Thank you for visiting nature.com. You are using a browser version with limited support for CSS. To obtain the best experience, we recommend you use a more up to date browser (or turn off compatibility mode in Internet Explorer). In the meantime, to ensure continued support, we are displaying the site without styles and JavaScript.
- View all journals
- Explore content
- About the journal
- Publish with us
- Sign up for alerts
- 19 June 2024

Human neuroscience is entering a new era — it mustn’t forget its human dimension
You have full access to this article via your institution.
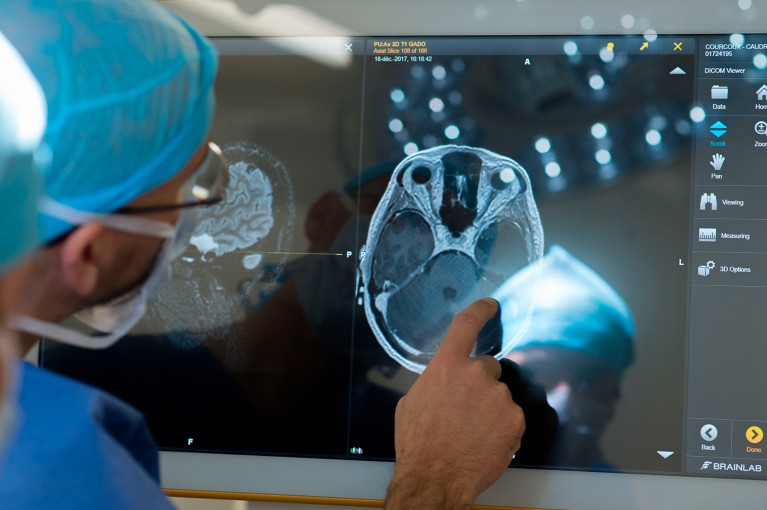
Studies involving people who are awake during brain surgery are helping to explain how the brain produces and perceives speech. Credit: BSIP/Universal Images Group/Getty
In neuroscience, ‘Broca’s area’ is a well-known part of the brain that is crucial for speech production. It is named after the nineteenth-century physician-researcher who discovered it — Paul Broca. Less well known, however, is the person whose brain enabled Broca to do so. His name was Louis Victor Leborgne and he had lost his ability to speak at age 30.
Leborgne’s story reminds us why we must never ignore the people involved, assume they’ve consented or fail to acknowledge them appropriately — especially in an age when a lot of neuroscientific research involves humans.
This week’s issue of Nature includes several studies devoted to human neuroscience. They highlight the opportunities researchers have to study the human brain in never-before-seen detail. For example, single-neuron recordings of people who are awake while undergoing brain surgery are helping to explain how the brain produces and perceives speech . Similarly, atlases of brain-cell types, neural circuits and gene-expression maps have the potential to revolutionize our understanding of the cellular and molecular processes that underline behaviour and cognition .
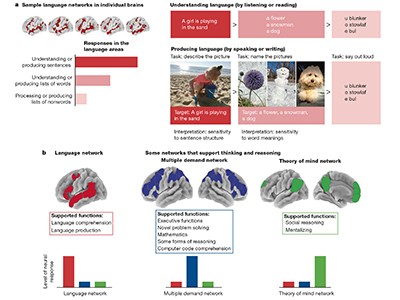
Read the paper: Language is primarily a tool for communication rather than thought
These technologies are helping researchers to explore what sets the human brain apart from those of other species, and how its cognitive abilities have evolved. For example, the role of non-invasive imaging in learning about cognitive abilities is discussed in a Perspective article by Feline Lindhout at the Medical Research Council’s Laboratory of Molecular Biology in Cambridge, UK, and her colleagues 1 . In another article, Evelina Fedorenko at the Massachusetts Institute of Technology in Cambridge and her colleagues also draw on this literature to argue that, in humans, language probably serves mainly as a communication tool rather than as a means for thinking or reasoning 2 — and that language is not a prerequisite for complex thought.
One desirable outcome for human neuroscience would be to develop personalized treatments for neurological and psychiatric disorders, because translating the results of studies in animals has not proved successful or sufficient for generating effective therapies at scale. But in grasping these opportunities, researchers must keep in mind that the brain is different from other organs — it’s the seat of people’s memory, experiences and personality. When using the human brain — whether in small cubes removed during neurosurgery, or through 3D organoids made from stem cells and grown in cultures to resemble parts of the developing human brain — for research, scientists must consider the dignity and respect owed to the individuals concerned.
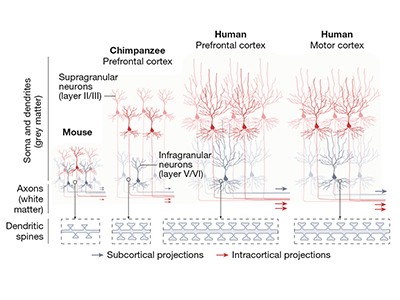
Read the paper: A molecular and cellular perspective on human brain evolution and tempo
The 1964 Declaration of Helsinki is the basis of research ethics for studies involving humans. Participants are asked to complete a consent form before the start of a study. Researchers have to ensure participants are fully informed about the study’s goals and whether and how they will benefit from the research. Sources of funding should also be declared and a participant must be able to withdraw at any time. According to neuroethicist Judy Illes at the University of British Columbia in Vancouver, Canada, ideally, consent should not be something that is done only once. It should be revisited during a study, so that participants can make informed decisions at different stages 3 . This is especially important for studies involving vulnerable people, because their circumstances might change during a study.
In another Perspective article, Tomasz Nowakowski at the University of California, San Francisco, and a team of neurosurgeons, neurologists and neuroscientists 4 call on the neuroscience community to revisit these standards of ethical practice. A key challenge they identify is how to handle the ramifications of advances in machine learning and artificial intelligence (AI).
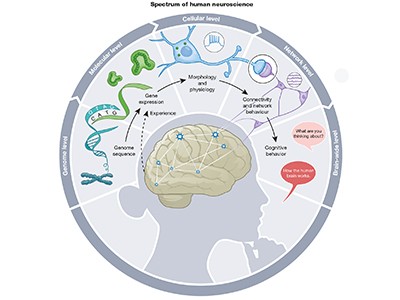
Read the paper: Large-scale neurophysiology and single-cell profiling in human neuroscience
Researchers who use cell atlases, single-cell technologies and spatial-genomic analyses benefit hugely from AI and machine-learning algorithms when analysing large data sets. Yet, AI technologies have the potential to re-identify anonymized information by analysing vast data sets and finding patterns that trace back to individuals. AI models that analyse large data sets can also make predictions related to features of peoples’ behaviour and their cognitive abilities. This has the potential to cause harm, for example, through biased or erroneous profiling of people on the basis of their neurological data, says neuroethicist Karen Rommelfanger, founder of the Institute of Neuroethics, who is based in Atlanta, Georgia.
Nowakowski and his colleagues propose that researchers use controlled archives, access to which requires approval, and that they restrict data use to the conditions specified in consent forms. To implement such changes will require conversations between study participants, academic researchers and the companies that have a considerable role in the current AI advances. Informed-consent information will also need to change, to account for the risks of researchers’ increased reliance on AI tools.
The team is right to stress the need for improved standards in data ethics and sharing that are jointly created by scientists, private partners and the research participants. Without a doubt, human neuroscience is entering a new and important era. However, it can fulfil its goals of improving human experiences only when study participants are involved in discussions about the future of such research.
Nature 630 , 530 (2024)
doi: https://doi.org/10.1038/d41586-024-02022-3
Lindhout, F. W., Krienen, F. M., Pollard, K. S. & Lancaster, M. A. Nature 630 , 596–608 (2024).
Article Google Scholar
Fedorenko, E., Piantadosi, S. T. & Gibson, E. A. F. Nature 630 , 575–586 (2024).
Van der Loos, K. I., Longstaff, H., Virani, A. & Illes, J. J. Law Biosc. 2 , 69–78 (2014).
Lee, A. T., Chang, E. F., Paredes, M. F. & Nowakowski, T. J. Nature 630 , 587–595 (2024).
Download references
Reprints and permissions
Related Articles
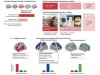
- Medical research
- Neuroscience
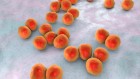
Gut microbiome discovery provides roadmap for life-saving cancer therapies
News 20 JUN 24
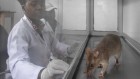
Could rats and dogs detect disease better than the finest lab equipment?
Outlook 19 JUN 24
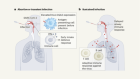
First encounter with SARS-CoV-2: immune portraits of COVID susceptibility
News & Views 19 JUN 24
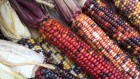
No CRISPR: oddball ‘jumping gene’ enzyme edits genomes without breaking DNA
Technology Feature 27 JUN 24
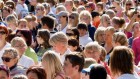
Estonians gave their DNA to science — now they’re learning their genetic secrets
News 26 JUN 24
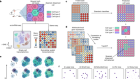
Multiscale topology classifies cells in subcellular spatial transcriptomics
Article 19 JUN 24
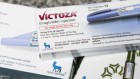
How blockbuster obesity drugs create a full feeling — even before one bite of food
News 27 JUN 24
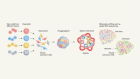
Chimeric brain organoids capture human genetic diversity
News & Views 26 JUN 24
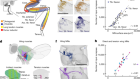
Synaptic architecture of leg and wing premotor control networks in Drosophila
Article 26 JUN 24
Osaka University Immunology Frontier Research Center Postdoctoral Researcher
IFReC, Osaka University in Japan offers Advanced Postdoc Positions for Immunology, Cell Biology, Bioinformatics and Bioimaging.
Suita Campus, Osaka University in Osaka, Japan
Immunology Frontier Research Center, Osaka University
PostDoc Researcher, Magnetic Recording Materials Group, National Institute for Materials Science
Starting date would be after January 2025, but it is negotiable.
Tsukuba, Japan (JP)
National Institute for Materials Science
Tenure-Track/Tenured Faculty Positions
Tenure-Track/Tenured Faculty Positions in the fields of energy and resources.
Suzhou, Jiangsu, China
School of Sustainable Energy and Resources at Nanjing University
Postdoctoral Associate- Statistical Genetics
Houston, Texas (US)
Baylor College of Medicine (BCM)
Senior Research Associate (Single Cell/Transcriptomics Senior Bioinformatician)
Metabolic Research Laboratories at the Clinical School, University of Cambridge are recruiting 3 senior bioinformatician specialists to create a dynam
Cambridge, Cambridgeshire (GB)
University of Cambridge
Sign up for the Nature Briefing newsletter — what matters in science, free to your inbox daily.
Quick links
- Explore articles by subject
- Guide to authors
- Editorial policies
Information
- Author Services
Initiatives
You are accessing a machine-readable page. In order to be human-readable, please install an RSS reader.
All articles published by MDPI are made immediately available worldwide under an open access license. No special permission is required to reuse all or part of the article published by MDPI, including figures and tables. For articles published under an open access Creative Common CC BY license, any part of the article may be reused without permission provided that the original article is clearly cited. For more information, please refer to https://www.mdpi.com/openaccess .
Feature papers represent the most advanced research with significant potential for high impact in the field. A Feature Paper should be a substantial original Article that involves several techniques or approaches, provides an outlook for future research directions and describes possible research applications.
Feature papers are submitted upon individual invitation or recommendation by the scientific editors and must receive positive feedback from the reviewers.
Editor’s Choice articles are based on recommendations by the scientific editors of MDPI journals from around the world. Editors select a small number of articles recently published in the journal that they believe will be particularly interesting to readers, or important in the respective research area. The aim is to provide a snapshot of some of the most exciting work published in the various research areas of the journal.
Original Submission Date Received: .
- Active Journals
- Find a Journal
- Proceedings Series
- For Authors
- For Reviewers
- For Editors
- For Librarians
- For Publishers
- For Societies
- For Conference Organizers
- Open Access Policy
- Institutional Open Access Program
- Special Issues Guidelines
- Editorial Process
- Research and Publication Ethics
- Article Processing Charges
- Testimonials
- Preprints.org
- SciProfiles
- Encyclopedia
Topic Information
Participating journals, topic editors.
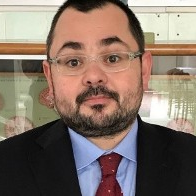
Find support for a specific problem in the support section of our website.
Please let us know what you think of our products and services.
Visit our dedicated information section to learn more about MDPI.
Human Anatomy and Pathophysiology
Dear Colleagues,
Anatomists are scientists that study how the human body is made for correlating structure with function and dysfunction at all levels, from macroscopic to microscopic, from ultrastructural to molecular ones.
Human anatomy provides researchers of many disciplines and areas with all the basic information to effectively plan their experiments and interpret their results.
Hence, this article collection intends to gather review and original papers about human anatomy, histology and embryology, as well as about other closely related medical fields—e.g., physiology, pharmacology, radiology, surgery, clinical medicine, etc.—that have an anatomical focus.
Furthermore, we would like to collect papers about phylogenesis, bioethics, and history of science, especially those that have an anatomical perspective. Sport, physical exercise, nutrition, and active aging can be academic fields of interest for an anatomist as well. Finally, bioengineering and regenerative medicine need knowledge in human anatomy for their advancements.
In conclusion, we welcome submissions from Applied Sciences , Biology, Medicina, Bioengineering and Pathophysiology that cover, but are not limited to, the following topics:
- Advances in human anatomy, histology, and embryology teaching and research.
- Advances in the pathophysiology of human organs or anatomical districts.
- Bioengineering the human body.
- Bioethical aspects in biomedical research about human anatomy and pathobiology.
- Clinical, surgical, and radiological anatomy: new insights.
- From human anatomy to pathophysiology: experimental models.
- Effects of physical exercise on the maintenance and/or improvement of healthy status.
- Extracellular vesicles: looking for new markers of health and disease.
- History of anatomy and medicine: learning from our past.
- How a healthy lifestyle can slow down senescence and contribute to active aging.
- How cell stress can influence cell differentiation, tissue homeostasis, and organ remodeling during the whole lifespan of an individual.
- Human body structures from a phylogenetic point of view.
- Liquid biopsy as a new frontier of medicine, including the personalized one.
- Microbiota/microbiome and its relationship with human body structures.
- Molecular anatomy: the relationship between shape and function at the molecular level.
- Neuroanatomy, neurobiology, neuropathology: new discoveries.
- Nutrition, sport, and health: looking for a virtuous combination.
- Stem cells, 3D cultures, and outgrowth in regenerative medicine.
- Using the corpse: the usefulness of the cadaver in medical training.
Prof. Dr. Francesco Cappello Prof. Dr. Mugurel Constantin Rusu Topic Editors
Journal Name | Impact Factor | CiteScore | Launched Year | First Decision (median) | APC |
---|---|---|---|---|---|
applsci | 2011 | 17.8 Days | CHF 2400 | ||
biology | 2012 | 16.1 Days | CHF 2700 | ||
bioengineering | 2014 | 15.6 Days | CHF 2700 | ||
medicina | 1920 | 17.8 Days | CHF 2200 | ||
pathophysiology | 1994 | 22.8 Days | CHF 1400 |

