.1155/2013/640139
- 40. Sharma D. Treatment of dairy waste water by electro coagulation using aluminum electrodes and settling, filtration studies. International Journal of ChemTech Research. 2014; 6 (1):591-599. ISSN: 0974-4290
- 41. Vaccari DA, Li Y, Shammas NK. Chemical precipitation. In: Wang LK, Hung YT, Shammas NK, editors. Phsysicochemical Treatment Processes. Totowa, New Jersey: Humana Press Inc.; 2005. pp. 141-174. DOI: 10.1016/j.watres.2010.07.001
- 42. Beneois K, Dahmani S, Berrebah K. Efficiency and limits of physicochemical treatment of dairy wastewater.(Case Study: Dairy industry in Western Algeria). International Journal of Chemical, Environmental and Biological Sciences. 2016; 4 (1):9-11. ISSN: 2320-4087
- 43. Tzoupanas ND, Zouboulis AI. Coagulation-Flocculation processes in waster/wastewater treatment: The application of new generation of chemical reagents. In: Proceedings of the 6th International Conference on Heat Transfer, Thermal Engineering and Envıronment (HTE'08); 20-22 Aug 2008; Greece
- 44. Aghili F, Ghoreyshi AA, Rahimpour A, Rahimnejad M. Enhanced treatment of pretreated sour whey by PAC adsorption/ membrane process. Chemical Engineering and Processing. 2016; 99 :80-85. DOI: 10.1016/j.cep.2015.11.006
- 45. Hung YT, Lo HH, Wang LK, Taricksa JR, Li KH. In: Wang LK, Hung YT, Shammas NK, editors. Granular activated carbon adsorption: Phsysicochemical treatment processes, Phsysicochemical Treatment Processes. Totowa, New Jersey: Humana Press Inc.; 2005. pp. 573-630. DOI: 10.1016/j.watres.2010.07.001
- 46. Al-Jabari M. Kinetic mass transfer adsorption model for treating dairy wastewater with stone cutting solid waste. Environmental Technology & Innovation. 2017; 7 :21-29. DOI: 10.1016/j.eti.2016.11.004
- 47. Vignesvaran S, Ngo HH, Chaudry DS, Hung YT. Physicochemical treatment processes for water reuse. In: Wang LK, Hung YT, Shammas NK, editors. Phsysicochemical Treatment Processes. Totowa, New Jersey: Humana Press Inc.; 2005. pp. 359-376. DOI: 10.1385/159259820x
- 48. Kuswaha JP, Srivastava VC, Mall ID. Organics removal from dairy wastewater by electrochemical treatment and residue disposal. Separation and Purification Technology. 2010; 76 :198-205. DOI: 10.1016/j.watres.2010.07.001
- 49. Chen JP, Chang SY, Hung YT. Electrolysis. In: Wang LK, Hung YT, Shammas NK, editors. Phsysicochemical Treatment Processes. Totowa, New Jersey: Humana Press Inc.; 2005. pp. 359-376. DOI: 10.1385/159259820x
© 2018 The Author(s). Licensee IntechOpen. This chapter is distributed under the terms of the Creative Commons Attribution 3.0 License , which permits unrestricted use, distribution, and reproduction in any medium, provided the original work is properly cited.
Continue reading from the same book
Published: 20 June 2018
By Nurcan Koca, Müge Urgu and Turkuaz Ecem Saatli
2507 downloads
By Qiming Chen, Liming Zhao, Lei Yao, Qianqian Chen, ...
2128 downloads
By Francesca Patrignani, Lorenzo Siroli, Diana I. Ser...
1241 downloads
Enhancing anaerobic digestion efficiency in dairy waste water treatment: a comprehensive review of enzyme-based pre-treatment by microorganisms in South Africa

- Article contents
- Figures & tables
- Supplementary Data
- Open the PDF for in another window
- Guest Access
- Cite Icon Cite
- Permissions
- Search Site
Hugendra Rishay Moodley , Laurah Gutu , Wasiu B. Ayinde , David Ikumi , Moses Basitere; Enhancing anaerobic digestion efficiency in dairy waste water treatment: a comprehensive review of enzyme-based pre-treatment by microorganisms in South Africa. Water Practice and Technology 2024; wpt2024095. doi: https://doi.org/10.2166/wpt.2024.095
Download citation file:
- Ris (Zotero)
- Reference Manager
The escalating global demand for dairy products due to population growth has led to increased production in the dairy industry, resulting in a significant rise in wastewater generation. This wastewater, laden with contaminants such as fats, oils, and greases (FOGs), biological oxygen demand (BOD), chemical oxygen demand (COD), nitrogen, and phosphorus, poses a threat to freshwater sources. Anaerobic digestion (AD) is considered the optimal treatment method for dairy wastewater, but the high-fat content poses challenges like reactor clogging. To overcome this, various authors propose and implement an enzymatic pre-treatment strategy that improves FOG and organic content removal, increases biogas production, and addresses economic and environmental concerns. Despite the proven efficacy of enzymatic pre-treatment, a significant drawback is the associated cost. However, it remains a promising strategy for enhancing the biodegradability of complex organic compounds in dairy effluents. This review delves into the crucial role of enzyme-producing microorganisms in enhancing AD efficiency for dairy wastewater treatment, emphasizing their potential benefits and addressing the economic and environmental considerations associated with this approach.
Enzymatic pre-treatment coupled with an AD produces a high-quality effluent.
Enzymatic pre-treatment is effective in the reduction of SS, FOGs in DWW but the cost implication makes it less appealing.
Recommendations have been offered for promising research areas in enzymatic pre-treatment with the use of new enzymes.
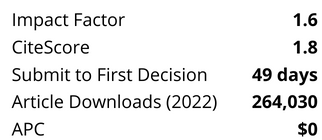
Affiliations
- EISSN 1751-231X
- Open Access
- Collections
- Subscriptions
- Subscribe to Open
- Editorial Services
- Rights and Permissions
- Sign Up for Our Mailing List
- IWA Publishing
- Republic – Export Building, Units 1.04 & 1.05
- 1 Clove Crescent
- London, E14 2BA, UK
- Telephone: +44 208 054 8208
- Fax: +44 207 654 5555
- IWAPublishing.com
- IWA-network.org
- IWA-connect.org
- Cookie Policy
- Terms & Conditions
- Get Adobe Acrobat Reader
- ©Copyright 2021 IWA Publishing
This Feature Is Available To Subscribers Only
Sign In or Create an Account
Academia.edu no longer supports Internet Explorer.
To browse Academia.edu and the wider internet faster and more securely, please take a few seconds to upgrade your browser .
Enter the email address you signed up with and we'll email you a reset link.
- We're Hiring!