- Immediately share your ideas ahead of publication and establish your research priority;
- Protect your idea from being stolen with this time-stamped preprint article;
- Enhance the exposure and impact of your research;
- Receive feedback from your peers in advance;
- Have it indexed in Web of Science (Preprint Citation Index), Google Scholar, Crossref, SHARE, PrePubMed, Scilit and Europe PMC.
Published Papers (36 papers)
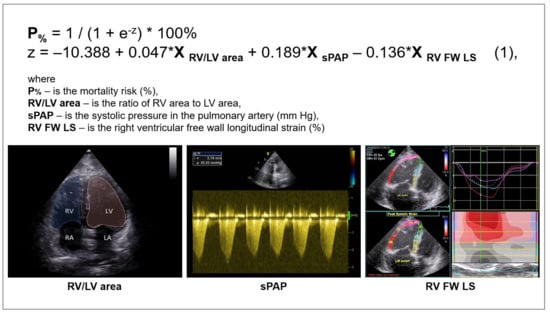
Further Information
Mdpi initiatives, follow mdpi.
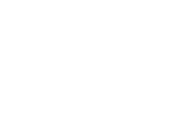
Subscribe to receive issue release notifications and newsletters from MDPI journals
Submit your Manuscript
Submit your abstract.
Biomedical Research with Human Body “Parts”
Cite this chapter.
- Wim J. M. Dekkers 5 &
- Henk A. M. J. ten Have 5
Part of the book series: Philosophy and Medicine ((ESPM,volume 59))
370 Accesses
4 Citations
For several decades, the morality of medical research with human subjects has been one of the main areas of activity and reflection in philosophy of medicine and bioethics. It is argued that precisely the issues and problems of medical experimentation have led to the emergence of modern bioethics [18]. The body of literature that has developed since then focuses exclusively on experimentation with human subjects ‘as a whole’, that is, persons and their (entire) bodies [15,19]. Much less attention has been given to biomedical research (medical experimentation) with human body parts. For example, the Declaration of Helsinki contains no ethical guidelines concerning the collection, storage, and use of human body parts. However, in present-day medicine, human tissues and body fluids are increasingly used for research purposes. The availability of human body parts is of undeniable importance for basic research, for research aimed at improving therapies or developing new treatments. The ethical aspects of procurement, donation, and allocation of human body parts have been discussed in the context of blood transfusion and transplantation medicine, but the scope for using human body parts in the treatment of other patients is rapidly expanding. Human tissues also play an important part in quality control in the health care system Finally, they are sources for the manufacture of diagnostic and therapeutic aids. These various uses of human body parts are connected with various practices of biomedical research. In some cases, body parts are directly obtained for research purposes, but in other cases human tissues, organs, and body fluids are used for purposes other than those for which they were originally obtained. These practices raise questions concerning the ethical and legal issues involved [12], but also lead to an exploration of the nature and value of human bodies and body parts. In this chapter, we shall focus on some of the ethical problems related to biomedical research with human body parts.
This is a preview of subscription content, log in via an institution to check access.
Access this chapter
Subscribe and save.
- Get 10 units per month
- Download Article/Chapter or Ebook
- 1 Unit = 1 Article or 1 Chapter
- Cancel anytime
- Available as PDF
- Read on any device
- Instant download
- Own it forever
- Available as EPUB and PDF
- Compact, lightweight edition
- Dispatched in 3 to 5 business days
- Free shipping worldwide - see info
- Durable hardcover edition
Tax calculation will be finalised at checkout
Purchases are for personal use only
Institutional subscriptions
Unable to display preview. Download preview PDF.
Similar content being viewed by others
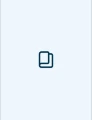
Donation: Tissues and Body Parts
Bibliography
Andrews, L.B.: 1986, ‘My Body, My Property’, Hastings Center Report 16 (5), 28–38.
Article PubMed CAS Google Scholar
Broxmeyer, H.E. et al.: 1992, ‘Human Umbilical Cord Blood as a Source of Transplantable Hematopoietic Stem and Progenitor Cell’, Current Topics in Microbiology and Immunology 177 195–204.
Google Scholar
Campbell, C.S.: 1992, ‘Body, Self, and the Property Paradigm’, Hastings Center Report 22 , 34–42.
PubMed CAS Google Scholar
Caplan, A.L.: 1985, ‘Blood, Sweat, Tears and Profits: The Ethics of the Sale and Use of Patient Derived Materials in Biomedicine’, Clinical Research 33 , 448–451.
Capron, A.M.: 1991, ‘Protection of Research Subjects: Do Special Rules apply in Epidemiology?’, Journal of Clinical Epidemiology 44 , 81S - 89S.
Article PubMed Google Scholar
Corbitt, G., Bailey, A.S., Williams, G.: 1990, ‘HIV Infection in Manchester, 1959’, Lancet 336 , 51.
Dworkin, G. and Kennedy, I.: 1993, ‘Human Tissue: Rights and the Body and its Parts’, Medical Law Review 1 , 291–319.
Gevers, J.K.M.: 1989, ‘Het gebruik van afgenomen lichaamsmateriaal in epidemiologisch onderzoek’, Nederlands Tijdschrift voor Geneeskunde 133 , 173–75.
Gevers, J.K.M.: 1990, Beschikken over cellen en weefsels , Kluwer, Deventer, the Netherlands.
Gezondheidsraad: 1989, Erfelijkheid: maatschappij en wetenschap. Over de mogelijkheden en grenzen van erfelijkheidsdiagnostiek en gentherapie , Staatsdrukkerij en Uitgeverij, Den Haag, the Netherlands.
Harré R.: 1991, Physical Being. A Theory for a Corporeal Psychology . Blackwell, Oxford, UK.
Health Council of the Netherlands, Committee on Human Tissue for Special Purposes: 1994, Proper Use of Human Tissue ,Health Council of the Netherlands (publication no. 1994/01E), The Hague, the Netherlands.
Heubel, F.: 1998, ‘ Defining the Functional Body and its Parts: A review of German law ’, in this volume, pp. 27–37.
James, J.: 1988, ‘ De cel in het organisme; over leven en dood ’, Nederlands Tijdschrift voor Geneeskunde 132 , 2348–2351.
Katz, J.: 1972, Experimentation with Human Beings , Russell Sage Foundation, NY.
Munzer, S.R.: 1994, ‘An Uneasy Case against Property Rights in Body Parts’, Social Philosophy Policy 11 (2), 259–286.
Protection of Human Subjects: March 8, 1983, 45 Code of Federal Regulations , 46, Office of Protection from Research Risks Reports.
Rothman, D.J.: 1991, Strangers at the Bedside . A History of How Law and Bioethics Transformed Medical Decision Making, Basic Books, NY.
Spicker, S.F. et al.: 1988, The Use of Human Beings in Research . With special reference to clinical trials, Kluwer Academic Publishers, Dordrecht, the Netherlands.
Gewijzigd voorstel van Wet inzake medisch wetenschappelijk onderzoek met mensen. Tweede Kamer der Staten-Generaal, vergaderjaar: 1995–1996.
Download references
Author information
Authors and affiliations.
Department of Ethics, Philosophy and History of Medicine, and Center for Ethics, Catholic University of Nijmegen, Nijmegen, The Netherlands
Wim J. M. Dekkers & Henk A. M. J. ten Have
You can also search for this author in PubMed Google Scholar
Editor information
Editors and affiliations.
Center for Ethics and Department of Ethics, Philosophy and History of Medicine, Catholic University of Nijmegen, Nijmegen, The Netherlands
Henk A. M. J. Ten Have
Center for Health Policy and Ethics, Creighton University, Omaha, Nebraska, USA
Jos V. M. Welie
Rights and permissions
Reprints and permissions
Copyright information
© 1998 Springer Science+Business Media Dordrecht
About this chapter
Dekkers, W.J.M., ten Have, H.A.M.J. (1998). Biomedical Research with Human Body “Parts”. In: Ten Have, H.A.M.J., Welie, J.V.M. (eds) Ownership of the Human Body. Philosophy and Medicine, vol 59. Springer, Dordrecht. https://doi.org/10.1007/978-94-015-9129-4_5
Download citation
DOI : https://doi.org/10.1007/978-94-015-9129-4_5
Publisher Name : Springer, Dordrecht
Print ISBN : 978-90-481-5059-5
Online ISBN : 978-94-015-9129-4
eBook Packages : Springer Book Archive
Share this chapter
Anyone you share the following link with will be able to read this content:
Sorry, a shareable link is not currently available for this article.
Provided by the Springer Nature SharedIt content-sharing initiative
- Publish with us
Policies and ethics
- Find a journal
- Track your research
Academia.edu no longer supports Internet Explorer.
To browse Academia.edu and the wider internet faster and more securely, please take a few seconds to upgrade your browser .
- We're Hiring!
- Help Center
- Most Cited Papers
- Most Downloaded Papers
- Newest Papers
- Last »
- Radio Frequency Follow Following
- Pressure Sensor Follow Following
- Embalming Follow Following
- Skeleton Follow Following
- Nanoparticle Follow Following
- Polymer Technology Follow Following
- Max/MSP/Jitter Follow Following
- Technique Follow Following
- RF MEMS Follow Following
- Composite resins Follow Following
Enter the email address you signed up with and we'll email you a reset link.
- Academia.edu Publishing
- We're Hiring!
- Help Center
- Find new research papers in:
- Health Sciences
- Earth Sciences
- Cognitive Science
- Mathematics
- Computer Science
- Academia ©2024
Loading metrics
Open Access
Peer-reviewed
Research Article
Structure, function, and control of the human musculoskeletal network
Roles Data curation, Formal analysis, Methodology, Validation, Writing – original draft, Writing – review & editing
Affiliations Department of Bioengineering, University of Pennsylvania, Philadelphia, Pennsylvania, United States of America, Perelman School of Medicine, University of Pennsylvania, Philadelphia, Pennsylvania, United States of America
Roles Methodology, Visualization, Writing – original draft
Affiliations Department of Bioengineering, University of Pennsylvania, Philadelphia, Pennsylvania, United States of America, Department of Mathematics, University of Buffalo, Buffalo, New York, United States of America
Roles Data curation, Methodology
Affiliations Department of Bioengineering, University of Pennsylvania, Philadelphia, Pennsylvania, United States of America, Department of Electrical and Systems Engineering, University of Pennsylvania, Philadelphia, Pennsylvania, United States of America
Roles Methodology, Validation
Affiliation Haverford College, Haverford, Pennsylvania, United States of America
Roles Visualization
Affiliations Haverford College, Haverford, Pennsylvania, United States of America, Philadelphia Academy of Fine Arts, Philadelphia, Pennsylvania, United States of America
Roles Investigation, Methodology, Resources, Software
Affiliations Department of Bioengineering, University of Pennsylvania, Philadelphia, Pennsylvania, United States of America, Applied Mathematical and Computational Science Graduate Group, University of Pennsylvania, Philadelphia, Pennsylvania, United States of America
Roles Conceptualization, Funding acquisition, Investigation, Project administration, Resources, Software, Supervision, Writing – original draft, Writing – review & editing
* E-mail: [email protected]
Affiliations Department of Bioengineering, University of Pennsylvania, Philadelphia, Pennsylvania, United States of America, Department of Electrical and Systems Engineering, University of Pennsylvania, Philadelphia, Pennsylvania, United States of America, Department of Neurology, University of Pennsylvania, Philadelphia, Pennsylvania, United States of America

- Andrew C. Murphy,
- Sarah F. Muldoon,
- David Baker,
- Adam Lastowka,
- Brittany Bennett,
- Muzhi Yang,
- Danielle S. Bassett
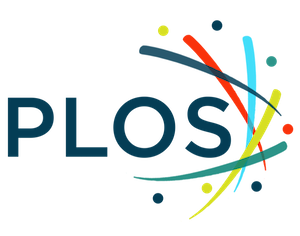
- Published: January 18, 2018
- https://doi.org/10.1371/journal.pbio.2002811
- Reader Comments
The human body is a complex organism, the gross mechanical properties of which are enabled by an interconnected musculoskeletal network controlled by the nervous system. The nature of musculoskeletal interconnection facilitates stability, voluntary movement, and robustness to injury. However, a fundamental understanding of this network and its control by neural systems has remained elusive. Here we address this gap in knowledge by utilizing medical databases and mathematical modeling to reveal the organizational structure, predicted function, and neural control of the musculoskeletal system. We constructed a highly simplified whole-body musculoskeletal network in which single muscles connect to multiple bones via both origin and insertion points. We demonstrated that, using this simplified model, a muscle’s role in this network could offer a theoretical prediction of the susceptibility of surrounding components to secondary injury. Finally, we illustrated that sets of muscles cluster into network communities that mimic the organization of control modules in primary motor cortex. This novel formalism for describing interactions between the muscular and skeletal systems serves as a foundation to develop and test therapeutic responses to injury, inspiring future advances in clinical treatments.
Author summary
While network science is frequently used to characterize networks from genomics, proteomics, and connectomics, its utility in understanding biomechanics, orthopedics, and physical therapy has remained largely unexplored. Indeed, current clinical practice and knowledge regarding the musculoskeletal system largely focuses on single areas of the body, single muscles, or single injuries and therefore remains agnostic to mesoscale or global features of the body’s architecture that may have critical implications for injury and recovery. We addressed this gap by representing the musculoskeletal system as a graph or network, in which we considered bones and the muscular connections between them. By modeling muscles as springs and bones as point masses, we developed a perturbative approach to interrogate the function of this network. Employing this model, we calculated the network level effects of perturbing individual muscles. Using this formalism, we are able to draw new parallels between this system and the primary motor cortex that controls it, and illustrate clinical connections between network structure and muscular injury.
Citation: Murphy AC, Muldoon SF, Baker D, Lastowka A, Bennett B, Yang M, et al. (2018) Structure, function, and control of the human musculoskeletal network. PLoS Biol 16(1): e2002811. https://doi.org/10.1371/journal.pbio.2002811
Academic Editor: Graham Taylor, University of Oxford, United Kingdom of Great Britain and Northern Ireland
Received: April 21, 2017; Accepted: December 15, 2017; Published: January 18, 2018
Copyright: © 2018 Murphy et al. This is an open access article distributed under the terms of the Creative Commons Attribution License , which permits unrestricted use, distribution, and reproduction in any medium, provided the original author and source are credited.
Data Availability: All relevant data are within the paper and its Supporting Information files. The two musculoskeletal graphs used, as well as muscle community assignments, and data used to generate all figures can be found at DOI: 10.5281/zenodo.1069104 .
Funding: National Science Foundation (grant number PHY-1554488). The funder had no role in study design, data collection and analysis, decision to publish, or preparation of the manuscript.
Competing interests: The authors have declared that no competing interests exist.
Introduction
The interconnected nature of the human body has long been the subject of both scientific inquiry and superstitious beliefs. From the ancient humors linking heart, liver, spleen, and brain with courage, calm, and hope [ 1 ] to the modern appreciation of the gut–brain connection [ 2 ], humans tend to search for interconnections between disparate parts of the body to explain complex phenomena. Yet, a tension remains between this basic conceptualization of the human body and the reductionism implicit in modern science [ 3 ]. An understanding of the entire system is often relegated to a futuristic world, while individual experiments fine-tune our understanding of minute component parts.
The human musculoskeletal system is no exception to this dichotomy. While medical practice focuses in hand, foot, or ankle, clinicians know that injuries to a single part of the musculoskeletal system necessarily impinge on the workings of other (even remotely distant) parts [ 4 ]. An injury to an ankle can alter gait patterns, leading to chronic back pain; an injury to a shoulder can alter posture, causing radiating neck discomfort. Understanding the fundamental relationships between focal structure and potential distant interactions requires a holistic approach.
Here, we detail such an approach. Our conceptual framework is motivated by recent theoretical advances in network science [ 5 ], which is an emerging discipline built from an ordered amalgamation of mathematics (specifically, graph theory [ 6 ]) and physics (specifically, statistical mechanics [ 7 ]), computer science, statistics [ 8 ], and systems engineering. The approach simplifies complex systems by delineating their components and mapping the pattern of interactions between those components [ 9 ]. This representation appears particularly appropriate for the study of the human musculoskeletal system, which is composed of bones and the muscles that link them. In this study, we used this approach to assess the structure, function, and control of the musculoskeletal system.
The use of network science to understand the musculoskeletal system has increased in recent years [ 10 ]. However, the framework has largely been employed to investigate the properties of local muscle or bone networks. For example, the local structure of the skull has been examined to investigate how bones can be categorized [ 11 ]. Additionally, studies of the topology of the musculoskeletal spine network have been conducted to evaluate stresses and strains across bones [ 12 ]. A few studies do exist that address the entire musculoskeletal system, although they do not use the mathematical tools that we employed here [ 13 , 14 ]. The current study differs from previous work in its assessment of the entire musculoskeletal system combined with the mathematical tools of network science.
Within this broader context, we focused on the challenge of rehabilitation following injury to either skeletal muscle or cerebral cortex. Direct injury to a muscle or associated tendon or ligament affects other muscles via compensatory mechanisms of the body [ 15 ]. Similarly, loss of use of a particular muscle or muscle group from direct cortical insult can result in compensatory use of alternate muscles [ 16 , 17 ]. How the interconnections of the musculoskeletal system are structured and how they function directly constrains how injury to a certain muscle will affect the musculoskeletal system as a whole. Understanding these interconnections could provide much needed insight into which muscles are most at risk for secondary injury due to compensatory changes resulting from focal injury, thereby informing more comprehensive approaches to rehabilitation. Additionally, an understanding of how the cortex maps onto not only single muscles but also groups of topologically close muscles could inform future empirical studies of the relationships between focal injuries (including stroke) to motor cortex and risk for secondary injury.
Materials and methods
Network construction.
Using the Hosford Muscle tables [ 18 ], we constructed a musculoskeletal hypergraph by representing 173 bones (several of these are actually ligaments and tendons) as nodes and 270 muscles as hyperedges linking those nodes (muscle origin and insertion points are listed in S9 Table ). This hypergraph can also be interpreted as a bipartite network, with muscles as one group and bones as the second group ( Fig 1a ). The 173 × 270 incidence matrix C of the musculoskeletal network is thus defined as C ij = 1 if v i ∈ e j and 0 otherwise, where V = {v 1 , · · ·, v 173 } is the set of nodes (bones) and E = {e 1 , · · ·, e 270 } is the set of hyperedges (muscles). This hypergraph representation of the body eliminates much of the complexity from the musculoskeletal system, encoding only which muscles attach to which bones. All analysis was applied to only one half (left or right) of the body, because each cerebral hemisphere controls only the contralateral side of the body. Therefore, we further simplified our model by assuming left–right symmetry; in any figures in which both halves of the body are shown, the second half is present purely for visual intuition.
- PPT PowerPoint slide
- PNG larger image
- TIFF original image
(a) The musculoskeletal network was first converted to a bipartite matrix, where 1/0 indicates a present/absent muscle±bone connection. (b) Communities of topologically related muscles are identified by (1) transforming the hypergraph to a muscle±muscle graph, in which each entry encodes the number of common bones of each muscle pair, and (2) subsequently, muscles were broken into communities, in which constituent members connected more densely to other members within their community than to members in other communities. (c) To facilitate perturbations, the musculoskeletal network was physically embedded, such that bones (nodes) are initially placed at their correct anatomical positions. (d) To understand the impact of single muscles on the interconnected system, all nodes linked by a selected hyperedge were perturbed in a fourth spatial dimension.
https://doi.org/10.1371/journal.pbio.2002811.g001
The bone-centric graph A and muscle-centric graph B ( Fig 1b ) are simply the one-mode projections of C. The projection onto bones is A = C T C, and the projection onto muscles is B = CC T . Then, the diagonal elements were set equal to zero, leaving us with a weighted adjacency matrix [ 5 ]. We obtained estimated anatomical locations for the center of mass of each muscle (and bone) by examining anatomy texts [ 19 ] and estimating x-, y-, and z-coordinates for mapping to a graphical representation of a human body ( Fig 1c ).
Calculation of impact score
To measure the potential functional role of each muscle in the network, we stretched a muscle hyperedge and measured the impact of the perturbation on the rest of the network. Rather than perturbing the network in some arbitrary three-dimensional direction, we extended the scope of our simulation into a fourth dimension. When perturbing a muscle, we displaced all of the nodes (bones) contained in that muscle hyperedge by a constant vector in the fourth dimension and held them with this displacement ( Fig 1d ). The perturbation then rippled through the network of springs in response. We sequentially stretched each muscle hyperedge and defined the impact score of this perturbation to be the total distance moved by all nodes in the musculoskeletal network from their original positions. The displacement value is the summed displacement over all time points, from perturbation onset to an appropriate cutoff for equilibration time. Here, we solved for the equilibrium of the system by allowing dynamics to equalize over a sufficient period of time. Note that the equilibrium can also be solved for using a steady-state, nondynamic approach; we chose to use dynamics in this instance to more broadly support future applications.
Impact score deviation
For each muscle, we calculated an index that quantifies how much the impact score of that muscle deviates from expected, given its hyperedge degree; we call this index “impact deviation”. We begin by constructing a null model that dictates the expected impact under a set of statistical assumptions. In the current study, we used several different null models with differing sets of assumptions, which we detail in later sections. Impact deviation was computed as follows: we calculated the mean, standard deviation, and 95% confidence intervals (CIs) for each of the null hypergraph degree categories from an ensemble of 100 null hypergraphs. The distance from a given muscle to the mean ± 95% CI (whichever is closest) was calculated and divided by the standard deviation of that null hypergraph degree distribution. In this way, we calculated deviation from the expected value, in standard deviations (similar to a z-score). Table 1 contains the muscles that lie outside the 95% CI of deviation ratios, relative to their hyperedge degree. Muscles can be naturally grouped according to the homunculus, a coarse one-dimensional representation of how the control areas of muscles group onto the motor cortex. For a given homunculus group, we calculated the deviation ratio as the number of muscles with positive deviation divided by the total number of muscles in the group ( Table 2 ).
The muscles on the left side have less impact than expected, given their hyperedge degree: their impacts are more than 1.96 standard deviations below the mean, indicating that they lie outside the 95% confidence interval of the distribution. The muscles on the right side have more impact than expected given their hyperedge degree: their impacts are more than 1.96 standard deviations above the mean, ordered from most to least extreme. This table shows the muscles that had the greatest positive and greatest negative difference in impact, relative to degree-matched controls.
https://doi.org/10.1371/journal.pbio.2002811.t001
Categories on the left are composed entirely of muscles with less impact than expected, compared to degree-matched controls. Categories on the right are composed entirely of muscles with more impact than expected, compared to degree-matched controls.
https://doi.org/10.1371/journal.pbio.2002811.t002
Community detection
The above method of community detection is nondeterministic [ 23 ]. That is, the same solution will not be reached on each individual run of the algorithm. Therefore, one must ensure that the community assignments used are a good representation of the network and not just a local maximum of the landscape. We therefore maximized the modularity quality function 100 times, obtaining 100 different community assignments. From this set of solutions, we identified a robust representative consensus community structure [ 24 ]. S1 Fig illustrates how the detected communities change as a function of the resolution parameter for the muscle-centric network.
Network null models
We use rewired graphs as a null model against which to compare the empirical data. Specifically, we constructed a null hypergraph by rewiring muscles that are assigned the same category ( Table 3 , defined below) uniformly at random. In this way, muscles of the little finger will only be rewired within the little finger, and similarly for muscles in other categories. Importantly, this method also preserves the degree of each muscle as well as the degree distribution of the entire hypergraph.
https://doi.org/10.1371/journal.pbio.2002811.t003
Categories were assigned to muscles such that the overall topology of the musculoskeletal system was grossly preserved, and changes were spatially localized. Specifically, we partitioned the muscles into communities of roughly size 3, such that each muscle was grouped with the two muscles that are most topologically related. We then permuted only within these small groups. This is a data-driven way of altering connections only within very small groups of related muscles.
Multidimensional scaling
To conduct multidimensional scaling (MDS) on the muscle-centric network, the weighted muscle-centric adjacency matrix was simplified to a binary matrix (all nonzero elements set equal to 1). From this data, a distance matrix D was constructed, the elements D ij of which are equal to the length of the shortest path between muscles i and j, or are equal to 0 if no path exists. MDS is then applied to this distance matrix to yield its first principal component using the MATLAB function, cmdscale.m. To construct the binary matrix, a threshold of 0 was set, and all values above that threshold were converted to 1. However, to make analysis robust to this choice, we explored a range of threshold values to verify that results are invariant with respect to threshold. The upper bound of the threshold range was established by determining the maximal value that would maintain a fully connected matrix; otherwise, the distance matrix D would have entries of infinite weight. In our case, this value was 0.0556 × max(B′). Within this range of thresholds (i.e., for all thresholds resulting in fully connected matrices), results were qualitatively consistent. As a supplementary analysis, we also employed a method of constructing a distance matrix from a weighted adjacency matrix in order to preclude thresholding ( S5 Fig ), and we again observed qualitatively consistent results.
Muscle injury data
We calculated the correlation between impact score and muscle injury recovery times. Injury recovery times were collected from the sports medicine literature and included injury to the triceps brachii and shoulder muscles [ 26 ]; thumb muscles [ 27 ]; latissimus dorsi and teres major [ 28 ]; biceps brachii [ 29 ]; ankle muscles [ 30 ]; neck muscles [ 31 ]; jaw muscles [ 32 ]; hip muscles [ 33 ]; eye/eyelid muscles [ 34 ]; and muscles of the knee [ 35 ], elbow [ 36 ], and wrist/hand [ 37 ]. The recovery times and associated citations, listed in Table 4 , are average recovery times gathered from population studies. If the literature reported a range of different severity levels and associated recovery times for a particular injury, the least severe level was selected. If the injury was reported for a group of muscles rather than a single muscle, the impact score deviation for that group was averaged together. Data points for muscle groups were weighted according to the number of muscles in that group for the purpose of the linear fit. The fit was produced using the MATLAB function, fitlm.m, with option “Robust” set to “on.” Robust regression is a method of regression designed to be less sensitive to outliers within the data, in which outliers are down-weighted in the regression model.
https://doi.org/10.1371/journal.pbio.2002811.t004
Somatotopic representation area data
We calculated the correlation between impact score deviation and the area of somatotopic representation devoted to a particular muscle group. The areas of representation were collected from two separate sources [ 38 , 39 ]. The volumes and associated citations are listed in Table 5 . In both studies, subjects were asked to articulate a joint repetitively, and the volumes of the areas of primary motor cortex that underwent the greatest change in BOLD signal were recorded. We then calculated the correlation coefficient between cortical volumes and the mean impact of all muscles associated with that joint, as determined by the Hosford Muscle tables. We found a significant linear correlation between the two measures by using the MATLAB function, fitlm.m, with option “Robust” set to “on.”
https://doi.org/10.1371/journal.pbio.2002811.t005
Structure of the human musculoskeletal network
To examine the structural interconnections of the human musculoskeletal system, we used a hypergraph approach. Drawing from recent advances in network science [ 5 ], we examined the musculoskeletal system as a network in which bones (network nodes) are connected to one another by muscles (network hyperedges). A hyperedge is an object that connects multiple nodes; muscles link multiple bones via origin and insertion points. The degree, k, of a hyperedge is equal to the number of nodes it connects; thus, the degree of a muscle is the number of bones it contacts. For instance, the trapezius is a high-degree hyperedge that links 25 bones throughout the shoulder blade and spine; conversely, the adductor pollicis is a low-degree hyperedge that links 7 bones in the hand ( Fig 2a and 2b ). A collection of hyperedges (muscles) that share nodes (bones) is referred to as a hypergraph: a graph H = (V, E) with N nodes and M hyperedges, where V = {v 1 ,···, v N } is the set of nodes and E = {e 1 ,···, e M } is the set of hyperedges.
(a) Left: Anatomical drawing highlighting the trapezius. Right: Transformation of the trapezius into a hyperedge (red; degree k = 25), linking 25 nodes (bones) across the head, shoulder, and spine. (b) Adductor pollicis muscle linking 7 bones in the hand. (c) Spatial projection of the hyperedge degree distribution onto the human body. High-degree hyperedges are most heavily concentrated at the core. (d) The musculoskeletal network displayed as a bipartite matrix (1 = connected, 0 otherwise). (e) The hyperedge degree distribution for the musculoskeletal hypergraph, which is significantly different than that expected in a random hypergraph. Data available for (e) at DOI : 10.5281/zenodo.1069104 .
https://doi.org/10.1371/journal.pbio.2002811.g002
The representation of the human musculoskeletal system as a hypergraph facilitates a quantitative assessment of its structure ( Fig 2c ). We observed that the distribution of hyperedge degree is heavy-tailed: most muscles link 2 bones, and a few muscles link many bones ( Fig 2d and 2e ). The skew of the degree distribution differs significantly from that of random networks (two-sample Kolmogorov-Smirnov test, KS = 0.37, p < 0.0001, see Materials and methods ) [ 5 ], indicating the presence of muscles of unexpectedly low and high degree ( Fig 2e ).
Function of the human musculoskeletal network
To probe the functional role of muscles within the musculoskeletal network, we employed a simplified model of the musculoskeletal system and probed whether the model could generate useful clinical correlates. We implemented a physical model in which bones form the core scaffolding of the body, while muscles fasten this structure together. Each node (bone) is represented as a mass, whose spatial location and movement are physically constrained by the hyperedges (muscles) to which it is connected. Specifically, bones are points located at their center of mass, derived from anatomy texts [ 19 ], and muscles are springs (damped harmonic oscillators) connecting these points [ 40 , 41 ]; for a hyperedge of degree k, we created k(k − 1)/2 springs linking the k nodes. That is, for a muscle connecting k bones, we placed springs such that each of the k muscles had a direct spring connection to each of the other k − 1 bones.
Next, we perturbed each of 270 muscles in the body and calculated their impact score on the network (see Materials and methods and Fig 1c and 1d ). As a muscle is physically displaced, it causes a rippling displacement of other muscles throughout the network. The impact score of a muscle is the mean displacement of all bones (and indirectly, muscles) resulting from its initial displacement. We observed a significant positive correlation between muscle degree and impact score (F(1,268) = 23.3, R 2 = 0.45, p < 0.00001; Fig 3a ), suggesting that hyperedge structure dictates the functional role of muscles in the musculoskeletal network. Muscles with a larger number of insertion and origin points have a greater impact on the musculoskeletal system when perturbed than muscles with few insertion and origin points [ 42 ]. We can gain further insights into the results of these analyses by explicitly studying the relation between impact score and statistical measures of the network’s topology. In S11 Fig , we show that the network function as measured by the impact score was significantly correlated with the average shortest path length. While the network statistics are static in nature, their functional interpretation is provided by the perturbative simulations of system dynamics.
(a) The impact score plotted as a function of the hyperedge degree for a null hypergraph model and the observed musculoskeletal hypergraph. (b) Impact score deviation correlates with muscle recovery time following injury to muscles or muscle groups (F(1,12) = 37.3, R 2 = 0.757, p < 0.0001). Shaded areas indicate 95% confidence intervals, and data points are scaled according to the number of muscles included. The plot is numbered as follows, corresponding to Table 4 : triceps (1), thumb (2), latissimus dorsi (3), biceps brachii (4), ankle (5), neck (6), jaw (7), shoulder (8), teres major (9), hip (10), eye muscles (11), knee (12), elbow (13), wrist/hand (14). Data available at DOI : 10.5281/zenodo.1069104 .
https://doi.org/10.1371/journal.pbio.2002811.g003
To guide interpretation, it is critical to note that the impact score, while significantly correlated with muscle degree, is not perfectly predicted by it ( Fig 3a ). Instead, the local network structure surrounding a muscle also plays an important role in its functional impact and ability to recover. To better quantify the effect of this local network structure, we asked whether muscles existed that had significantly higher or significantly lower impact scores than expected in a null network. We defined a positive (negative) impact score deviation that measures the degree to which muscles are more (less) impactful than expected in a network null model (see Materials and methods ). This calculation resulted in a metric that expresses the impact of a particular muscle, relative to muscles of identical hyperedge degree in the null model. In other words, this metric accounts for the complexity of a particular muscle ( Table 1 ).
Is this mathematical model clinically relevant? Does the body respond differently to injuries to muscles with higher impact score than to muscles with lower impact score? To answer this question, we assessed the potential relationship between muscle impact and recovery time following injury. Specifically, we gathered data on athletic sports injuries and the time between the initial injury and return to sport. Critically, we observed that recovery times were strongly correlated with impact score deviations of the individual muscle or muscle group injured (F(1,12) = 37.3, R 2 = 0.757, p < 0.0001; Fig 3b ), suggesting that our mathematical model offers a useful clinical biomarker for the network’s response to damage. We note that it is important to consider the fact that recovery might be slower in a person who is requiring maximal effort in a performance sport, compared to an individual who is seeking only to function in day-to-day life. In order to generalize our findings to the entire population, we therefore also examined recovery time data collected from nonathletes, and we present these complementary results in the Supporting information ( S6 Text ).
Finally, to provide intuition regarding how focal injury can produce distant effects potentially slowing recovery, we calculated the impact of the ankle muscles and determined which other muscles were most impacted. That is, for each individual ankle muscle, we calculated the impact on each of the remaining 264 non-ankle muscles and then averaged this over all ankle muscles. Out of the 264 non-ankle muscles, the single muscle that is most impacted by the perturbation of ankle muscles is the biceps femoris of the hip, and the second most impacted is the vastus lateralis of the knee. Additionally, the muscle most impacted by perturbation to hip muscles is the soleus.
Control of the human musculoskeletal network
What is the relationship between the functional impact of a muscle on the body and the neural architecture that affects control? Here, we interrogate the relationship between the musculoskeletal system and the primary motor cortex. We examined the cerebral cortical representation map area devoted to muscles with low versus high impact by drawing on the anatomy of the motor strip represented in the motor homunculus [ 43 ] ( Fig 4a ), a coarse one-dimensional representation of the body in the brain [ 44 ]. We observed that homunculus areas differentially control muscles with positive versus negative impact deviation scores ( Table 2 ). Moreover, we found that homunculus areas controlling only positively (negatively) deviating muscles tend to be located medially (laterally) on the motor strip, suggesting the presence of a topological organization of a muscle’s expected impact in neural tissue. To probe this pattern more deeply, for each homunculus area, we calculated a deviation ratio as the percent of muscles that positively deviated from the expected impact score (i.e., a value of 1 for brow, eye, face and a value of 0 for knee, hip, shoulder; see Table 2 ). We found that the deviation ratio was significantly correlated with the topological location on the motor strip (F(1,19) = 21.3, R 2 = 0.52, p < 0.001; Fig 4b ).
(a) The primary motor cortex homunculus as constructed by Penfield. (b) Deviation ratio correlates significantly with homuncular topology (F(1,19) = 21.3, R 2 = 0.52, p < 0.001), decreasing from medial (area 0) to lateral (area 22). (c) Impact score deviation significantly correlates with motor strip activation volume (F(1,5) = 14.4, R 2 = 0.743, p = 0.012). Data points are sized according to the number of muscles required for the particular movement. The plot is numbered as follows, corresponding to Table 5 : thumb (1), index finger (2), middle finger (3), hand (4), all fingers (5), wrist (6), elbow (7). (d) Correlation between the spatial ordering of Penfield’s homunculus categories and the linear muscle coordinate from a multidimensional scaling analysis (F(1,268) = 316, R 2 = 0.54, p < 0.0001). Data available at DOI : 10.5281/zenodo.1069104 .