- Help Center
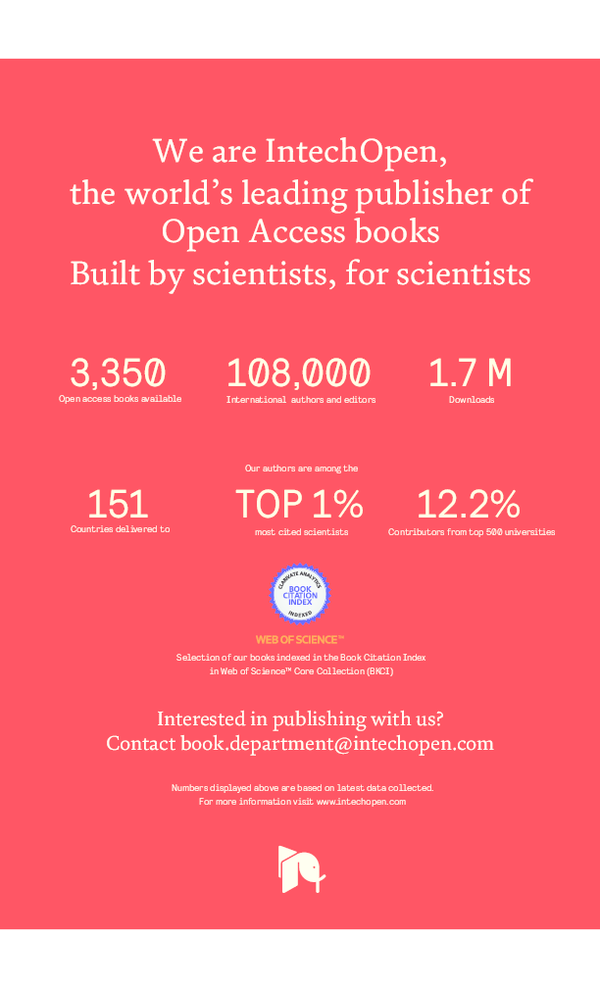
Physico-Chemical Treatment of Dairy Industry Wastewaters: A Review

Dairy industries have grown in most countries because of the demand in milk and milk products. This rise has led to the growth of dairy industries. The wastewaters discharged from this industry contain high concentrations of nutrients, chemical oxygen demand (COD), biological oxygen demand (BOD), total suspended solids (TSS) and organic and inorganic contents, which can cause serious environmental problems if not properly treated. The conventional biological treatment methods are suitable for dairy wastewaters due to its high biodegradability. However, long chain faty acids formed during the hydrolysis of lipids show the inhibitory action during anaerobic treatment. Sequencing batch reactor (SBR) and up low anaerobic sludge blanket (UASB) systems seem to be the most promising technology for the biological treatment of dairy waste-waters. Several research papers have been published on the application of aerobic and anaerobic treatment technologies for dairy industry wastewater, but both treatment methods still have some disadvantages. The most important challenge is to ind cost-eicient and environmentally sustainable approaches to enable water reuse and waste management. Therefore, alternative treatment technologies against biological treatment methods such as coagulation, adsorption, membrane and electrolysis processes are under investigation. This chapter provides a critical review focusing on physico-chemical treatment technologies of dairy wastewater.
Related Papers
DESALINATION AND WATER TREATMENT
JANET JOSHIBA
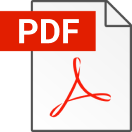
Reza Sanaye
Dairy industries have grown tremendously in many regions around the world due to the growth of demand for milk-related products. Dairy industries release wastewater containing high chemical oxygen demand (COD), biological oxygen demand (BOD), nutrients, in addition to organic and inorganic substances. Such wastewater, if improperly treated, severely pollutes water resources. For many years, anaerobic–aerobic processes have been used to remarkable effect in the treatment of dairy industry wastewater. Previously, a large portion of wastewater treatment was carried out in conventional anaerobic–aerobic treatment units. Nowadays, high-rate anaerobic–aerobic bioreactors are progressively employed for treating wastewater with high COD content. This paper reviews dairy wastewater sources, their production, and characteristics. Furthermore, different types of high-rate anaerobic–aerobic wastewater treatment methods currently available, including aerobic and anaerobic bioreactors over and ab...
Water Science & Technology
Environmental Management and Health
Process Biochemistry
Afindy Kadir
Denise Freire , Magali Cammarota
International Journal of Dairy Technology
Rogers Ribeiro
Journal of Food, Nutrition and Population Health
Romi Rangi , Gajanan Deshmukh
Dairy industry waste water is generally have fats, lactose, whey proteins, nutrients which lead play important role to increase the biological oxygen demand (BOD) of water. With milk components also wastewater contain detergents and sanitizing agents which are result of cleaning process increase the concentration of chemical oxygen demand (COD). There are many ways to reduce the BOD and COD, but biological treatment is the primary mean. Both aerobic and anaerobic technologies have been used, while anaerobic treatment of wastewater has emerged as viable and economical alternative over the conventional aerobic treatment particularly for high BOD. In aerobic technologies number of different treatment methods are there like, activated sludge, sequencing batch reactor, rotating biological contactors trickling filter. In anaerobic treatment methods are up flow sludge blanket (UASB), anaerobic sequencing batch reactors (ASBR), continuous-flow stirred tank reactor, hybrid anaerobic digesters, contact reactor, up flow and down flow anaerobic filter, and two-stage systems that separate the acid-forming and methane forming. There are many cases where aerobic and anaerobic processes are combined in one single treatment system. Membrane technology is alternatives to bio treatment being used for BOD reduction in dairy wastewaters which replaces secondary clarifiers in the waste treatment plants with membranes.
Biochemical Engineering Journal
Süleyman Uzuner
The dairy industry is generally considered to be the largest source of food processing wastewater in many countries. The highly variable nature of dairy wastewaters in terms of volumes and flowrates and in terms of high organic materials contents such as COD 921-9004 mg L −1 , BOD 483-6080 mg L −1 , TN of 8-230 mg L −1 and SS of 134-804 mg L −1 makes the choice of an effective wastewater treatment regime difficult. A high performance bioreactor, an aerobic jet loop reactor, combined with a ceramic membrane filtration unit, was used to investigate its suitability for the treatment of the dairy processing wastewater. The oxygen transfer rates of the bioreactor were found to be very high (100-285 h −1) on the operating conditions. A loading rate of 53 kg COD m −3 d −1 resulted in 97-98% COD removal efficiencies under 3 h hydraulic retention time. The high MLSS concentrations could be retained in the system (up to 38,000 mg L −1) with the contribution of UF (ultrafiltration) unit. During the filtration of activated sludge, the fluxes decreased with increasing MLSS. Cake formation fouling was determined as dominant fouling mechanisms. The results demonstrate that jet loop membrane bioreactor system was a suitable and effective treatment choice for treating dairy industry wastewater.