https://doi.org/10.1371/journal.pbio.2002811.g004
As a stricter test of this relationship between a muscle’s impact on the network and neural architecture, we collated data for the physical volumes of functional MRI-based activation on the motor strip that are devoted to individual movements (e.g., finger flexion or eye blinks). Activation volumes are defined as voxels that become activated (defined by blood-oxygen-level-dependent signal) during movement [ 38 , 39 ]. Critically, we found that the functional activation volume independently predicts the impact score deviation of muscles ( Fig 4c , F(1,5) = 14.4, p = 0.012, R 2 = 0.743), consistent with the intuition that the brain would devote more real estate in gray matter to the control of muscles that are more impactful than expected in a null model. Again, impact deviation is a metric that accounts for the hyperedge degree of a particular muscle and is relative to the impact of muscles with identical hyperedge degree in the null model. Thus, the impact deviation measures the local network topology beyond simply the immediate connections of the muscle in question.
As a final test of this relationship, we asked whether the neural control strategy embodied by the motor strip is optimally mapped to muscle groups. We constructed a muscle-centric graph by connecting two muscles if they touch on the same bone ( Fig 1c , left). We observed the presence of groups of muscles that were densely interconnected with one another, sharing common bones. We extracted these groups using a clustering technique designed for networks [ 45 , 46 ], which provides a data-driven partition of muscles into communities ( Fig 1b , right). To compare the community structure present in the muscle network to the architecture of the neural control system, we considered each of the 22 categories in the motor homunculus [ 18 ] as a distinct neural community and compared these brain-based community assignments with the community assignments obtained from a data-driven partition of the muscle network. Using the Rand coefficient [ 47 ], we found that the community assignments from both homunculus and muscle network were statistically similar (z Rand > 10), indicating a correspondence between the modular organization of the musculoskeletal system and the structure of the homunculus. For example, the triceps brachii and the biceps brachii belong to the same homuncular category, and we found that they also belong to the same topological muscle network community.
Next, because the homunculus has a linear topological organization, we asked whether the order of communities within the homunculus ( Table 3 ) was similar to a data-driven ordering of the muscle groups in the body, as determined by MDS [ 48 ]. From the muscle-centric network ( Fig 1b ), we derived a distance matrix that encodes the smallest number of bones that must be traversed to travel from one muscle to another. An MDS of this distance matrix revealed a one-dimensional linear coordinate for each muscle, such that topologically close muscles were close together and topologically distant muscles were far apart. We observed that each muscle’s linear coordinate is significantly correlated with its homunculus category ( Fig 4d , F(1,268) = 316, p < 0.0001, R 2 = 0.54), indicating an efficient mapping between the neural representation of the muscle system and the network topology of the muscle system in the body.
Our results from Fig 4d demonstrate a correspondence between the topology of the homunculus and a data-driven ordering of muscles obtained by considering the topological distances between them. This result could be interpreted in one of two ways: one reasonable hypothesis is that because most connections in the musculoskeletal network are short range, the finding is primarily driven by short-range connections. A second reasonable hypothesis is that while short-range connections are the most prevalent, long-range connections form important intramodular links that help determine the organization of the network. To arbitrate between these two hypotheses, we considered two variations of our MDS experiment: one including only connections shorter than the mean connection length and the other including only connections longer than the mean connection length. We found that the data-driven ordering derived from only short and only long connections both led to significant correlations with the homuncular topology (F(1,268) = 24.9, R 2 = 0.085, p < 0.0001 and F(1,268) = 5, R 2 = 0.018, p = 0.026, respectively). Notably, including both long and short connections leads to a stronger correlation with homuncular topology than considering either independently, suggesting a dependence on connections of all lengths. It would be interesting in the future to test the degree to which this network-to-network map is altered in individuals with motor deficits or changes following stroke.
By representing the complex interconnectivity of the musculoskeletal system as a network of bones (represented by nodes) and muscles (represented by hyperedges), we gained valuable insight into the organization of the human body. The study of anatomical networks using similar methods is becoming more common in the fields of evolutionary and developmental biology [ 10 ]. However, the approach has generally been applied only to individual parts of the body—including the arm [ 49 ], the head [ 11 ], and the spine [ 12 ]—thereby offering insights into how that part of the organism evolved [ 50 , 51 ]. Moreover, even when full body musculature [ 13 ] and the neuromusculoskeletal [ 14 ] system more generally have been modeled, some quantitative claims can remain elusive, in large part due to the lack of a mathematical language in which to discuss the complexity of the interconnection patterns. In this study, we offer an explicit and parsimonious representation of the complete musculoskeletal system as a graph of nodes and edges, and this representation allowed us to precisely characterize the network in its entirety.
When modeling a system as a network, it is important to begin the ensuing investigation by characterizing a few key architectural properties. One particularly fundamental measure of a network’s structure is its degree distribution [ 52 ], which describes the heterogeneity of a node’s connectivity to its neighbors in a manner that can provide insight into how the system formed [ 7 ]. We observed that the degree distribution of the musculoskeletal system is significantly different from that expected in a null graph ( Fig 2e ), displaying fewer high-degree nodes and an overabundance of low-degree nodes. The discrepancy between real and null model graphs is consistent with the fact that the human musculoskeletal system develops in the context of physical and functional constraints that together drive its decidedly nonrandom architecture [ 53 ]. The degree distribution of this network displays a peak at approximately degree two, that is then followed by a relatively heavy tail of high-degree nodes. The latter feature is commonly observed in many types of real-world networks [ 54 ], whose hubs may be costly to develop, maintain, and use [ 55 , 56 ] but play critical roles in system robustness, enabling swift responses [ 55 ], buffering environmental variation [ 57 ], and facilitating survival and reproduction [ 58 ]. The former feature—the distribution’s peak—is consistent with the intuition that most muscles within the musculoskeletal system connect with only two bones, primarily for the function of simple flexion or extension at a joint. By contrast, there are only a few muscles that require a high degree to support highly complex movements, such as maintaining the alignment and angle of the spinal column by managing the movement of many bones simultaneously. These expected findings provide important validation of the model as well as offer a useful visualization of the musculoskeletal system.
The musculoskeletal network is characterized by a particularly interesting property that distinguishes it from several other real-world networks: the fact that it is embedded into three-dimensional space [ 59 ]. This property is not observed in semantic networks [ 60 ] or the World Wide Web [ 61 ], which encode relationships between words, concepts, or documents in some abstract (and very likely non-euclidean) geometry. In contrast, the musculoskeletal system composes a volume, with nodes having specific coordinates and edges representing physically extended tissues. To better understand the physical nature of the musculoskeletal network, we examined the anatomical locations of muscles with varying degrees ( Fig 2c ). We observed that muscle hubs occur predominantly in the torso, providing dense structural interconnectivity that can stabilize the body’s core and prevent injury [ 62 ]. Specifically, high-degree muscles cluster about the body’s midline, close to the spine, and around the pelvic and shoulder girdle, consistent with the notion that both agility and stability of these areas requires an ensemble of muscles with differing geometries and tissue properties [ 63 ]. Indeed, muscles at these locations must support not only flexion and extension but also abduction, adduction, and both internal and external rotation.
It is important to note that significant variation exists within the musculoskeletal system across individuals, and not all anatomical atlases agree on the most representative set of insertion and origin points. The results presented here reflect how the musculoskeletal system was presented in the text from which it was constructed [ 19 ] and therefore provide only one possible network representation of the musculoskeletal system. To assess the reliability of our results across reasonable variation of the musculoskeletal configuration, we created a second musculoskeletal network from an alternate atlas [ 64 ]. Using this second atlas, we observed consistent results, and we report these complementary analyses in S3 Text .
It is also important to note that we mapped the first atlas [ 19 ] into a musculoskeletal graph composed of both bony and non-bony nodes. This choice equates the structural roles of bones and certain tendons and ligaments, which is admittedly a simplification of the biology. One justification for this simplification is that non-bony structures frequently serve as critical attachment points of muscles (i.e., the plantar fascia of the foot). Thus, it is reasonable to separate the musculoskeletal network into the two categories of muscles and structures that serve as muscular attachment points, as we did here. Nonetheless, this second category is quite heterogeneous in composition, and in future work, one could also consider constructing a multilayer graph, with a separate layer accounting for each type of muscular attachment structure. To confirm that our findings and interpretations are not significantly altered by the presence of non-bony muscular attachment points, we removed such points in an alternative atlas and observe that our main findings still hold (see S3 Text ).
To better understand the functional role of a single muscle within the interconnected musculoskeletal system, we implemented a physics-based model of the network’s impulse response properties by encoding the bones as point masses and the muscles as springs [ 65 ]. Significantly, this highly simplified model of the musculoskeletal system is able to identify important functional features. While muscles of high degree also tended to have a large impact on the network’s response ( Fig 3a ), there were several notable deviations from this trend ( Table 1 ).
The muscle noted to have the least impact relative to that expected is the orbicularis oculi, the muscle used for controlling movement of the eyelid. This muscle is small and relatively isolated in the body, originating and inserting on bones of the skull. The face muscles in general form a tight and isolated community, with few connections reaching outside that community. These factors likely contribute to the low impact of this muscle, and an analogous argument could be made for the remaining two muscles with less impact than expected, which are also muscles of the face.
The muscles with more impact than expected are more numerous but almost entirely located in the upper limb or upper limb girdle. The extensor carpi radialis longus, anconeus, brachioradialis, and brachialis muscles are all intrinsic arm muscles, the latter three acting at the elbow. All of these muscles may have higher impact than expected in a null model because they can either directly or indirectly affect the movement of the many bones of the wrist and hand. The observed high impact of these muscles could be a result of the fact that they control the movement of a limb, and at the end of the limb are many bones whose movement depends directly on these muscles. The remainder of the high-impact muscles, with the exception of the piriformis, all attach the upper limb to the axial skeleton. These muscles are the coracobrachialis, infraspinatus, supraspinatus, subscapularis, teres minor, teres major, and pectoralis major muscles. These muscles, like the previous four, have the property that they control the movement of an entire limb, which likely contributes to their impact. Unlike the previous group, these muscles also connect to the axial skeleton, which may also add to their impact. Many of these muscles originate on bones of the shoulder girdle and have the potential to affect all other shoulder girdle muscles, and potentially all bones connected to those muscles. This same dynamic likely exists in the lower limb, which is reflected by the presence of the piriformis muscle of the pelvic girdle. An in-depth discussion of how local network structure and muscle configuration may interact with impact deviation is presented in S7 Text . In addition to our work presented in the Supporting information, further insight may be gained into the properties of these outliers by performing experiments to closely examine the bones that are impacted most by each of these muscles.
While the network representation of a system can provide basic physical intuitions due to its parsimony and simplicity, it also remains agnostic to many details of the system’s architecture and function. It is a perennial question whether these first-principles models of complex systems can provide accurate predictions of real-world outcomes. We addressed this question by studying the relationship between the impact score of a muscle and the amount of time it takes for a person to recover from an injury. We quantified time of recovery by summing (i) the time to recover from the primary disability of the initial muscle injury and (ii) the time to recover from any secondary disabilities resulting from altered usage of other muscles in the network, due to the initial muscle injury [ 66 ]. We found that the deviation from the expected impact score in a null network correlated significantly with time of recovery ( Fig 3b ), supporting the notion that focal injury can have extended impacts on the body due to the inherently interconnected nature of the musculoskeletal system.
Indeed, muscular changes in one part of the body are known to affect other muscle groups. For example, strengthening hip muscles can lead to improved knee function following knee replacement [ 67 ]. Alteration of muscular function in the ankle following sprains can cause altered hip muscle function [ 68 , 69 ], a result replicated by our model (which found the biceps femoris and vastus lateralis were most impacted by ankle injury), and injury to limb muscles can lead to secondary injury of the diaphragm [ 70 ]. Our model offers a mathematically principled way in which to predict which muscles are more likely to have such a secondary impact on the larger musculoskeletal system and which muscles are at risk for secondary injury, given primary injury at a specific muscle site. It would be interesting in the future to test whether these predictions could inform beneficial adjustments to clinical interventions by explicitly taking the risk of secondary injury to particular muscles into account. Previously, prevention of secondary muscle injury has been largely relegated to cryotherapy [ 71 , 72 ] and has yet to be motivated by such a mechanistic model. Finally, an important question to ask is how this musculoskeletal configuration is evolutionarily advantageous and how evolutionary pressures may have optimized muscle impacts. Intuitively, one might expect that evolutionary pressures drive muscle impact down, perhaps by increasing muscular redundancy. A thorough investigation of the evolutionary advantages of the musculoskeletal network topology would be an interesting topic for future work.
Control of the human musculoskeletal system
Given the complexity of the musculoskeletal network and its critical role in human survival, it is natural to ask questions about how that network is controlled by the human brain. Indeed, the study of motor control has a long and illustrious history [ 73 ], which has provided important insights into how the brain is able to successfully and precisely make voluntary movements despite challenges such as redundancies, noise [ 74 ], delays in sensory feedback [ 75 ], environmental uncertainty [ 76 ], neuromuscular nonlinearity [ 77 ], and nonstationarity [ 78 ]. Here, we took a distinct yet complementary approach and asked how the topology of the musculoskeletal network may be mapped onto the topology of the motor strip within the cortex. We began by noting that the impact deviation of a muscle is positively correlated with the size of the cortical volume devoted to its control ( Fig 4c ). One interpretation of this relationship is that those muscles with greater impact than expected in a null model by their immediate connections tend to control more complex movements and therefore necessitate a larger number of neurons to manage those movements [ 79 ]. A second interpretation builds on an evolutionary argument that muscles with more impact need a greater redundancy in their control systems [ 80 ], and this redundancy takes the form of a greater cortical area.
Local cortical volumes aside [ 81 ], one might also wish to understand to what degree the larger-scale organization of the musculoskeletal network reflects the organization of the motor strip that controls it. Building on the recent application of community detection techniques to the study of skull anatomy [ 11 , 82 , 83 ], we reported the modular organization of the muscle network: groups of muscles in which the muscles in one group are more likely to connect to one another than to muscles in other groups. More intriguingly, we observed that muscle communities closely mimic the known muscle grouping of the motor strip ( Fig 1b , right): muscles that tend to connect to the same bones as each other also tend to be controlled by the same portion of the motor strip. Furthermore, a natural linear ordering of muscle communities—such that communities are placed close to one another on a line if they share network connections—mimics the order of control in the motor strip ( Fig 4d ). These results extend important prior work suggesting that the one-dimensional organization of the motor strip is related to both the structural and functional organization of the musculoskeletal network [ 84 , 85 ]. In fact, the results more specifically offer a network-level definition for optimal network control: the consistency of the linear map from musculoskeletal communities to motor strip communities.
Finally, we interrogated the physical locations of the cortical control of impactful muscles. We observed that muscles with more impact than expected given a null graph tend to be controlled by medial points on the motor strip, while muscles with less impact than expected tend to be controlled by lateral points on the motor strip ( Fig 4b ). This spatial specificity indicates that the organization of the motor strip is constrained by the physical layout of the body as well as aspects of how muscles function. Previous studies have examined a general temporal correspondence between cortical activity and muscle activity during movement [ 86 ], but little is known about topological correspondence.
Methodological considerations
The construction of a hypergraph from the human musculoskeletal system requires assumptions and simplifications that impact the flexibility of the current model. Most prominent is the reduction of the system into two categories: muscles and bones. These categories hold no additional information and therefore do not account for features of a muscle’s or bone’s internal architecture. This simplification introduces several limitations to the perturbative model, including the capability of modeling the functional architecture of complex muscles, or those with the ability to independently contract a subset of fibers. For example, the two-headed biceps brachii has an origin both on the scapula and supraglenoid tubercle, and it is possible to contract the fibers of one head separately from the fibers of the other head. Future work could extend our modeling framework to represent this complex functional architecture. Furthermore, nonmuscular soft tissue structures essential to the musculoskeletal system cannot be explicitly accounted for. These structures, including tendons and ligaments, can either be (1) encoded as bones, as in the main text network, or (2) excluded from the network, as in the supplement; neither option is completely anatomically accurate.
In the case of bones, the model is unable to account for bone–bone interactions (joints). The majority of muscles act at joints, and the exclusion of joints obfuscates the specific function of muscles. That is, the model accounts for the fact that muscles move bones but not how they move or in what direction. In the perturbative simulation, the lack of joint constraints allows bones to be placed at unnatural angles relative to adjacent bones. In addition, bones are modeled as point masses, which in the perturbative simulation may allow bones to undergo trajectories involving the passage through space that, in reality, is occupied by another bone. Future work could extend our modeling framework to account for these additional biophysical constraints.
Insights generated by this model are a result of the input data. As individual variation exists within the musculoskeletal system, it similarly exists in muscle impacts. We have made an effort to use two input datasets to justify our main findings, but these findings may not be generalizable to all healthy musculoskeletal configurations. Specifically, the degree of a muscle, subject to individual variation, is likely to affect the impact of that muscle. Exactly how normative individual variation in muscle degree is related to variation in predicted muscle impact is an important question that, nevertheless, is outside of the scope of the current study.
Lastly, the human musculoskeletal system is a complex and densely interconnected network. Neither muscles nor bones function as independent entities. As such, it is difficult to parse the function of a single muscle from effects due to surrounding muscles. Nonindependence of muscles can be partially eliminated by appropriate null model selection, and our results hold under a variety of choices. Nonetheless, the notion that muscles—and impact factors—are not truly independent should be considered when interpreting these results.
In summary, here we developed a novel network-based representation of the musculoskeletal system, constructed a mathematical modeling framework to predict recovery, and validated that prediction with data acquired from athletic injuries. Moreover, we directly linked the network structure of the musculoskeletal system to the organization of cortical architecture, suggesting an evolutionary pressure for optimal network control of the body. We compared the structure, function, and control of the human musculoskeletal system to a null system in which small groups of closely related muscles are rewired with each other. Our results suggest that the structure, function, and control of the musculoskeletal system are emergent from the highly detailed, small-scale organization, and when this small-scale organization is destroyed, so are the emergent features. Our work directly motivates future studies to test whether faster recovery may be attained by not only focusing rehabilitation on the primary muscle injured but also directing efforts towards muscles that the primary muscle impacts. Furthermore, our work supports the development of a predictive framework to determine the extent of musculoskeletal repercussions from insults to the primary motor cortex. An important step in the network science of clinical medicine [ 87 ], our results inform the attenuation of secondary injury and the hastening of recovery.
Supporting information
S1 text. alternative null models..
This text file details construction of the alternative null models.
https://doi.org/10.1371/journal.pbio.2002811.s001
S2 Text. Resolution of community detection.
This file provides a description of the choice of community detection resolution parameter.
https://doi.org/10.1371/journal.pbio.2002811.s002
S3 Text. Alternative musculoskeletal network.
This file provides a description of the alternative musculoskeletal network.
https://doi.org/10.1371/journal.pbio.2002811.s003
S4 Text. Bone dynamics resulting from muscle perturbation.
https://doi.org/10.1371/journal.pbio.2002811.s004
S5 Text. Accounting for bone weights and muscle strengths.
https://doi.org/10.1371/journal.pbio.2002811.s005
S6 Text. Nonathlete muscle recovery analysis.
https://doi.org/10.1371/journal.pbio.2002811.s006
S7 Text. Local network structure and impact deviation.
https://doi.org/10.1371/journal.pbio.2002811.s007
S1 Table. Muscles with greater and lesser impact than expected in randomly rewired hypergraphs.
This null model required randomly rewiring muscles within the hypergraph, preserving degree. The muscles on the left side have less impact than expected, given their hyperedge degree: their impacts are more than 1.96 standard deviations below the mean, indicating that they lie outside the 95% CI of the distribution. The muscles on the right side have more impact than expected, given their hyperedge degree: their impacts are more than 1.96 standard deviations above the mean, ordered from most to least extreme. This table shows the muscles that had the greatest positive and greatest negative difference in impact, relative to degree-matched controls.
https://doi.org/10.1371/journal.pbio.2002811.s008
S2 Table. Homunculus categories, the member muscles of which either all have more impact than expected or all have less impact than expected, compared to randomly rewired hypergraphs.
This null model required randomly rewiring muscles within the hypergraph, preserving degree. Categories on the left are composed entirely of muscles with less impact than expected, compared to degree-matched controls. Categories on the right are composed entirely of muscles with more impact than expected, compared to degree-matched controls.
https://doi.org/10.1371/journal.pbio.2002811.s009
S3 Table. Muscles with greater and lesser impact than expected in hypergraphs randomly rewired within their homunculus category.
This null model required randomly rewiring muscles within their homunculus category, preserving degree. The muscles on the left side have less impact than expected, given their hyperedge degree: their impacts are more than 1.96 standard deviations below the mean, indicating that they lie outside the 95% CI of the distribution. The muscles on the right side have more impact than expected, given their hyperedge degree: their impacts are more than 1.96 standard deviations above the mean, ordered from most to least extreme. This table shows the muscles that had the greatest positive and greatest negative difference in impact, relative to degree-matched controls.
https://doi.org/10.1371/journal.pbio.2002811.s010
S4 Table. Homunculus categories, member muscles of which either all have more impact than expected or all have less impact than expected, compared to hypergraphs randomly rewired within their homunculus category.
This null model required randomly rewiring muscles within their homunculus category, preserving degree. Categories on the left are composed entirely of muscles with less impact than expected, compared to degree-matched controls. Categories on the right are composed entirely of muscles with more impact than expected, compared to degree-matched controls.
https://doi.org/10.1371/journal.pbio.2002811.s011
S5 Table. Muscles with greater and lesser impact than expected in a random hypergraph.
This null model required randomly assigning muscle–bone connections, only preserving overall degree and not individual muscle degree. The muscles on the left side have less impact than expected, given their hyperedge degree: their impacts are more than 1.96 standard deviations below the mean, indicating that they lie outside the 95% CI of the distribution. The muscles on the right side have more impact than expected, given their hyperedge degree: their impacts are more than 1.96 standard deviations above the mean and are ordered from most to least extreme.
https://doi.org/10.1371/journal.pbio.2002811.s012
S6 Table. Volumes of muscles of the leg sub-network.
Here, we include the muscle name (column 1), the muscle volume (in cm 3 ; column 2), and the reference from which the estimate was taken.
https://doi.org/10.1371/journal.pbio.2002811.s013
S7 Table. Masses of the bones of the leg sub-network.
Here, we include the bone name (column 1), the bone mass (in g; column 2), and the reference from which the estimate was taken.
https://doi.org/10.1371/journal.pbio.2002811.s014

S8 Table. The assigned homunculus categories and data-driven community assignments of muscles.
Also available at DOI: 10.5281/zenodo.1069104 .
https://doi.org/10.1371/journal.pbio.2002811.s015
S9 Table. The hypergraph of muscles and bones from the Hosford Muscle tables [ 18 ], used in the main text.
https://doi.org/10.1371/journal.pbio.2002811.s016
S10 Table. The hypergraph of muscles and bones from Grant’s atlas [ 64 ], used in the supplementary text.
https://doi.org/10.1371/journal.pbio.2002811.s017
S1 Fig. Community detection with differing resolution parameters.
This figure illustrates how the selection of the resolution parameter during community detection will change the number and size of communities detected. As the resolution parameter is increased, the size of individual communities decreases, while the number of communities increases. (a-d) Community detection for the muscle-centric network, using γ values of 1, 2, 8, and 16, respectively. The final community structure for each γ is a consensus partition of 100 individual runs of the community detection algorithm.
https://doi.org/10.1371/journal.pbio.2002811.s018
S2 Fig. Community detection with differing resolution parameters.
This figure illustrates stability around the chosen tuning parameter of γ = 4.3. Here, we explore partitions generated from nearby resolution parameters γ = 4.2 and γ = 4.4. Visually, the three partitions appear to have similar structure. The two nearby partitions are also mathematically similar, with z-score of the Rand coefficient [ 47 ] z Rand (γ = 4.2, γ = 4.3) = 105, z Rand (γ = 4.3, γ = 4.4) = 110, and z Rand (γ = 4.2, γ = 4.4) = 105. The final community structure for each γ is a consensus partition of 100 individual runs of the community detection algorithm.
https://doi.org/10.1371/journal.pbio.2002811.s019
S3 Fig. Visual comparison of null models.
This figure illustrates the differences in the null bipartite graphs. (A) The original unpermuted muscle–bone bipartite graph. (B) The random null bipartite graph. (C) The randomly rewired bipartite graph. (D) The in-community randomly rewired bipartite graph used in the main text, which permutes topology locally while preserving global topology.
https://doi.org/10.1371/journal.pbio.2002811.s020
S4 Fig. Main results as a function of the null model.
Here, we show results using a random hypergraph model or a rewired (permuted) hypergraph model that does not maintain local connections. (A) The impact score plotted as a function of the hyperedge degree for random hypergraphs and the observed musculoskeletal hypergraph. (B) The impact score plotted as a function of the hyperedge degree for permuted hypergraphs and the observed musculoskeletal hypergraph. (C) Deviation ratio correlates significantly with homuncular category (F(1,19) = 6.67, p = 0.018, R 2 = 0.26), decreasing from medial (area 0) to lateral (area 22) using a random hypergraph null model. (D) Deviation ratio correlates significantly with homuncular category (F(1,19) = 6.86, p = 0.017, R 2 = 0.26), decreasing from medial (area 0) to lateral (area 22) using a permuted hypergraph null model. (E) Impact score deviation significantly correlates with motor strip activation area (F(1,5) = 13.4, p = 0.014, R 2 = 0.72) using a random hypergraph null model. Data points are sized according to the number of muscles required for the particular movement. (F) Impact score deviation significantly correlates with motor strip activation area (F(1,5) = 13.7, p = 0.022, R 2 = 0.73) using a permuted hypergraph null model. Data points are sized according to the number of muscles required for the particular movement. (G) Impact score deviation correlates with muscle recovery time following injury to muscles or muscle groups (F(1,11) = 64.5, p = 6.3 × 10 −6 , R 2 = 0.85), using a random hypergraph null model. Data points are scaled according to the number of muscles included. (H) Impact score deviation correlates with muscle recovery time following injury to muscles or muscle groups (F(1,11) = 70.5, p < 0.0001, R 2 = 0.86), more so than expected in a permutation-based hypergraph null model. Data points are scaled according to the number of muscles included. Data available at DOI: 10.5281/zenodo.1069104 .
https://doi.org/10.1371/journal.pbio.2002811.s021
S5 Fig. Network topology and the homunculus.
Linear muscle coordinates determined using multidimensional scaling without thresholding via a weighted distance matrix (calculated using distance_wei.m included in the Brain Connectivity Toolbox, https://sites.google.com/site/bctnet/ ). Without thresholding, a significant correlation also exists between linear muscle coordinate and homunculus area (F(1,268) = 303, p < 0.0001, R 2 = 0.53). Data available at DOI: 10.5281/zenodo.1069104 .
https://doi.org/10.1371/journal.pbio.2002811.s022
S6 Fig. Probing musculoskeletal function for an alternate network.
(a) The impact score plotted as a function of the hyperedge degree for a null hypergraph model and the observed musculoskeletal hypergraph. (b) Impact score deviation correlates with muscle recovery time following injury to muscles or muscle groups (F(1,12) = 40.2, p < 0.0001, R 2 = 0.77). Shaded areas indicate 95% CIs, and data points are scaled according to the number of muscles included. Data available at DOI: 10.5281/zenodo.1069104 .
https://doi.org/10.1371/journal.pbio.2002811.s023
S7 Fig. Probing musculoskeletal control for an alternate network.
(a) Deviation ratio is significantly correlated with homuncular topology (F(1,18) = 8.88, R 2 = 0.33, p = 0.0080), decreasing from medial (area 0) to lateral (area 22) regions. (b) Impact score deviation is significantly correlated with motor strip activation area (F(1,5) = 23.4, R 2 = 0.82, p = 0.005). Data points are sized according to the number of muscles required for the particular movement. Data available at DOI: 10.5281/zenodo.1069104 .
https://doi.org/10.1371/journal.pbio.2002811.s024
S8 Fig. Dynamics of biceps brachii perturbation.
This figure shows the movement of the clavicle, as well as a bone of the finger and toe, in response to the perturbation of the biceps brachii. Data available at DOI: 10.5281/zenodo.1069104 .
https://doi.org/10.1371/journal.pbio.2002811.s025
S9 Fig. Comparing models with and without bone weights and muscle strengths.
The impact of the leg muscles was calculated with and without the addition of anatomical values for bone weight and muscle volume. These impacts were found to be significantly correlated with one another (F(1,25) = 6.83, R 2 = 0.0214, p = 0.015), suggesting that at least in some portions of the body, our simplified network representation provides a reasonable approximation for more biophysically accurate network representations. Data available at DOI: 10.5281/zenodo.1069104 .
https://doi.org/10.1371/journal.pbio.2002811.s026
S10 Fig. Probing musculoskeletal function for nonathletes.
Recovery times were gathered for injuries to various muscles of nonathletes. We observed a significant correlation between muscle recovery time and impact deviation (F(1,14) = 5.02, R 2 = 0.264, p = 0.041). Data available at DOI: 10.5281/zenodo.1069104 .
https://doi.org/10.1371/journal.pbio.2002811.s027
S11 Fig. Correspondence of network topology and system function.
Network topology, specifically average shortest path length, is significantly negatively correlated with the impact score estimated from the perturbative simulations of system dynamics (F(1,268) = 65.1, R 2 = −0.4422, p < 0.0001). Data available at DOI: 10.5281/zenodo.1069104 .
https://doi.org/10.1371/journal.pbio.2002811.s028
S12 Fig. Relation between musculoskeletal variation and muscular impact across two musculoskeletal networks.
Here, we compare the percent change in impact score and degree for each muscle between the musculoskeletal network reported in the main text and that reported in the supplementary text. We observe that the impact score of muscles is more affected by larger changes in degree than by smaller changes in degree (F(1,268) = 5.76, R = 0.1450, p = 0.017). Data available at DOI: 10.5281/zenodo.1069104 .
https://doi.org/10.1371/journal.pbio.2002811.s029
S13 Fig. Alternative perturbative approach.
To establish a measure of impact per muscle hyperedge, objects were displaced into a fourth spatial dimension to avoid making arbitrary choices within three dimensions. An alternative approach would be to perturb each muscle in each of three orthogonal directions, calculating impact each time and calculating the vector sum of these three results. To answer the question of how these two approaches compare, we performed this experiment on the muscle-bone bipartite matrix to create two 270 × 1 vectors, one encoding the impact scores via displacement in the fourth dimension, and one encoding the vector sum of the three orthogonal displacements. The two vectors were significantly correlated with each other (F(1,268) = 1590, R 2 = 0.856, p < 0.0001).
https://doi.org/10.1371/journal.pbio.2002811.s030
Acknowledgments
We thank orthopedic surgeon John F. Perry, M.D., and professor of orthopedics Robert Mauck, M.D., for helpful comments on earlier versions of the manuscript. We also acknowledge the Penn Network Visualization Program. The content is solely the responsibility of the authors and does not necessarily represent the official views of any of the funding agencies.
- 1. Bynum WF. Companion Encyclopedia of the History of Medicine. Routledge; 1997.
- View Article
- PubMed/NCBI
- Google Scholar
- 3. Craver CF. Explaining the Brain: Mechanisms and the Mosaic Unity of Neuroscience. Oxford University Press; 2009.
- 4. Neumann DA. Kinesiology of the musculoskeletal system: foundations for rehabilitation. Elsevier Health Sciences; 2013.
- 5. Newman MEJ. Networks: An Introduction. Oxford University Press; 2010.
- 6. Bollobás B. Random graphs. In: Modern Graph Theory. Springer; 1998. p. 215–252.
- 8. Kolaczyk E. Statistical Analysis of Network Data, volume 69 of Springer Series in Statistics. Springer New York. 2009.
- 9. Newman M, Barabasi AL, Watts DJ. The structure and dynamics of networks. Princeton University Press; 2011.
- 14. Murai A, Yamane K, Nakamura Y. Modeling and identification of human neuromusculoskeletal network based on biomechanical property of muscle. 30th Annual International Conference of the IEEE Engineering in Medicine and Biology Society. 2008.
- 18. Hosford D. The Hosford Muscle Tables: Skeletal Muscles of the Human Body; 1998. http://www.ptcentral.com/muscles .
- 19. McMinn RMH, Hutchings RT, Pegington J, Abrahams P. Color atlas of human anatomy. 3rd ed. Mosby Year Book; 1993.
- 21. Jutla IS, Jeub LGS, Mucha PJ. A generalized Louvain method for community detection implemented in MATLAB; 2011–2012. http://netwiki.amath.unc.edu/GenLouvain .
- 25. Betzel RF, Medaglia JD, Bassett DS. Diversity of meso-scale architecture in human and non-human connectomes. Nature Communications, 2018, in Press.
- 31. Torg JS. Athletic injuries to the head, neck, and face. In: Concepts of Athletic Training. Philadelphia, PA: Lea and Febiger; 1982.
- 43. Penfield W, Rasmussen T. The cerebral cortex of man. The Macmillan Company; 1950.
- 48. Wang J. In: Classical Multidimensional Scaling. Berlin, Heidelberg: Springer Berlin Heidelberg; 2011. p. 115–129.
- 51. Riedl R. Order in living organisms: a systems analysis of evolution. Wiley; 1978.
- 52. West DB. Introduction to graph theory. Vol 2. Upper Saddle River: Prentice hall; 2001.
- 64. Agur AM, Dalley AF. Grant’s atlas of anatomy. Lippincott Williams & Wilkins; 2013.