RELATED PAPERS
Warta Penelitian Perhubungan
sapto setyodhono
Neuroscience Letters
Peter Vaughan
EMILIOS SOLOMOU
Claudine Mary
Genetical Research
David Remington
Luis Alberto Mendez-Rosado
Journal of Biological Chemistry
David Westaway
Proceedings of International Structural Engineering and Construction
FESTUS OLUTOGE
Arquivos Brasileiros de Endocrinologia & Metabologia
Eliana Ferreira da Silva
AIChE Journal
Pramod Patil
International Journal of Pharmacy and Pharmaceutical Sciences
International Journal of Pharmacy and Pharmaceutical Sciences (IJPPS)
The Journal of Psychology
linh phương
Endocrine Practice
Mandalam Seshadri
Ville Ropponen
Applied Composite Materials
Rinze Benedictus
International Journal of Interactive Mobile Technologies (iJIM)
Adam Abubakar
magang bismilahajadulu
Evelyn Otero
kjgg fdfdgg
Sudheesh K Shukla, PhD
Journal of Feline Medicine and Surgery Open Reports
Mary Labato
Personality and Individual Differences
Peer Scheepers
FRANCISCO F A B I A N Y MOLINA BUSTOS
Saint-Augustin
Philippe Chenaux
RELATED TOPICS
- We're Hiring!
- Help Center
- Find new research papers in:
- Health Sciences
- Earth Sciences
- Cognitive Science
- Mathematics
- Computer Science
- Academia ©2024
Advertisement
Sustainability in the dairy industry: a systematic literature review
- Review Article
- Published: 21 June 2020
- Volume 27 , pages 33527–33542, ( 2020 )
Cite this article
- Alexandre André Feil ORCID: orcid.org/0000-0003-2217-3351 1 ,
- Dusan Schreiber 2 ,
- Claus Haetinger 3 ,
- Ângela Maria Haberkamp 4 ,
- Joice Inês Kist 1 ,
- Claudete Rempel 1 ,
- Alisson Eduardo Maehler 5 ,
- Mario Conill Gomes 5 &
- Gustavo Rodrigo da Silva 1
4918 Accesses
34 Citations
Explore all metrics
The dairy industry can contribute to global food security in a sustainable way by efficiently converting milk into dairy ingredients and products, even though they are polluting on a large scale. In this context, this study aimed to conduct a systematic literature review on sustainable indicators and dairy industries. The methodology used has a qualitative and quantitative approach and its technical procedure was the systematic literature review. The bases of journals consulted, using the keywords “sustainability indicator” and “dairy industry” which resulted in 130 valid scientific articles. The main results show that the sustainability indicators in the dairy industry are emerging and lacking research; being found seven papers, that highlight 12 indicators of the environmental, 11 of the social and eight economic dimensions, that may be considered fragile and initial. The studied problems are related to wastewater treatment methods, electric power consumption, efficiency of the industrial plant, among others, and the benefits on the theme are related to solutions to the difficulties, such as electricity reduction, sustainable practices. Among others, it is concluded that the dairy industries address the sustainability theme since 2011, with an ambiguous trend, being found evidence of the fragility of the sustainability indicators was found, mainly in the initial stage of their conception, when considering holistic approach (triple bottom line).
This is a preview of subscription content, log in via an institution to check access.
Access this article
Price includes VAT (Russian Federation)
Instant access to the full article PDF.
Rent this article via DeepDyve
Institutional subscriptions
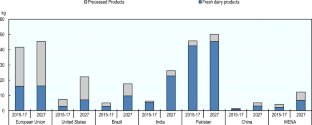
Similar content being viewed by others
The Sustainability Challenge of Dairy Livestock Systems
Sustainability in the Dairy Sector in Turkey: A Case Study Approach
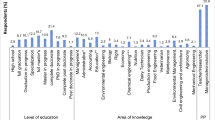
Set of sustainability indicators for the dairy industry
Alexandre André Feil, Caroline Constantin do Amaral, … Alisson Eduardo Maehler
Aguirre-Villegas HA, Milani FX, Kraatz S, Reinemann DJ (2012) Life cycle impact assessment and allocation methods development for cheese and whey processing. A Soc Agricul Biol Eng. https://doi.org/10.13031/2013.41363
Akoum O, Jaffrin MY, Ding LH, Frappart M (2004) Treatment of dairy process waters using a vibrating filtration system and NF and RO membranes. J Membr Sci 235:111–122
CAS Google Scholar
Alkhatim HS, Alcaina MI, Soriano E, Iborra MI, Lora J, Arnal J (1998) Poster: treatment of whey effluents from dairy industries by nanofiltration membranes. Desalination 119(1–3):177–183
Arbeli Z, Brenner A, Abeliovich A (2006) Treatment of high-strength dairy wastewater in an anaerobic deep reservoir: analysis of the methanogenic fermentation pathway and the rate-limiting step. Water Res 40(19):3653–3659
Audic JL, Chaufer B, Daufin G (2003) Non-food applications of milk components and dairy co-products: a review. Lait 83(6):417–438
Augustin MA, Udabage P, Juliano P, Clarke PT (2013) Towards a more sustainable dairy industry: integration across the farm–factory interface and the dairy factory of the future. Int Dairy J 31(1):2–11
Google Scholar
Aydiner C, Sen U, Topcu S, Ekinci D, Altinay AD, Koseoglu-Imer DY, Keskinler B (2014) Techno-economic viability of innovative membrane systems in water and mass recovery from dairy wastewater. J Membr Sci 458:66–75
Aydiner C, Sen U, Koseoglu-Imer DY, Dogan EC (2016) Hierarchical prioritization of innovative treatment systems for sustainable dairy wastewater management. J Clean Prod 112:4605–4617
Bai X, Ren X, Khanna NZ, Zhou N, Hu M (2018) Comprehensive water footprint assessment of the dairy industry chain based on ISO 14046: a case study in China. Resour Conserv Recycl 132:369–375
Balannec B, Gésan-Guiziou G, Chaufer B, Rabiller-Baudry M, Daufin G (2002) Treatment of dairy process waters by membrane operations for water reuse and milk constituents concentration. Desalination 147(1–3):89–94
Balannec B, Vourch M, Rabiller-Baudry M, Chaufer B (2005) Comparative study of different nanofiltration and reverse osmosis membranes for dairy effluent treatment by dead-end filtration. Sep Purif Technol 42(2):195–200
Barać Ž, Muminović S (2013) The impact of capital investments on dairy processing industry features: evidence from Slovenia, Croatia and Serbia. Mljekarstvo 63(3):140–149
Baran J (2013) Efficiency of the production scale of polish dairy companies based on data envelopment analysis. Oeconomia 12(2):5–13