- / Professionals
- / The Human Body in Research
Systems of the Human Body in Research and Education
Virtually every organ, tissue and system of the human body is needed for medical research, and nearly every disease that plagues mankind is being studied with the help of donated human organs and tissues.
Many organs act as the focal points of regenerative medicine, such as organs being decellularized to rid them of either diseased or potentially incompatible cells and later recellularized with a recipient’s own cells for eventual transplantation.
Virtually all organs are studied to identify precursors to a multitude of diseases. Through this incredible research, preventative care can be introduced to a patient well before their organs begin to fail and they face the need for an organ transplant or, in extreme circumstances, death.
Please see the downloadable diagram below for the complete list of organs and tissues for research available through IIAM.

Used to study the influence of hormones on cardiovascular disease.

For studies on the congenital defects of the kidney and urinary tract as a major cause of pediatric renal failure. The bladder is also studied to test new compounds to cure urinary incontinence.

For studies of genetic markers, inflammatory diseases and central nervous system disorders.

Heart, Aorta, Arteries and Veins
Medical investigators use the human heart to learn about the development of atherosclerosis in coronary arteries. These and other blood vessels are used to find ways to control clot formation and high blood pressure. Ground-breaking research for bypass procedures and the development of plaque-removal devices and improved imaging methods is also underway.

Renal tissue is used to study the toxicity of anti-cancer therapies, for example, and to study the safe concentrations of other drugs in development. Kidneys are also used to identify new biomarkers to determine their transplantability and to produce safer cold preservation methods.

The liver is responsible for most drug and chemical metabolism, and is the site of drug-drug interaction and toxicity. IIAM has been a leader in providing whole, human livers to help researchers understand how human livers respond to various drugs, to develop effective screening assays for anti-HCV drugs, and to reduce researchers’ reliance on animal testing.

Research into chronic obstructive pulmonary disease (COPD), cystic fibrosis, asthma, emphysema and allergies are advanced by the use of human lung tissues. In addition, researchers are testing the potential for new drugs to cause broncho-constriction of airways thus preventing possible life-threatening events.

Diabetes researchers rely on pancreatic tissues to explore the regulation of insulin production, to identify the genetic components of the disease and for toxicity studies on new compounds. Researchers are also seeking ways to prevent and cure Types I & II Diabetes. Transplantation of islet cells, the site of insulin production, is undergoing clinical trials with very promising results.

Researchers examining human skin to study comparative rates of drug absorption have developed such novel approaches as transdermal or “patch” delivery, as well as topical applications, for a variety of drug types.

As a prolific source of T-cells and B-cells, the human spleen is used by researchers to investigate AIDS and other autoimmune diseases and for tolerance studies.

Utilized in the study of gastro-esophageal reflux diseases (GERD), and to explore the side effects of new drugs for gastric disorders.

For antibody studies on human T-cells.
Professional Quick Links
- PROFESSIONALS
- The Human Body in Research
- Screening and Recovery Process
- Neonatal Donation FAQ
- Recovery Workshops
- Recovery Workshop Testimonials
- Education and Information
- Organ Placement Factors
24 Hour Service
Find out more, learn more about the research being done with referred organs and tissues..
The Fascinating Science Behind the Body Farm
This essay is about the concept of “body farms” and their crucial role in forensic science and anthropology. It explains how these research facilities, first established by Dr. William Bass in 1981, help study human decomposition under various conditions to improve the accuracy of post-mortem interval (PMI) estimations. The research conducted at body farms enhances forensic methodologies and provides valuable insights into human taphonomy, benefiting both modern criminal investigations and the understanding of ancient human remains.
How it works
Concept of “farm of body”, presumably, foremost causes inconvenience, however these experience resources, what is specialized, obligatory for understanding of human processes of time-table. It is formally known how the judicial centers of research of anthropology, the farms of body are central in the help of judicial researches, identifying bits and pieces, and our advancing penetrating in changes on post mortem.
The introductory farm of body was set by Doctor William Bass in 1981 in University of Tennessee in Knoxville. But opening initiative appeared from a necessity to the detailed data on a human time-table, excelling previous studies, certain on feral models, what inadequate mirror human processes of disintegration.
These resources are foremost concentrated on research, how an ecological alike for variables temperature, humidity, and curriculum of man of action of activity of insect.
In the farms of body, sacrificed human bodies test different scenarios, to simulate the real terms. Some bodies pochowane, second left it is proposed on earth, some submerged in water, and second limited in mechanizations. Researchers of meticulously phase of curriculum of document, collecting central data, beginning critical for an estimation posthumous interval (Pmi) of -the time death. This research refined Pmi of method of determination substantially, substantial in judicial researches.
One of leading additions of farms of body is their role exactly, estimating Pmi. Calculating time, what flows since death is inalienable complicated from numberless influences. The farms of body provide a settlement that is managed, to insulate and to study these variables, bringing more exact Pmi over technique of estimation. Therefore, judicial definiteness of certificate in criminal cases is very propped up.
Additionally, farms of body considerably benefit anthropology, especially in the study of human taphonomy processes, what influences on an organism from death to become numb. Carefully the corresponding penetrating helps archaeologists understand better, how natural terms influence on maintenance of bits’ and pieces of man, thus interpretations of cleaning of old human places.
While the concept of farms of body, presumably, disturbs something, their deep holding to science and society is indisputable. Investigate moves in these resources not only helps in the decision of modern criminal cases but and deepens our understanding to human biology and time-table. Eventually, this work supports a justice and provides a halt for families of deceased.
Thus, farms of body are obligatory experience resources central to judicial science and anthropology. Studying a human time-table on condition of various, these centers do critical data that increase the estimation of Pmi, refine judicial methodologies, and translate critical studies for specialists. Without regard to their sullen nature, the farms of body extend our understanding of death and disintegration, with far-reaching values how for scientific knowledge, so and social benefit.

Cite this page
The Fascinating Science Behind the Body Farm. (2024, Jun 28). Retrieved from https://papersowl.com/examples/the-fascinating-science-behind-the-body-farm/
"The Fascinating Science Behind the Body Farm." PapersOwl.com , 28 Jun 2024, https://papersowl.com/examples/the-fascinating-science-behind-the-body-farm/
PapersOwl.com. (2024). The Fascinating Science Behind the Body Farm . [Online]. Available at: https://papersowl.com/examples/the-fascinating-science-behind-the-body-farm/ [Accessed: 1 Jul. 2024]
"The Fascinating Science Behind the Body Farm." PapersOwl.com, Jun 28, 2024. Accessed July 1, 2024. https://papersowl.com/examples/the-fascinating-science-behind-the-body-farm/
"The Fascinating Science Behind the Body Farm," PapersOwl.com , 28-Jun-2024. [Online]. Available: https://papersowl.com/examples/the-fascinating-science-behind-the-body-farm/. [Accessed: 1-Jul-2024]
PapersOwl.com. (2024). The Fascinating Science Behind the Body Farm . [Online]. Available at: https://papersowl.com/examples/the-fascinating-science-behind-the-body-farm/ [Accessed: 1-Jul-2024]
Don't let plagiarism ruin your grade
Hire a writer to get a unique paper crafted to your needs.
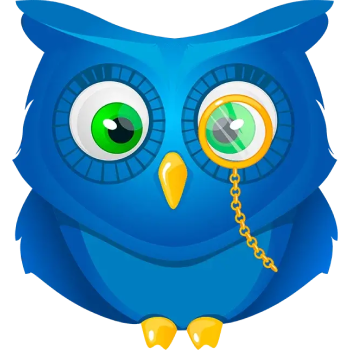
Our writers will help you fix any mistakes and get an A+!
Please check your inbox.
You can order an original essay written according to your instructions.
Trusted by over 1 million students worldwide
1. Tell Us Your Requirements
2. Pick your perfect writer
3. Get Your Paper and Pay
Hi! I'm Amy, your personal assistant!
Don't know where to start? Give me your paper requirements and I connect you to an academic expert.
short deadlines
100% Plagiarism-Free
Certified writers
Department of Cognitive Science
Recent graduate’s paper published in pnas.
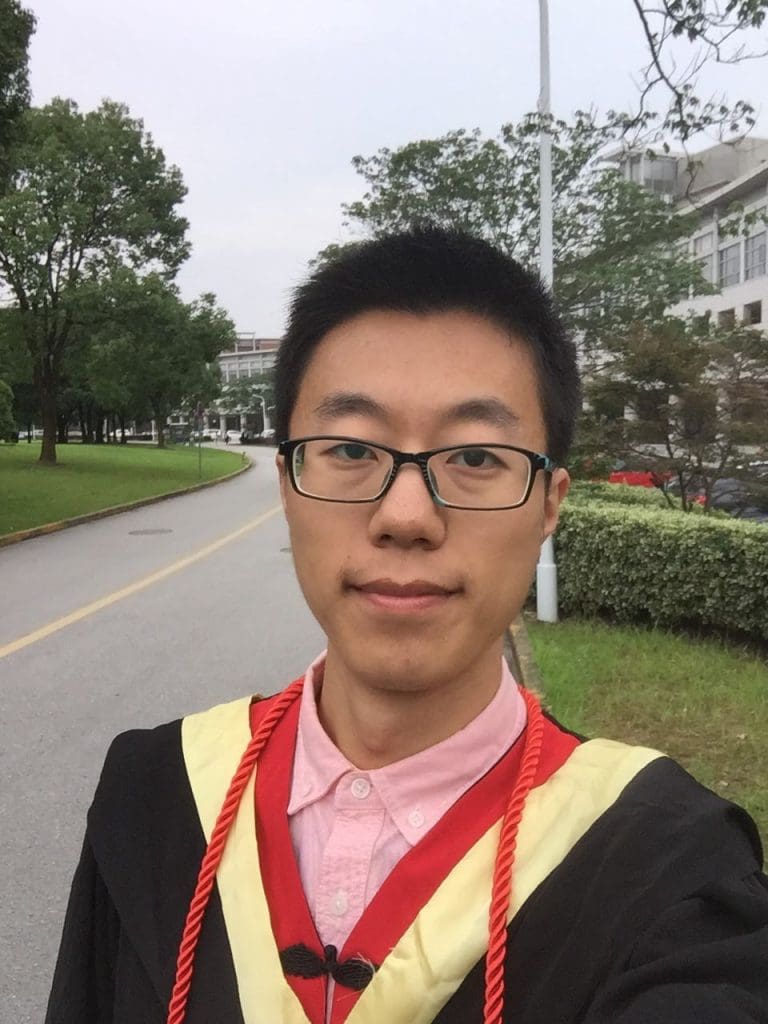
Hongru Zhu (PhD ’23) published a paper with Prof. Daniel Kersten (UMN) on Natural scenes reveal diverse representations of 2D and 3D body pose in the human brain in Proceedings of the National Academy of Sciences based on research he started as a student in our program.
https://www.pnas.org/doi/10.1073/pnas.2317707121

An official website of the United States government
The .gov means it’s official. Federal government websites often end in .gov or .mil. Before sharing sensitive information, make sure you’re on a federal government site.
The site is secure. The https:// ensures that you are connecting to the official website and that any information you provide is encrypted and transmitted securely.
- Publications
- Account settings
Preview improvements coming to the PMC website in October 2024. Learn More or Try it out now .
- Advanced Search
- Journal List
- J Res Med Sci
- v.19(2); 2014 Feb
Review on iron and its importance for human health
Nazanin abbaspour.
Department of Environmental Systems Science, Institute of Terrestrial Ecosystem, Swiss Federal Institute of Technology, Zurich, Switzerland
Richard Hurrell
1 Department of Health Sciences and Technology, Laboratory of Human Nutrition, Institute of Food, Nutrition and Health, Swiss Federal Institute of Technology, Zurich, Switzerland
Roya Kelishadi
2 Child Growth and Development Research Center, Isfahan University of Medical Sciences, Isfahan, Iran
It is well-known that deficiency or over exposure to various elements has noticeable effects on human health. The effect of an element is determined by several characteristics, including absorption, metabolism, and degree of interaction with physiological processes. Iron is an essential element for almost all living organisms as it participates in a wide variety of metabolic processes, including oxygen transport, deoxyribonucleic acid (DNA) synthesis, and electron transport. However, as iron can form free radicals, its concentration in body tissues must be tightly regulated because in excessive amounts, it can lead to tissue damage. Disorders of iron metabolism are among the most common diseases of humans and encompass a broad spectrum of diseases with diverse clinical manifestations, ranging from anemia to iron overload, and possibly to neurodegenerative diseases. In this review, we discuss the latest progress in studies of iron metabolism and bioavailability, and our current understanding of human iron requirement and consequences and causes of iron deficiency. Finally, we discuss strategies for prevention of iron deficiency.
INTRODUCTION
From ancient times, man has recognized the special role of iron in health and disease.[ 1 ] Iron had early medicinal uses by Egyptians, Hindus, Greeks, and Romans.[ 2 , 3 ] During the 17 th century, iron was used to treat chlorosis (green disease), a condition often resulting from the iron deficiency.[ 4 ] However, it was not until 1932 that the importance of iron was finally settled by the convincing proof that inorganic iron was needed for hemoglobin synthesis.[ 5 ] For many years, nutritional interest in iron focused on its role in hemoglobin formation and oxygen transport.[ 6 ] Nowadays, although low iron intake and/or bioavailability are responsible for most anemia in industrialized countries, they account for only about half of the anemia in developing countries,[ 7 ] where infectious and inflammatory diseases (especially malaria), blood loss from parasitic infections, and other nutrient deficiencies (vitamin A, riboflavin, folic acid, and vitamin B12) are also important causes.[ 8 ]
Biochemistry and physiology
In contrast to zinc, iron is an abundant element on earth[ 2 , 9 ] and is a biologically essential component of every living organism.[ 10 , 11 ] However, despite its geologic abundance, iron is often a growth limiting factor in the environment.[ 9 ] This apparent paradox is due to the fact that in contact with oxygen iron forms oxides, which are highly insoluble, and thus is not readily available for uptake by organisms.[ 2 ] In response, various cellular mechanisms have evolved to capture iron from the environment in biologically useful forms. Examples are siderophores secreted by microbes to capture iron in a highly specific complex[ 12 ] or mechanisms to reduce iron from the insoluble ferric iron (Fe +3 ) to the soluble ferrous form (Fe +2 ) as in yeasts.[ 13 ] Many of the mechanisms found in lower organisms, have analogous counterparts in higher organisms, including humans. In the human body, iron mainly exists in complex forms bound to protein (hemoprotein) as heme compounds (hemoglobin or myoglobin), heme enzymes, or nonheme compounds (flavin-iron enzymes, transferring, and ferritin).[ 3 ] The body requires iron for the synthesis of its oxygen transport proteins, in particular hemoglobin and myoglobin, and for the formation of heme enzymes and other iron-containing enzymes involved in electron transfer and oxidation-reductions.[ 14 , 3 ] Almost two-thirds of the body iron is found in the hemoglobin present in circulating erythrocytes, 25% is contained in a readily mobilizable iron store, and the remaining 15% is bound to myoglobin in muscle tissue and in a variety of enzymes involved in the oxidative metabolism and many other cell functions.[ 15 ]
Iron is recycled and thus conserved by the body. Figure 1 shows a schematic diagram of iron cycle in the body. Iron is delivered to tissues by circulating transferrin, a transporter that captures iron released into the plasma mainly from intestinal enterocytes or reticuloendothelial macrophages. The binding of iron-laden transferrin to the cell-surface transferrin receptor (TfR) 1 results in endocytosis and uptake of the metal cargo. Internalized iron is transported to mitochondria for the synthesis of heme or iron-sulfur clusters, which are integral parts of several metalloproteins, and excess iron is stored and detoxified in cytosolic ferritin.

Iron is bound and transported in the body via transferrin and stored in ferritin molecules. Once iron is absorbed, there is no physiologic mechanism for excretion of excess iron from the body other than blood loss, that is, pregnancy, menstruation, or other bleeding
The fraction of iron absorbed from the amount ingested is typically low, but may range from 5% to 35% depending on circumstances and type of iron.[ 3 ]
Iron absorption occurs by the enterocytes by divalent metal transporter 1, a member of the solute carrier group of membrane transport proteins. This takes place predominantly in the duodenum and upper jejunum.[ 16 ] It is then transferred across the duodenal mucosa into the blood, where it is transported by transferrin to the cells or the bone marrow for erythropoiesis [producing red blood cells (RBCs)].[ 14 , 17 , 18 ] A feedback mechanism exists that enhances iron absorption in people who are iron deficient. In contrast, people with iron overload dampen iron absorption via hepcidin. It is now generally accepted that iron absorption is controlled by ferroportin which allows or does not allow iron from the mucosal cell into the plasma.
The physical state of iron entering the duodenum greatly influences its absorption. At physiological pH, ferrous iron (Fe +2 ) is rapidly oxidized to the insoluble ferric (Fe +3 ) form. Gastric acid lowers the pH in the proximal duodenum reducing Fe +3 in the intestinal lumen by ferric reductases, thus allowing the subsequent transport of Fe +2 across the apical membrane of enterocytes. This enhances the solubility and uptake of ferric iron. When gastric acid production is impaired (for instance by acid pump inhibitors such as the drug, prilosec), iron absorption is reduced substantially.
Dietary heme can also be transported across the apical membrane by a yet unknown mechanism and subsequently metabolized in the enterocytes by heme oxygenase 1 (HO-1) to liberate (Fe +2 ).[ 19 ] This process is more efficient than the absorption of inorganic iron and is independent of duodenal pH. It is thus not influenced by inhibitors such as phytate and polyphenols. Consequently, red meats high in hemoglobin are excellent nutrient sources of iron. Directly internalized Fe +2 is processed by the enterocytes and eventually (or not) exported across the basolateral membrane into the bloodstream via Fe +2 transporter ferroportin. The ferroportin-mediated efflux of Fe +2 is coupled by its reoxidation to Fe +2 , catalyzed by the membrane-bound ferroxidase hephaestin that physically interacts with ferroportin[ 20 ] and possibly also by its plasma homologue ceruloplasmin. Exported iron is scavenged by transferrin, which maintains Fe +3 in a redox-inert state and delivers it into tissues. The total iron content of transferrin (≈3 mg) corresponds to less than 0.1% of body iron, but it is highly dynamic and undergoes more than 10 times daily turnover to sustain erythropoiesis. The transferrin iron pool is replenished mostly by iron recycled from effete RBCs and, to a lesser extent, by newly absorbed dietary iron. Senescent RBCs are cleared by reticuloendothelial macrophages, which metabolize hemoglobin and heme, and release iron into the bloodstream. By analogy to intestinal enterocytes, macrophages export Fe +2 from their plasma membrane via ferroportin, in a process coupled by reoxidation of Fe +2 to Fe +3 by ceruloplasmin and followed by the loading of Fe +3 to transferrin.[ 21 ]
Theil et al .,[ 21 ] recently reported that an independent mechanism also exists for the absorption of plant ferritins mostly present in legumes. However, the relevance of the ferritin transporter is unclear as most ferritin seems to be degraded during food processing and digestion, thereby releasing inorganic iron from the ferritin shell for absorption by the normal mechanism.[ 22 ] As one ferritin molecule contains 1000 or more iron atoms, and should also be unaffected by iron absorption inhibitors, such a mechanism would provide an important source of iron in the developing world where legumes are commonly consumed.
Regulation of iron homeostasis
Since iron is required for a number of diverse cellular functions, a constant balance between iron uptake, transport, storage, and utilization is required to maintain iron homeostasis.[ 11 ] As the body lacks a defined mechanism for the active excretion of iron, iron balance is mainly regulated at the point of absorption.[ 23 , 24 ]
Hepcidin is a circulating peptide hormone secreted by the liver that plays a central role in the regulation of iron homeostasis. It is the master regulator of systemic iron homeostasis, coordinating the use and storage of iron with iron acquisition.[ 25 ] This hormone is primarily produced by hepatocytes and is a negative regulator of iron entry into plasma [ Figure 2 ]. Hepcidin acts by binding to ferroportin, an iron transporter present on cells of the intestinal duodenum, macrophages, and cells of the placenta. Binding of hepcidin induces ferroportin internalization and degradation.[ 26 ] The loss of ferroportin from the cell surface prevents iron entry into plasma [ Figure 2a ]. Decreased iron entry into plasma results in low transferrin saturation and less iron is delivered to the developing erythroblast. Conversely, decreased expression of hepcidin leads to increased cell surface ferroportin and increased iron absorption[ 27 ] [ Figure 2c ]. In all species, the concentration of iron in biological fluids is tightly regulated to provide iron as needed and to avoid toxicity, because iron excess can lead to the generation of reactive oxygen species.[ 28 ] Iron homeostasis in mammals is regulated at the level of intestinal absorption, as there is no excretory pathway for iron.

Hepcidin-mediated regulation of iron homeostasis. (a) Increased hepcidin expression by the liver results from inflammatory stimuli. High levels of hepcidin in the bloodstream result in the internalization and degradation of the iron exporter ferroportin. Loss of cell surface ferroportin results in macrophage iron loading, low plasma iron levels, and decreased erythropoiesis due to decreased transferrin-bound iron. The decreased erythropoiesis gives rise to the anemia of chronic disease. (b) Normal hepcidin levels, in response to iron demand, regulate the level of iron import into plasma, normal transferrin saturation, and normal levels of erythropoiesis. (c) Hemochromatosis, or iron overload, results from insufficient hepcidin levels, causing increased iron import into plasma, high transferrin saturation, and excess iron deposition in the liver. Source: De Domenico, et al .[ 27 ]
Plasma hepcidin levels are regulated by different stimuli, including cytokines, plasma iron, anemia, and hypoxia. Dysregulation of hepcidin expression results in iron disorders. Overexpression of hepcidin leads to the anemia of chronic disease, while low hepcidin production results in hereditary hemochromatosis (HFE) with consequent iron accumulation in vital organs [ Figure 2 ]. Most hereditary iron disorders result from inadequate hepcidin production relative to the degree of tissue iron accumulation. Impaired hepcidin expression has been shown to result from mutations in any of 4 different genes: TfR2, HFE, hemochromatosis type 2 (HFE2), and hepcidin antimicrobial peptide (HAMP). Mutations in HAMP, the gene that encodes hepcidin, result in iron overload disease, as the absence of hepcidin permits constitutively high iron absorption. The role for other genes (TFR2, HFE, and HFE2) in the regulation of hepcidin production has been unclear.[ 27 ]
Ferritin concentration together with that of hemosiderin reflects the body iron stores. They store iron in an insoluble form and are present primarily in the liver, spleen, and bone marrow.[ 2 ] The majority of iron is bound to the ubiquitous and highly conserved iron-binding protein, ferritin.[ 18 ] Hemosiderin is an iron storage complex that less readily releases iron for body needs. Under steady state conditions, serum ferritin concentrations correlate well with total body iron stores.[ 29 ] Thus, serum ferritin is the most convenient laboratory test to estimate iron stores.
Apart from iron losses due to menstruation, other bleeding or pregnancy, iron is highly conserved and not readily lost from the body.[ 30 ] There are some obligatory loss of iron from the body that results from the physiologic exfoliation of cells from epithelial surfaces,[ 30 ] including the skin, genitourinary tract, and gastrointestinal tract.[ 3 ] However, these losses are estimated to be very limited (≈1 mg/day).[ 31 ] Iron losses through bleeding can be substantial and excessive menstrual blood loss is the most common cause of iron deficiency in women.
BIOAVAILABILITY
Dietary iron occurs in two forms: heme and nonheme.[ 23 ] The primary sources of heme iron are hemoglobin and myoglobin from consumption of meat, poultry, and fish, whereas nonheme iron is obtained from cereals, pulses, legumes, fruits, and vegetables.[ 32 ] Heme iron is highly bioavailable (15%-35%) and dietary factors have little effect on its absorption, whereas nonheme iron absorption is much lower (2%-20%) and strongly influenced by the presence of other food components.[ 23 ] On the contrary, the quantity of nonheme iron in the diet is manyfold greater than that of heme-iron in most meals. Thus despite its lower bioavailability, nonheme iron generally contributes more to iron nutrition than heme-iron.[ 33 ] Major inhibitors of iron absorption are phytic acid, polyphenols, calcium, and peptides from partially digested proteins.[ 23 ] Enhancers are ascorbic acid and muscle tissue which may reduce ferric iron to ferrous iron and bind it in soluble complexes which are available for absorption[ 23 ]
Factors enhancing iron absorption
A number of dietary factors influence iron absorption. Ascorbate and citrate increase iron uptake in part by acting as weak chelators to help to solubilize the metal in the duodenum [ Table 1 ].[ 34 ] Iron is readily transferred from these compounds into the mucosal lining cells. The dose-dependent enhancing effect of native or added ascorbic acid on iron absorption has been shown by researchers.[ 34 ] The enhancing effect is largely due to its ability to reduce ferric to ferrous iron but is also due to its potential to chelate iron.[ 35 ] Ascorbic acid will overcome the negative effect on iron absorption of all inhibitors, which include phytate,[ 36 ] polyphenols,[ 37 ] and the calcium and proteins in milk products,[ 38 ] and will increase the absorption of both native and fortification iron. In fruit and vegetables, the enhancing effect of ascorbic acid is often cancelled out by the inhibiting effect of polyphenols.[ 39 ] Ascorbic acid is the only absorption enhancer in vegetarian diets, and iron absorption from vegetarian and vegan meals can be best optimized by the inclusion of ascorbic acid-containing vegetables.[ 40 ] Cooking, industrial processing, and storage degrade ascorbic acid and remove its enhancing effect on iron absorption.[ 41 ]
Factors that could influence iron absorption

The enhancing effect of meat, fish, or poultry on iron absorption from vegetarian meals has been shown,[ 42 ] and 30 g muscle tissue is considered equivalent to 25 mg ascorbic acid.[ 33 ] Bjorn-Rasmussen and Hallberg[ 43 ] reported that the addition of chicken, beef, or fish to a maize meal increased nonheme iron absorption 2-3-fold with no influence of the same quantity of protein added as egg albumin. As with ascorbic acid, it has been somewhat more difficult to demonstrate the enhancing effect of meat in multiple meals and complete diet studies. Reddy et al .,[ 44 ] reported only a marginal improvement in iron absorption (35%) in self-selected diets over 5 days when daily muscle tissue intake was increased to 300 g/day, although, in a similar 5-day study, 60 g pork meat added to a vegetarian diet increased iron absorption by 50%.[ 45 ]
Factors inhibiting iron absorption
In plant-based diets, phytate (myo-inositol hexakisphosphate) is the main inhibitor of iron absorption.[ 23 ] The negative effect of phytate on iron absorption has been shown to be dose dependent and starts at very low concentrations of 2-10 mg/meal.[ 37 , 46 ] The molar ratio of phytate to iron can be used to estimate the effect on absorption. The ratio should be 1:1 or preferably, 0.4:1 to significantly improve iron absorption in plain cereal or legume-based meals that do not contain any enhancers of iron absorption, or, 6:1 in composite meals with certain vegetables that contain ascorbic acid and meat as enhancers.[ 47 ]
Polyphenols occur in various amounts in plant foods and beverages, such as vegetables, fruit, some cereals and legumes, tea, coffee, and wine. The inhibiting effect of polyphenols on iron absorption has been shown with black tea and to a lesser extent with herbal teas.[ 48 , 49 ] In cereals and legumes, polyphenols add to the inhibitory effect of phytate, as was shown in a study that compared high and low polyphenol sorghum.[ 23 ]
Calcium has been shown to have negative effects on nonheme and heme iron absorption, which makes it different from other inhibitors that affect nonheme iron absorption only.[ 50 ] Dose-dependent inhibitory effects were shown at doses of 75-300 mg when calcium was added to bread rolls and at doses of 165 mg calcium from milk products.[ 51 ] It is proposed that single-meal studies show negative effects of calcium on iron absorption, whereas multiple-meal studies, with a wide variety of foods and various concentrations of other inhibitors and enhancers, indicate that calcium has only a limited effect on iron absorption.[ 52 ]
Animal proteins such as milk proteins, egg proteins, and albumin, have been shown to inhibit iron absorption.[ 53 ] The two major bovine milk protein fractions, casein and whey, and egg white were shown to inhibit iron absorption in humans.[ 54 ] Proteins from soybean also decrease iron absorption.[ 55 ]
Competition with iron
Competition studies suggest that several other heavy metals may share the iron intestinal absorption pathway. These include lead, manganese, cobalt, and zinc Table 1 . As iron deficiency often coexists with lead intoxication, this interaction can produce particularly serious medical complications in children.[ 56 ]
Lead is a particularly pernicious element to iron metabolism.[ 57 ] Lead is taken up by the iron absorption machinery (DTM1), and secondarily blocks iron through competitive inhibition. Further, lead interferes with a number of important iron-dependent metabolic steps such as heme biosynthesis. This multifaceted influence has particularly dire consequences in children, were lead not only produces anemia, but can impair cognitive development. Lead exists naturally at high levels in ground water and soil in some regions, and can clandestinely attack children's health. For this reason, most pediatricians in the U.S. routinely test for lead at an early age through a simple blood test.
HUMAN REQUIREMENTS
During early infancy, iron requirements are met by the little iron contained in the human milk.[ 58 ] The need for iron rises markedly 4-6 months after birth and amounts to about 0.7-0.9 mg/day during the remaining part of the first year.[ 58 ] Between 1 and 6 years of age, the body iron content is again doubled.[ 58 ] Iron requirements are also very high in adolescents, particularly during the period of growth spurt. Girls usually have their growth spurt before menarche, but growth is not finished at that time. In boys there is a marked increase in hemoglobin mass and concentration during puberty. In this stage, iron requirements increase to a level above the average iron requirements in menstruating women[ 58 ] [see Table 2 ].
Iron requirements of 97.5% of individuals in terms of absorbed iron a , by age group and sex (World Health Organization, 1989)