Barford JP, Cail RG, Callander IJ, Floyd EJ (1986) Anaerobic digestion of high-strength cheese whey utilizing semicontinuous digesters and chemical flocculant addition. Biotechnol Bioeng 28(11):1601–1607
Barnes AP (2006) Does multi-functionality affect technical efficiency? A non-parametric analysis of the Scottish dairy industry. J Environ Manag 80:287–294
Barry JA (1982) Alcohol production from cheese whey. Dairy Indu Int 47(10):19–22
Baskaran K, Palmowski LM, Watson BM (2003) Wastewater reuse and treatment options for the dairy industry. Water Sci Technol Water Supply 3(3):85–91
Berlin J (2002) Environmental life cycle assessment (LCA) of Swedish semi-hard cheese. Int Dairy J 12(11):939–953
Berlin J, Sonesson U, Tillman AM (2007) A life cycle based method to minimise environmental impact of dairy production through product sequencing. J Clean Prod 15(4):347–356
Bhadouria BS, Sai VS (2011) Utilization and treatment of dairy effluent through biogas generation-a case study. Int J Environ Sci 1(7):1621
Blanc P, Goma G (1989) Propionic acid and biomass production using continuous ultrafiltration fermentation of whey. Biotechnol Lett 11(3):189–194
Blaskó B (2011) An analysis of the Hungarian dairy industry in the light of sustainability. Region Bus Stud 3(1):699–711
Blok V, Long TB, Gaziulusoy AI, Ciliz N, Lozano R, Huisingh D, Boks C (2015) From best practices to bridges for a more sustainable future: Advances and challenges in the transition to global sustainable production and consumption: Introduction to the ERSCP stream of the special volume. J Clean Prod 108:19–30
Boening PH, Larsen VF (1982) Anaerobic fluidized bed whey treatment. Biotechnol Bioeng 14:2539–2556
Boiral O, Guillaumie L, Heras-Saizarbitoria I, Tayo Tene CV (2018) Adoption and outcomes of ISO 14001: A systematic review. Int J Manag Rev 20(2):411–432
Bosco F, Chiampo F (2010) Production of polyhydroxyalcanoates (PHAs) using milk whey and dairy wastewater activated sludge: production of bioplastics using dairy residues. J Biosci Bioeng 109(4):418–421
Boulton AC, Rushton J, Wathes CM, Wathes DC (2011) Past trends and future challenges for a sustainable UK dairy industry. R Agric Soc England J 172:1–7
Bourlakis M, Maglaras G, Gallear D, Fotopoulos C (2014) Examining sustainability performance in the supply chain: the case of the Greek dairy sector. Ind Mark Manag 43(1):56–66
Brião VB, Granhen Tavares CR (2007) Effluent generation by the dairy industry: preventive attitudes and opportunities. Braz J Chem Eng 24(4):487–497
Brião VB, Salla ACV, Miorando T, Hemkemeier M, Favaretto DPC (2019) Water recovery from dairy rinse water by reverse osmosis: giving value to water and milk solids. Resour Conserv Recycl 140:313–323
Burgaud JL (1969) Les eaux résiduaires dans l'industrie laitière. Lait 49(487):417–433
Buys L, Mengersen K, Johnson S, van Buuren N, Chauvin A (2014) Creating a sustainability scorecard as a predictive tool for measuring the complex social, economic and environmental impacts of industries, a case study: assessing the viability and sustainability of the dairy industry. J Environ Manag 133:184–192
Carvalho A, Matos HA, Gani R (2013) SustainPro - A tool for systematic process analysis, generation and evaluation of sustainable design alternatives. Comput Chem Eng 50:8–27
Challis C, Tierney M, Todd A, Wilson E (2017) Human factors in dairy industry process control for energy reduction. J Clean Prod 168:1319–1334
Champagne CP, Goulet J, Lachance RA (1990) Production of bakers' yeast in cheese whey ultrafiltrate. Appl Environ Microbiol 56(2):425–430
Chmiel H, Mavrov V, Belieres E (2000) Reuse of vapour condensate from milk processing using nanofiltration. Filtration & separation 37(3):24–27
Cocci AA, Burke BF, Landine RC, Blickenstaff DL (1991) Anaerobic-aerobic pretreatment of a dairy waste; a case history. Dairy Food Environ Sanitation 11(9):505–509
Cox GC, Miller EJ (1986) Comparative energy efficiencies of the dairy manufacturing and processing industry: Australia and New Zealand. Engineering costs and production economics 10(4):293–303
Daneshi A, Esmaili-Sari A, Daneshi M, Baumann H (2014) Greenhouse gas emissions of packaged fluid milk production in Tehran. J Clean Prod 80:150–158
Daufin G, Escudier JP, Carrere H, Berot S, Fillaudeau L, Decloux M (2001) Recent and emerging applications of membrane processes in the food and dairy industry. Food Bioprod Process 79(2):89–102
Demirel B, Yenigun O, Onay TT (2005) Anaerobic treatment of dairy wastewaters: a review. Process Biochem 40(8):2583–2595
Djekic I, Smigic N, Glavan R, Miocinovic J, Tomasevic I (2018) Transportation sustainability index in dairy industry–fuzzy logic approach. J Clean Prod 180:107–115
Drescher K, Maurer O (1999) Competitiveness in the European dairy industries. Agribusiness: An International Journal 15(2):163–177
Eide MH (2002) Life cycle assessment (LCA) of industrial milk production. Int J Life Cycle Assess 7(2):115–126
Eide MH, Homleid JP, Mattsson B (2003) Life cycle assessment (LCA) of cleaning-in-place processes in dairies. LWT-Food Sci Technol 36(3):303–314
Fantin V, Buttol P, Pergreffi R, Masoni P (2012) Life cycle assessment of Italian high quality milk production. A comparison with an EPD study. J Clean Prod 28:150–159
Farizoglu B, Uzuner S (2011) The investigation of dairy industry wastewater treatment in a biological high performance membrane system. Biochem Eng J 57:46–54
Farizoglu B, Keskinler B, Yildiz E, Nuhoglu A (2004) Cheese whey treatment performance of an aerobic jet loop membrane bioreactor. Process Biochem 39(12):2283–2291
Feitz AJ, Lundie S, Dennien G, Morain M, Jones M (2007) Generation of an industry-specific physico-chemical allocation matrix. Application in the dairy industry and implications for systems analysis (9 pp). Int J Life Cycle Assess 12(2):109–117
Flysjö A, Thrane M, Hermansen JE (2014) Method to assess the carbon footprint at product level in the dairy industry. Int Dairy J 34(1):86–92
Frigon JC, Breton J, Bruneau T, Moletta R, Guiot SR (2009) The treatment of cheese whey wastewater by sequential anaerobic and aerobic steps in a single digester at pilot scale. Bioresour Technol 100(18):4156–4163
Gast J, Gundolf K, Cesinger B (2017) Doing business in a green way: a systematic review of the ecological sustainability entrepreneurship literature and future research directions. J Clean Prod 147:44–56
Gavala HN, Kopsinis H, Skiadas IV, Stamatelatou K, Lyberatos G (1999) Treatment of dairy wastewater using an upflow anaerobic sludge blanket reactor. J Agric Eng Res 73(1):59–63
Genç S, Yıldırım N (2017) Sustainable dairy industry by using renewable energy source. Celal Bayar Üniversitesi Fen Bilimleri Dergisi 13(3):665–670
Glover JL, Champion D, Daniels KJ, Dainty AJD (2014) An institutional theory perspective on sustainable practices across the dairy supply chain. Int J Prod Econ 152:102–111
Gonçalves NP, Maderi TR, Santos PF (2017) Avaliação das práticas ambientais em indústrias de laticínios–estudo de caso. Periódico Eletrônico Fórum Ambiental da Alta Paulista 13(2):66–77
González Siso MI (1996) The biotechnological utilization of cheese whey: a review. Bioresour Technol 57(1):1–11
González-García S, Castanheira ÉG, Dias AC, Arroja L (2013a) Using life cycle assessment methodology to assess UHT milk production in Portugal. Sci Total Environ 442:225–234
González-García S, Castanheira ÉG, Dias AC, Arroja L (2013b) Environmental life cycle assessment of a dairy product: the yoghurt. Int J Life Cycle Assess 18(4):796–811
Grochowska R, Szczepaniak I (2019) Sustainability business models in milk processing. Considerations based on the polish experience. J Agribus Rural Dev 52(2):111–122
Haast J, Britz TJ, Novello JC, Verwey EW (1985) Anaerobic digestion of deproteinated cheese whey. J Dairy Res 52:457–467
Houldsworth DW (1980) Demineralization of whey by means of ion exchange and electrodialysis. Int J Dairy Technol 33(2):45–51
Huang J, Xu CC, Ridoutt BG, Liu JJ, Zhang HL, Chen F, Li Y (2014) Water availability footprint of milk and milk products from large-scale dairy production systems in Northeast China. J Clean Prod 79:91–97
Hwang DC, Damodaran S (1995) Selective precipitation and removal of lipids from cheese whey using chitosan. J Agric Food Chem 43(1):33–37
IDF International Dairy Federation. (2018). Bulletin of the IDF N° 494/ 2018: The World Dairy Situation. https://store.fil-idf.org/wp-content/uploads/2018/10/WDS2018Preview-1.pdf . Accessed 05 may 2019
Irfan ZB, Mondal M (2016) Water footprint analysis in dairy industry in India. Int J Environ Sci Dev 7(8):591–594
Jalali S, Wohlin C (2012) Systematic literature studies: database searches vs. backward snowballing In Proceedings of the ACM-IEEE international symposium on Empirical software engineering and measurement. ESEM 12:29–38
Jokandan MJ, Aghbashlo M, Mohtasebi SS (2015) Comprehensive exergy analysis of an industrial-scale yogurt production plant. Energy 93:1832–1851
Kalyuzhnyi SV, Martinez EP, Martinez JR (1997) Anaerobic treatment of high-strength cheese-whey wastewaters in laboratory and pilot UASB-reactors. Bioresour Technol 60(1):59–65
Kasmi M (2018) Biological processes as promoting way for both treatment and valorization of dairy industry effluents. Waste Biomass Valorization 9(2):195–209
Kim D, Thoma G, Nutter D, Milani F, Ulrich R, Norris G (2013) Life cycle assessment of cheese and whey production in the USA. Int J Life Cycle Assess 18(5):1019–1035
Kosikowski FV (1979) Whey utilization and whey products. J Dairy Sci 62:1149–1160
Kothari R, Kumar V, Pathak VV, Tyagi VV (2017) Sequential hydrogen and methane production with simultaneous treatment of dairy industry wastewater: bioenergy profit approach. Int J Hydrog Energy 42(8):4870–4879
Koyuncu I, Turan M, Topacik D, Ates A (2000) Application of low pressure nanofiltration membranes for the recovery and reuse of dairy industry effluents. Water Sci Technol 41(1):213–221
Labbé JI, Ramos-Suárez JL, Hernández-Pérez A, Baeza A, Hansen F (2017) Microalgae growth in polluted effluents from the dairy industry for biomass production and phytoremediation. J Environ Chem Eng 5(1):635–643
Lhanafi S, Anfar Z, El Alem NE, Chebli B, Benafqir M (2020) Methanisation: a promising green technology to manage organic wastes in the Moroccan dairy industry. Mater Today: Proceedings 22:57–60
Li Y, Mathiyazhagan K (2018) Application of DEMATEL approach to identify the influential indicators towards sustainable supply chain adoption in the auto components manufacturing sector. J Clean Prod 172:2931–2941
Lima LP, Ribeiro GB, Perez R (2018) The energy mix and energy efficiency analysis for Brazilian dairy industry. J Clean Prod 181:209–216
Loubère L, Ratinaud P (2013). Documentation IRaMuTeQ 0.6 alpha 3 version 0.1. 2013. http://www.iramuteq.org/documentation/fichiers/documentation_iramuteq_21_12_2013.pdf . Accessed 01 may 2019
Luo J, Ding L, Qi B, Jaffrin MY, Wan Y (2011) A two-stage ultrafiltration and nanofiltration process for recycling dairy wastewater. Bioresour Technol 102(16):7437–7442
Luthra S, Govindan K, Kannan D, Mangla SK, Garg CP (2017) An integrated framework for sustainable supplier selection and evaluation in supply chains. J Clean Prod 140:1686–1698
Malaspina F, Stante L, Cellamare CM, Tilche A (1995) Cheese whey and cheese factory wastewater treatment with a biological anaerobic - aerobic process. Water Sci Technol 32(12):59–72
Malaspina F, Cellamare CM, Stante L, Tilche A (1996) Anaerobic treatment of cheese whey with a downflow-upflow hybrid reactor. Bioresour Technol 55(2):131–139
Méndez R, Blazquez R, Lorenzo F, Lema JM (1989) Anaerobic treatment of cheese whey: start-up and operation. Water Sci Technol 21(12):1857–1860
Meneses YE, Flores RA (2016) Feasibility, safety, and economic implications of whey-recovered water in cleaning-in-place systems: a case study on water conservation for the dairy industry. J Dairy Sci 99(5):3396–3407
Miller EJ (1984) Energy use in the New Zealand dairy processing industry. Energy in agriculture 3:307–312
Mockaitis G, Ratusznei SM, Rodrigues JA, Zaiat M, Foresti E (2006) Anaerobic whey treatment by a stirred sequencing batch reactor (ASBR): effects of organic loading and supplemented alkalinity. J Environ Manag 79(2):198–206
Monroy O, Vazquez F, Derramadero JC, Guyot JP (1995) Anaerobic-aerobic treatment of cheese wastewater with national technology in Mexico: the case of “El Sauz”. Water Sci Technol 32(12):149–156
Muangrat R, Onwudili JA, Williams PT (2011) Alkaline subcritical water gasification of dairy industry waste (whey). Bioresour Technol 102(10):6331–6335
Muehlhoff E, Bennett A, McMahon D (2013) Milk and dairy products in human nutrition. Food and Agriculture Organization of the United Nations (FAO), Rome
Mukhopadhyay R, Talukdar D, Chatterjee BP, Guha AK (2003) Whey processing with chitosan and isolation of lactose. Process Biochem 39(3):381–385
Munir MT, Yu W, Young BR (2014) Can exergy be a useful tool for the dairy industry? Comp Aided Chem Eng 33:1129–1134
Nanda S, Reddy SN, Hunter HN, Butler IS, Kozinski JA (2015) Supercritical water gasification of lactose as a model compound for valorization of dairy industry effluents. Ind Eng Chem Res 54(38):9296–9306
Nguyen MH, Durham RJ (2004) Status and prospects for cleaner production in the dairy food industry. Aust J Dairy Technol 59(2):171
Nisa, S. (2017). Sustainable business model in dairy sector. Int J Manag Res, 5(5)
OECD/FAO (2018) OECD-FAO Agricultural Outlook 2018–2027, OECD Publishing. Paris/Food and Agriculture Organization of the United Nations, Rome. https://doi.org/10.1787/agr_outlook-2018-en Accessed 17 may 2019
Book Google Scholar
Orhon D, Görgün E, Germirli F, Artan N (1993) Biological treatability of dairy wastewaters. Water Res 27(4):625–633
Özbay A, Demirer GN (2007) Cleaner production opportunity assessment for a milk processing facility. J Environ Manag 84(4):484–493
Öztürk I, Eroglu V, Ubay G, Demir I (1993) Hybrid upflow anaerobic sludge blanket reactor (HUASBR) treatment of dairy effluents. Water Sci Technol 28(2):77–85
Papoutsopoulou SV, Ekateriniadou LV, Kyriakidis DA (1994) Genetic construction of Xanthomonas campestris and xanthan gum production from whey. Biotechnol Lett 16(12):1235–1240
Pappa I, Illiopoulos C, Massouras T (2019) On sustainability of a dairy sector in crisis. Int J Food Syst Dyn 10(2):130–150
Passeggi M, López I, Borzacconi L (2012) Modified UASB reactor for dairy industry wastewater: performance indicators and comparison with the traditional approach. J Clean Prod 26:90–94
Place SE, Mitloehner FM (2010) Invited review: contemporary environmental issues: a review of the dairy industry's role in climate change and air quality and the potential of mitigation through improved production efficiency. J Dairy Sci 93(8):3407–3416
Popović R, Panić D (2018) Technical efficiency of Serbian dairy processing industry. Econ Agric 65(2):569–581
Prazeres AR, Carvalho F, Rivas J (2012) Cheese whey management: a review. J Environ Manag 110:48–68
Quijera JA, Labidi J (2013) Pinch and exergy based thermosolar integration in a dairy process. Appl Therm Eng 50(1):464–474
Quijera JA, Alriols MG, Labidi J (2011) Integration of a solar thermal system in a dairy process. Renew Energy 36(6):1843–1853
Rad SJ, Lewis MJ (2014) Water utilisation, energy utilisation and waste water management in the dairy industry: a review. Int J Dairy Technol 67(1):1–20
Radha K, Thomas A, Sathian CT (2014) Application of nano technology in dairy industry: prospects and challenges-a review. Indian J Dairy Sci 67(5):367–374
Rafiee S, Khoshnevisan B, Mohammadi I, Aghbashlo M, Clark S (2016) Sustainability evaluation of pasteurized milk production with a life cycle assessment approach: an Iranian case study. Sci Total Environ 562:614–627
Ramirez CA, Patel M, Blok K (2006) From fluid milk to milk powder: energy use and energy efficiency in the European dairy industry. Energy 31(12):1984–2004
Rao M, Bhole AG (2002) Removal of organic matter from dairy industry wastewater using low-cost adsorbents. Indian Chemical Engineer 44(1):25–28
Riera FA, Suárez A, Muro C (2013) Nanofiltration of UHT flash cooler condensates from a dairy factory: characterisation and water reuse potential. Desalination 309:52–63
Salzman S, Scarborough H, Allinson G (2017) Exploring the economic viability of using constructed wetlands to manage waste-water in the dairy industry. Austr J Environ Manag 24(3):276–288
Sampaio RE, Mancini MC (2007) Estudos de revisão sistemática: um guia para a síntese criteriosa da evidência científica. Rev Bras Fis 11(1):83–89
Santos HCM, Maranduba HL, Almeida Neto JA, Rodrigues LB (2017) Life cycle assessment of cheese production process in a small-sized dairy industry in Brazil. Environ Sci Pollut Res 24(4):3470–3482
Sarkar B, Chakrabarti PP, Vijaykumar A, Kale V (2006) Wastewater treatment in dairy industries-possibility of reuse. Desalination 195(1–3):141–152
Satolo EG, Campos RSD, Ussuna GDA, Simon AT, Mac-Lean PAB, Braga Júnior SS (2020) Sustainability assessment of logistics activities in a dairy: an example of an emerging economy. Production 30
Schütz, R. E. (2018). O Inglês como idioma internacional. Inglês Made in Brazil. http://www.sk.com.br/sk-ingl.html . Accessed 05 may 2019
Severino AJ (2007) Metodologia do Trabalho Científico, 23rd edn. Cortez, São Paulo
Sharma VK, Chandana P, Bhardwaj A (2015) Critical factors analysis and its ranking for implementation of GSCM in Indian dairy industry. J Manuf Technol Manag 26(6):911–922
Sharma VK, Chandna P, Bhardwaj A (2017) Green supply chain management related performance indicators in agro industry: a review. J Clean Prod 141(1):1194–1208
Sharma AK, Sharma C, Mullick SC, Kandpal TC (2018) Financial viability of solar industrial process heating and cost of carbon mitigation: a case of dairy industry in India. Sustain Energy Technol Assess 27:1–8
Shay LK, Wegner GH (1986) Nonpolluting conversion of whey permeate to food yeast protein. J Dairy Sci 69(3):676–683
Singh G, Tyagi VV, Singh PJ, Pandey AK (2020) Estimation of thermodynamic characteristics for comprehensive dairy food processing plant: an energetic and exergetic approach. Energy 194:116799
Soboh R, Lansink A, Dijk G (2014) Efficiency of European dairy processing firms. Wageningen J Live Sci 70-71:53–59
Sorgüven E, Özilgen M (2012) Energy utilization, carbon dioxide emission, and exergy loss in flavored yogurt production process. Energy 40(1):214–225
Soufiyan MM, Aghbashlo M, Mobli H (2017) Exergetic performance assessment of a long-life milk processing plant: a comprehensive survey. J Clean Prod 140:590–607
Switzenbaum MS, Danskin SC (1982) Anaerobic expanded bed treatment of whey. Agricultural Wastes 4(6):411–426
Thoma G, Popp J, Nutter D, Shonnard D, Ulrich R, Matlock M, Adom F (2013) Greenhouse gas emissions from milk production and consumption in the United States: a cradle-to-grave life cycle assessment circa 2008. Int Dairy J 31:S3–S14
Thongplew N, van Koppen CK, Spaargaren G (2016) Transformation of the dairy industry toward sustainability: the case of the organic dairy industries in the Netherlands and Thailand. Environ Dev 17:6–20
Tiwari S, Behera CR, Srinivasan B (2016) Simulation and experimental studies to enhance water reuse and reclamation in India’s largest dairy industry. J Environ Chem Eng 4(1):605–616
Tonelli F, Evans S, Taticchi P (2013) Industrial sustainability: challenges, perspectives, actions. Int J Bus Innov Res 7(2):143c163
Torres López ER, Doval Leira R, Galera MM, Bello Bugallo PM (2017) Integrated environmental permit through best available techniques: evaluation of the dairy industry. J Clean Prod 162:512–528
Üçtuğ FG (2019) The environmental life cycle assessment of dairy products. Food Eng Rev:1–18
Van den Berg L, Kennedy KJ (1983) Dairy waste treatment with anaerobic stationary fixed film reactors. Water Sci Technol 15(8–9):359–368
Van der Horst HC, Timmer JMK, Robbertsen T, Leenders J (1995) Use of nanofiltration for concentration and demineralization in the dairy industry: model for mass transport. J Membr Sci 104(3):205–218
Veleva V, Ellenbecker M (2001) Indicators of sustainable production: framework and methodology. J Clean Prod 9(6):519–549
Venkatraman K, Achi M (2004) To eliminate the disposal of salty whey from a dairy industry into the sewer in an environmental, social and economical way–a case study from dairy farmers, Toowoomba, Queensland, Australia. Int J Environ Technol Manag 4(4):365–374
Vlontzos G, Theodoridis A (2013) Efficiency and productivity change in the Greek dairy industry. Agric Econ Rev 14(389-2016-23494):14–28
Von Keyserlingk MAG, Martin NP, Kebreab E, Knowlton KF, Grant RJ, Stephenson M, Smith SI (2013) Invited review: sustainability of the US dairy industry. J Dairy Sci 96(9):5405–5425
Vourch M, Balannec B, Chaufer B, Dorange G (2005) Nanofiltration and reverse osmosis of model process waters from the dairy industry to produce water for reuse. Desalination 172(3):245–256
Vourch M, Balannec B, Chaufer B, Dorange G (2008a) Treatment of dairy industry wastewater by reverse osmosis for water reuse. Desalination 219(1–3):190–202
Vourch M, Balannec B, Chaufer B, Dorange G (2008b) Treatment of dairy industry wastewater by reverse osmosis for water reuse. Desalination 219(1–3):190–202
Xu T, Flapper J, Kramer KJ (2009) Characterization of energy use and performance of global cheese processing. Energy 34(11):1993–2000
Yan JQ, Lo KV, Liao PH (1989) Anaerobic digestion of cheese whey using up-flow anaerobic sludge blanket reactor. Biol Wastes 27(4):289–305
Yildirim N, Genc S (2017) Energy and exergy analysis of a milk powder production system. Energy Convers Manag 149:698–705
Yorgun MS, Balcioglu IA, Saygin O (2008) Performance comparison of ultrafiltration, nanofiltration and reverse osmosis on whey treatment. Desalination 229(1–3):204–216
Zall RR (1984) Trends in whey fractionation and utilization, a global perspective. J Dairy Sci 67(11):2621–2629
Download references
Author information
Authors and affiliations.
Universidade do Vale do Taquari – Univates, Lajeado, Rio Grande do Sul, Brazil
Alexandre André Feil, Joice Inês Kist, Claudete Rempel & Gustavo Rodrigo da Silva
Universidade Feevale, Novo Hamburgo, Rio Grande do Sul, Brazil
Dusan Schreiber
Instituto Federal de Educação, Ciência e Tecnologia do Rio Grande do Sul, campus Caxias do Sul, Caxias do Sul, RS, Brazil
Claus Haetinger
Ângela Maria Haberkamp
Universidade Federal de Pelotas (UFPEL), Pelotas, Rio Grande do Sul, Brazil
Alisson Eduardo Maehler & Mario Conill Gomes
You can also search for this author in PubMed Google Scholar
Corresponding author
Correspondence to Alexandre André Feil .
Additional information
Responsible editor: Philippe Garrigues
Publisher’s note
Springer Nature remains neutral with regard to jurisdictional claims in published maps and institutional affiliations.
Rights and permissions
Reprints and permissions
About this article
Feil, A.A., Schreiber, D., Haetinger, C. et al. Sustainability in the dairy industry: a systematic literature review. Environ Sci Pollut Res 27 , 33527–33542 (2020). https://doi.org/10.1007/s11356-020-09316-9
Download citation
Received : 18 November 2019
Accepted : 14 May 2020
Published : 21 June 2020
Issue Date : September 2020
DOI : https://doi.org/10.1007/s11356-020-09316-9
Share this article
Anyone you share the following link with will be able to read this content:
Sorry, a shareable link is not currently available for this article.
Provided by the Springer Nature SharedIt content-sharing initiative
- Sustainability
- Dairy industry
- Cleaner production
- Environmental science
- Find a journal
- Publish with us
- Track your research
ISSN: 2663-6859
Editor-in-Chief
Frank Gardner
Editorial Board
JEIL Editorial Office
4246 Albert Street, Suite 413
Regina, Sask S4S 3R9
Phone: (306)337-2306
Fax: (306)337-2305
Scopus Google Scholar EBSCO Scopus ProQuest EBSCO Environmental Engineering Abstracts International Abstracts in Operations Research Pollution Abstracts Sustainability Science Abstracts Water Resources Abstract ZETOC Microsoft Academic Search
- Current Issue
- Back Issues
- Alerting Service
- Subscription
- Recommendation
- Instructions
- Manuscript Submission
- Private Policy
- Copyright Form
- Reprint Order Form
- Special Issue
- Publication Ethics
- Guidelines for Reviewers
- For Associate Editors
- Review Form
- Permission Request
- About ISEIS
- articles in press
Dairy Wastewater Treatment through Synergies of the Biological and Hybrid Membrane: A Systematic Review
- Department of Industrial Engineering, College of Engineering, Debre Berhan University, Debre Berhan, Ethiopia
Keywords : aerobic, anaerobic, dairy wastewater, physico-chemical treatment, pre-hydrolysis, pre- and post-treatment
Supplementary Files:
- There are currently no refbacks.

IMAGES
VIDEO
COMMENTS
Also some other methods like Biological treatment & Reverse Osmosis process can be also useful for the treatment of the Dairy Wastewater. 1.1. Literature survey1.1.1. Sources of wastewater. Dairy wastewater and liquid waste arise from different sections of the dairy industry like, cheese and butter plants, ice cream plants, and condensed milk ...
A crucial requirement for biological treatment of dairy wastewater is their pH value between 6 and 9 ( 37 ). Milk and butter factories have effluents with active reaction close to neutral (pH=6.8-7.4). In plants where a certain amount of whey is discharged, the pH of the effluent is reduced to below 6.2.
Dairy Wastewaters - General Characte ristics and Treatment Possibilities -. A Review. Aleksandar Kolev Slavov. University of Food Technologies, Faculty of Economics, Department of ...
In this literature review, wastewater characterizations obtained from different sampling points within a dairy wastewater treatment plant (WWTP) were also found. Wastewater was categorized as shown in Fig. 5 and its characteristics have also been evaluated herein. Download : Download high-res image (132KB) Download : Download full-size image ...
Abstract Dairy industry is one of the leading consumer of water and producer of wastewater. Increased demand of dairy products results in rapid growth of dairy industry and hence wastewater production increases. Dairy effluent contains high concentrations of organic and inorganic substances that cause eutrophication in water bodies. This review study emphasizes on various treatment methods of ...
This article uses a systematic literature review method to review and examine other research findings. ... the most promising techniques for dairy wastewater treatment are combinations of ...
Water pollution caused by population growth and human activities is a critical problem exacerbated by limited freshwater resources and increasing water demands. Various sectors contribute to water pollution, with the dairy industry being a significant contributor due to the high concentrations of harmful contaminants in dairy wastewater. Traditional treatment methods have been employed, but ...
This review study emphasizes on various treatment methods of DWW for period 2002 to 2022. Dairy wastewater imposes serious environmental concern because of the presence of wide range of fatty acids, proteins, nutrients, and other organics. These contaminants are difficult to treat in single step conventional treatment technique. In recent years ...
The global dairy sector produces upwards of 900 million tonnes of milk each year, resulting in 2.25 billion tonnes of wastewater being generated. This is significant in the South African context as a large percentage of the population lives without reliable access to clean, reliable water. The treatment of dairy wastewater (DWW) in this context would help alleviate the unavailability for many ...
This review aims to analyze phytoremediation as a sustainable alternative for dairy wastewater treatment. It initially briefs about dairy wastewater characteristics and treatment alternatives and discusses constructed wetlands and hydroponic system in detail with mechanism insights and influenced process parameters.
Summarised literature of the dairy industry wastewater treated with physico-chemical processes are given in Table 3. Treatment process Characterisation ... Anaerobic treatment of dairy wastewater: A review. Process Biochemistry. 2005; 40:2583-2595. DOI: 10.1016/j.procbio.2004.12.015; 21. Marshall KR, Harper WJ. Treatment of wastes from the ...
It is estimated that about 0.2-10 L of wastewater with an average value of 2.5 L is generated per liter of milk processed, therefore, wastewater treatment is of huge concern in these industries. The study shall review the literature available on wastewater treatment technologies used in dairy industry and predict areas for future studies.
Dairy Wastewater Aerobic Treatment Proc ess. Dairy waste water contains mainly solids, B OD, ammonia, nitrite, nitr ate, milk protein, fat, amino acid, urea, uric acids, triv-. ial nitroge n ...
However, it remains a promising strategy for enhancing the biodegradability of complex organic compounds in dairy effluents. This review delves into the crucial role of enzyme-producing microorganisms in enhancing AD efficiency for dairy wastewater treatment, emphasizing their potential benefits and addressing the economic and environmental ...
This is one of the most effective membrane-cleaning procedures reported in the literature [38, 39]. For comparison, a set of experiments was also conducted without GAC fluidization for 56 days. ... Methanogenic treatment of dairy wastewater: a review of current obstacles and new technological perspectives. Sci. Total Environ., 866 (2023), ...
Increased demand of dairy products results in rapid growth of dairy industry and hence wastewater production increases. Dairy effluent contains high concentrations of organic and inorganic substances that cause eutrophication in water bodies. This review study emphasizes on various treatment methods of DWW for period 2002 to 2021.
The dairy industry is generally considered to be the largest source of food processing wastewater in many countries. The highly variable nature of dairy wastewaters in terms of volumes and flowrates and in terms of high organic materials contents such as COD 921-9004 mg L −1 , BOD 483-6080 mg L −1 , TN of 8-230 mg L −1 and SS of 134-804 mg L −1 makes the choice of an effective ...
Sequencing batch reactor is the most preferred technology for the treatment of dairy waste water. In this technology dairy wastewater is added in a single batch reactor, and treated for the ...
Among the food industries, dairy industry is most polluting in regards to its large water consumption because water has been a key processing medium. Environmental protection agencies have started a strict vigil for banning of wastewater discharge into the natural resources of water. This has made the water treatment more expensive thereby becoming a huge burden for the industries. Thus, water ...
Dairy and soy products are two of the most common sources of protein in the human diet. Both industries produce large volumes of wastewater (up to 10 L L −1 raw material), high treatment costs as high as 130 and 3374 US$/meter cubic of effluent treated for soy and dairy, respectively. The biological oxygen demand reaches 6.8 and 48 g L −1, while the chemical oxygen demand reaches 12 and 95 ...
The dairy industry can contribute to global food security in a sustainable way by efficiently converting milk into dairy ingredients and products, even though they are polluting on a large scale. In this context, this study aimed to conduct a systematic literature review on sustainable indicators and dairy industries. The methodology used has a qualitative and quantitative approach and its ...
This article uses a systematic literature review method to review and examine other research findings. The results indicate that despite extensive studies on pre- and post-treatment techniques, both have limitations. ... the most promising techniques for dairy wastewater treatment are combinations of adsorption-aerobic and enzymatic hydrolysis ...
The use of decentralized wastewater treatment technologies is a reasonable solution for rural areas. As a decentralized treatment technology, the multi-soil-layering (MSL) system has recently drawn an increasing amount of attention owing to its merits, such as a high hydraulic load rate, small land area occupation, low probability of clogging, low investment, and low operation cost.
This review aims at providing comprehensive overview of the profiling of livestock wastewater from the dairy, swine and poultry sub-sectors along with the biological, physico-chemical, AI-based and integrated treatment methodologies, and valorisation for the generation of value-added products such as bioplastics, biofertilizers, biohydrogen and ...