The average adult stores about 1-3 g of iron in his or her body. A fine balance between dietary uptake and loss maintains this balance. About 1 mg of iron is lost each day through sloughing of cells from skin and mucosal surfaces, including the lining of the gastrointestinal tract.[ 59 ] Menstruation increases the average daily iron loss to about 2 mg per day in premenopausal female adults.[ 60 ] The augmentation of body mass during neonatal and childhood growth spurts transiently boosts iron requirements.[ 61 ]
A dietary intake of iron is needed to replace iron lost in the stools and urine as well as through the skin. These basal losses represent approximately 0.9 mg of iron for an adult male and 0.8 mg for an adult female.[ 62 ] The iron lost in menstrual blood must be taken into consideration for women of reproductive age [ Table 2 ].
GROUPS AT HIGH RISK
The highest probability of suffering iron deficiency is found in those parts of a population that have inadequate access to foods rich in absorbable iron during stages of high iron demand. These groups correspond to children, adolescents, and women of reproductive age, in particular during pregnancy.[ 63 , 58 ]
In the case of infants and adolescents, the increased iron demand is the result of rapid growth. For women of reproductive age the principle reason is the excessive blood loss during menstruation. During pregnancy, there is a significant increase in iron requirement due to the rapid growth of the placenta and the fetus and the expansion of the globular mass.[ 63 ] In contrast, adult men and postmenopausal women are at low risk of iron deficiency and the amount of iron in a normal diet is usually sufficient to cover their physiological requirements.[ 63 ]
CONSEQUENCES AND CAUSES OF IRON DEFICIENCY
Consequences of iron deficiency.
Iron deficiency is defined as a condition in which there are no mobilizable iron stores and in which signs of a compromised supply of iron to tissues, including the erythron, are noted.[ 64 ] Iron deficiency can exist with or without anemia. Some functional changes may occur in the absence of anemia, but the most functional deficits appear to occur with the development of anemia.[ 2 ] Even mild and moderate forms of iron deficiency anemia can be associated with functional impairments affecting cognitive development,[ 65 ] immunity mechanisms,[ 66 ] and work capacity.[ 67 ] Iron deficiency during pregnancy is associated with a variety of adverse outcomes for both mother and infant, including increased risk of sepsis, maternal mortality, perinatal mortality, and low birth weight.[ 68 ] Iron deficiency and anemia also reduce learning ability and are associated with increased rates of morbidity.[ 68 ]
Causes of iron deficiency
Iron deficiency results from depletion of iron stores and occurs when iron absorption cannot keep pace over an extended period with the metabolic demands for iron to sustain growth and to replenish iron loss, which is primarily related to blood loss.[ 2 ] The primary causes of iron deficiency include low intake of bioavailable iron, increased iron requirements as a result of rapid growth, pregnancy, menstruation, and excess blood loss caused by pathologic infections, such as hook worm and whipworm causing gastrointestinal blood loss[ 69 , 70 , 71 , 72 ] and impaired absorption of iron.[ 73 ] The frequency of iron deficiency rises in female adolescents because menstrual iron losses are superimposed with needs for rapid growth.[ 74 ] Other risk factors for iron deficiency in young women are high parity, use of an intrauterine device, and vegetarian diets.[ 75 ]
Nutritional iron deficiency arises when physiological requirements cannot be met by iron absorption from the diet.[ 72 ] Dietary iron bioavailability is low in populations consuming monotonous plant-based diets with little meat.[ 72 ] In many developing countries, plant-based weaning-foods are rarely fortified with iron, and the frequency of anemia exceeds 50% in children younger than 4 years.[ 64 ]
When iron stores are depleted and insufficient iron is available for erythropoiesis, hemoglobin synthesis in erythrocyte precursors become impaired and hematologic signs of iron deficiency anemia appear.
EVALUATION OF IRON STATUS
Iron deficiency and eventually anemia develop in stages and can be assessed by measuring various biochemical indices. Although some iron enzymes are sensitive to iron deficiency,[ 63 ] their activity has not been used as a successful routine measure of iron status.[ 2 ]
Laboratory measurements are essential for a proper diagnosis of iron deficiency. They are most informative when multiple measures of iron status are examined and evaluated in the context of nutritional and medical history.
The plasma or serum pool of iron is the fraction of all iron in the body that circulates bound primarily to transferrin. Three ways of estimating the level of iron in the plasma or serum include 1) measuring the total iron content per unit volume in μg/dL; 2) measuring the total number of binding sites for iron atoms on transferrin, known as total iron-binding capacity in μg/dL 2 ; and 3) estimating the percentage of the two bindings sites on all transferrin molecules that are occupied called the percentage transferrin saturation.[ 76 ] However, marked biologic variation can occur in these values as a result of diurnal variation, the presence of infection or inflammatory conditions and recent dietary iron intake.[ 76 ]
Zinc protoporphyrin reflects the shortage of iron supply in the last stages of hemoglobin synthesis so that zinc is inserted into the protoporphyrin molecule in the place of iron. Zinc protoporphyrin can be detected in RBCs by fluorimetry and is a measure of the severity of iron deficiency.[ 76 ]
Serum ferritin is a good indicator of body iron stores under most circumstances. When the concentration of serum ferritin is ≥15 μg/L iron stores are present; higher concentrations reflect the size of the iron store; when the concentration is low (<12 μg/L for <5 years of age and <15 μg/L for >5 years of age) iron stores are depleted.[ 76 ] However, ferritin is an acute phase reactant protein and its serum concentrations can be elevated, irrespective of a change in iron stores, by infection or inflammation.[ 76 , 2 ] This means that it might be difficult to interpret the concentration of ferritin where infectious diseases are common.
Another indicator of iron status is the concentration of TfR in serum. Since TfR is mostly derived from developing RBCs, it reflects the intensity of erythropoiesis and the demand for iron. As iron stores are exhausted, the concentration rises in iron deficiency anemia indicating sever iron insufficiency. This is provided that there are no other causes of abnormal erythropoiesis.[ 76 ] Clinical studies indicate that the serum TfR is less affected by inflammation than serum ferritin.[ 77 ] The major advantage of TfR as an indicator is the possibility of estimating the magnitude of the functional iron deficit once iron stores are depleted.[ 78 ]
The ratio of TfR to ferritin (TfR/ferritin) was designed to evaluate changes in both stored iron and functional iron and was thought to be more useful than either TfR or ferritin alone.[ 79 ] TfR/ferritin has been used to estimate body iron stores in both children and adults.[ 80 ] However, the high cost and the lack of standardization of the TfR assay so far have limited the applicability of the method.[ 81 ]
Low hemoglobin concentration is a measure of anemia, the end stage of iron deficiency.[ 76 , 2 ]
ANEMIA AND ITS CAUSES
Anemia describes the condition in which the number of RBCs in the blood is low, or the blood cells have less than the normal amount of hemoglobin. A person who has anemia is called anemic. The purpose of the RBC is to deliver oxygen from the lungs to other parts of the body. The hemoglobin molecule is the functional unit of the RBCs and is a complex protein structure that is inside the RBCs. Even though the RBCs are made within the bone marrow, many other factors are involved in their production. For example, iron is a very important component of the hemoglobin molecule; erythropoietin, a molecule secreted by the kidneys, promotes the formation of RBCs in the bone marrow.
Having the correct number of RBCs and prevention of anemia requires cooperation among the kidneys, the bone marrow, and nutrients within the body. If the kidneys or bone marrow are not functioning, or the body is poorly nourished, then normal RBC count and functions may be difficult to maintain.
Anemia is actually a sign of a disease process rather than a disease itself. It is usually classified as either chronic or acute. Chronic anemia occurs over a long period of time. Acute anemia occurs quickly. Determining whether anemia has been present for a long time or whether it is something new, assists doctors in finding the cause. This also helps predict how severe the symptoms of anemia may be. In chronic anemia, symptoms typically begin slowly and progress gradually; whereas in acute anemia symptoms can be abrupt and more distressing.
RBCs live about 100 days, so the body is constantly trying to replace them. In adults, RBC production occurs in the bone marrow. Doctors try to determine if a low RBC count is caused by increased blood loss of RBCs or from decreased production of them in the bone marrow. Knowing whether the number of white blood cells and/or platelets has changed also helps determine the cause of anemia.
World Health Organization (WHO) estimates that two billion people are anemic worldwide and attribute approximately 50% of all anemia to iron deficiency.[ 64 ] It occurs at all stages of the life cycle but is more prevalent in pregnant women and young children.[ 82 ] Anemia is the result of a wide variety of causes that can be isolated, but more often coexist. Some of these causes include the following:
Iron deficiency anemia
The most significant and common cause of anemia is iron deficiency.[ 82 ] If iron intake is limited or inadequate due to poor dietary intake, anemia may occur as a result. This is called iron deficiency anemia. Iron deficiency anemia can also occur when there are stomach ulcers or other sources of slow, chronic bleeding (colon cancer, uterine cancer, intestinal polyps, hemorrhoids, etc).[ 83 ]
Anemia of chronic disease
Any long-term medical condition can lead to anemia. This type of anemia is the second most prevalent after anemia caused by iron deficiency and develops in patients with acute or chronic systemic illness or inflammation.[ 84 ] The condition has thus been termed “anemia of inflammation” due to elevated hepcidin which blocks both the recycling of iron from the macrophages and iron absorption.[ 85 ]
Anemia from active bleeding
Loss of blood through heavy menstrual bleeding or wounds can cause anemia.[ 82 ] Gastrointestinal ulcers or cancers such as cancer of the colon may slowly lose blood and can also cause anemia.[ 86 , 87 ]
Anemia related to kidney disease
The kidneys releases a hormone called the erythropoietin that helps the bone marrow make RBCs. In people with chronic (long-standing) kidney disease, the production of this hormone is diminished, and this in turn diminishes the production of RBCs, causing anemia.[ 88 ] Although deficiency of erythropoietin is the primary cause of anemia in chronic renal failure, it is not the only cause. Therefore, a minimal workup is necessary to rule out iron deficiency and other cell-line abnormalities.[ 89 ]
Anemia related to pregnancy
A gain in plasma volume during pregnancy dilutes the RBCs and may be reflected as anemia.[ 90 ] Iron deficiency anemia accounts for 75% of all anemia in pregnancy.[ 90 ]
Anemia related to poor nutrition
Vitamins and minerals are required to make RBCs. In addition to iron, vitamin B12, viamin A, folate, riboflavin, and copper are required for the proper production of hemoglobin.[ 82 ] Deficiency in any of these micronutrients may cause anemia because of inadequate production of RBCs. Poor dietary intake is an important cause of low vitamin levels and therefore anemia.
Obesity and anemia
Obesity is characterized by chronic, low-grade, systemic inflammation, elevated hepcidin, which, in turn has been associated with anemia of chronic disease. Ausk and Ioannou[ 91 ] hypothesized that obesity may be associated with the features of anemia of chronic disease, including low hemoglobin concentration, low serum iron and transferrin saturation, and elevated serum ferritin. Overweight and obesity were associated with changes in serum iron, transferrin saturation, and ferritin that would be expected to occur in the setting of chronic, systemic inflammation. Obesity-related inflammation may increase hepcidin concentrations and reduce iron availability. Aeberli et al .,[ 92 ] compared iron status, dietary iron intake and bioavailability, as well as circulating levels of hepcidin, leptin, and interleukin-6 (IL-6), in overweight versus normal weight children. They indicated that there is reduced iron availability for erythropoiesis in overweight children and that this is likely due to hepcidin-mediated reduced iron absorption and/or increased iron sequestration rather than low dietary iron supply.
Alcohol has numerous adverse effects on the various types of blood cells and their functions.[ 93 ] Alcoholics frequently have defective RBCs that are destroyed prematurely.[ 93 , 94 ] Alcohol itself may also be toxic to the bone marrow and may slow down the RBC production.[ 93 , 94 ] In addition, poor nutrition and deficiencies of vitamins and minerals are associated with alcoholism.[ 95 ] The combination of these factors may lead to anemia in alcoholics.
Sickle cell anemia
Sickle cell anemia is one of the most common inherited diseases.[ 96 ] It is a blood-related disorder that affects the hemoglobin molecule and causes the entire blood cell to change shape under stressed conditions.[ 97 ] In this condition, the hemoglobin problem is qualitative or functional. Abnormal hemoglobin molecules may cause problems in the integrity of the RBC structure and they may become crescent-shaped (sickle cells).[ 97 ] There are different types of sickle cell anemia with different severity levels. It is particularly common in African, Middle Eastern, and Mediterranean ancestry.[ 97 ]
Thalassemia
This is another group of hemoglobin-related causes of anemia, which involves the absence of or errors in genes responsible for production of hemoglobin.[ 97 ] A hemoglobin molecule has subunits commonly referred to as alpha and beta globin chains. A lack of a particular subunit determines the type of alpha or beta thalassemia.[ 97 , 98 ] There are many types of thalassemia, which vary in severity from mild (thalassemia minor) to severe (thalassemia major).[ 98 ] These are also hereditary, but they cause quantitative hemoglobin abnormalities, meaning an insufficient amount of the correct hemoglobin type molecules is made. The alpha and beta thalassemias are the most common-inherited single-gene disorders in the world with the highest prevalence in areas where malaria was or still is endemic.[ 97 ]
Aplastic anemia
Aplastic anemia is a disease in which the bone marrow is destructed and the production of blood cells is diminished.[ 99 ] This causes a deficiency of all three types of blood cells (pancytopenia) including RBCs (anemia), white blood cells (leukopenia), and platelets (thrombocytopenia).[ 100 , 101 ] Many common medications can occasionally cause this type of anemia as a side effect in some individuals.[ 99 ]
Hemolytic anemia
Hemolytic anemia is a type of anemia in which the RBCs rupture, known as hemolysis, and are destroyed faster than the bone marrow can replace them.[ 102 ] Hemolytic anemia could happen due to a variety of reasons and is often categorized as acquired or hereditary. Common acquired causes of hemolytic anemia are autoimmunity, microangiopathy, and infection. Disorders of RBC enzymes, membranes, and hemoglobin cause hereditary hemolytic anemia.[ 102 ]
PREVENTION OF IRON DEFICIENCY (INTERVENTION STRATEGIES)
The four principle strategies for correcting micronutrient efficiencies in populations can be used for correcting iron deficiency, either alone or in combination. These are education combined with dietary modification, to improve iron intake and bioavailability; iron supplementation (provision of iron, usually in higher doses, without food), iron fortification of foods and the new approach of biofortification. However, there are some difficulties in the application of some of these strategies when considering iron.
Food diversification
Dietary modifications for reducing Indian Dental Association involve increased intake of iron rich foods, especially flesh foods, increased consumption of fruits and vegetables rich in ascorbic acid to enhance nonheme iron absorption, and reduced intake of tea and coffee, which inhibit nonheme iron absorption.[ 103 , 58 ] Another strategy is to reduce antinutrient contents in order to make the iron supplied from their food sources more available. Iron bioavailability may be increased by techniques such as germination and fermentation, which promote enzymatic hydrolysis of phytic acid in whole grain cereals and legumes by enhancing the activity of endogenous or exogenous phytase enzymes.[ 104 ] Even the use of nonenzymatic methods, such as thermal processing, soaking, and milling, for reducing phytic acid content in plant-based staples has been successful in improving the bioavailability of iron (and zinc).[ 105 , 106 ]
Supplementation
For oral iron supplementation, ferrous iron salts (ferrous sulfate and ferrous gluconate) are preferred because of their low cost and high bioavailability.[ 72 ] Although iron absorption is higher when iron supplements are given on an empty stomach, nausea, and epigastric pain might develop due to the higher iron doses administered (usually 60 mg Fe/day). If such side-effects arise, lower doses between meals should be attempted or iron should be provided with meals, although food reduces absorption of medicinal iron by about two-thirds.[ 107 ] Iron supplementation during pregnancy is advisable in developing countries, where women often enter pregnancy with low iron stores.[ 108 ] Although the benefits of iron supplementation have generally been considered to outweigh the putative risks, there is some evidence to suggest that supplementation at levels recommended for otherwise healthy children carries the risk of increased severity of infectious disease in the presence of malaria.[ 109 , 110 ]
Fortification
Fortification of foods with iron is more difficult than fortification with nutrients, such as zinc in flour, iodine in salt, and vitamin A in cooking oil.[ 72 ] The most bioavailable iron compounds are soluble in water or diluted acid but often react with other food components to cause off-flavors, color changes or fat oxidation.[ 103 ] Thus, less soluble forms of iron, although less well absorbed, are often chosen for fortification to avoid unwanted sensory changes.[ 72 ] Fortification is usually made with much lower iron doses than supplementation. It is closer to the physiological environment and might be the safest intervention in malarious areas.[ 111 ] There is no concern over the safety of iron supplementation or iron fortification in nonmalarial endemic areas.[ 112 ]
Iron compounds recommended for food fortification by the[ 7 ] include ferrous sulfate, ferrous fumarate, ferric pyrophosphate, and electrolytic iron powder. Wheat flour is the most common iron fortified food and it is usually fortified with elemental iron powders which are not recommended by WHO.[ 7 , 113 ] Hurrell and Egli[ 23 ] reported that of the 78 national wheat flour programs only eight would be expected to improve iron status. These programs used recommended iron compounds at the recommended levels. The other countries used non recommended compounds or lower levels of iron relative to flour intake. Commercial infant foods, such as formulas and cereals, are also commonly fortified with iron.
Biofortification
Iron contents vary from 25 to 56 mg/kg in the different varieties of wheat and 7-23 mg/kg in rice grains. However, most of this iron is removed during the milling process. Iron absorption from cereals and legumes, many of which have high native iron content, is generally low because of their high contents of phytate and sometimes polyphenols.[ 48 ] Biofortification strategies include plant breeding and genetic engineering. Iron levels in common beans and millet have been successfully increased by plant breeding but other staple is more difficult or not possible (rice) due to insufficient natural genetic variation. Lucca et al .,[ 114 ] increased the iron content in rice endosperm to improve its absorption in the human intestine by means of genetic engineering. They introduced a ferritin gene from Phaseolus vulgaris into rice grains, increasing their iron content up to twofold. To increase iron bioavailability, they introduced a thermotolerant phytase from Aspergillus fumigatus into the rice endosperm. They indicated that this rice, with higher iron content and rich in phytase has a great potential to substantially improve iron nutrition in those populations where iron deficiency is so widely spread.[ 114 ] Unfortunately the phytase did not resist cooking. The importance of various minerals as zinc[ 115 ] and iron needs more attention at individual and public health levels.
Source of Support: Nil
Conflict of Interest: None declared.

IMAGES
VIDEO
COMMENTS
The human body is a complex organism, the gross mechanical properties of which are enabled by an interconnected musculoskeletal network controlled by the nervous system. ... All relevant data are within the paper and its Supporting Information files. The two musculoskeletal graphs used, as well as muscle community assignments, and data used to ...
Stress and Memory. Memory is one of the important functional aspects of the CNS and it is categorized as sensory, short term, and long-term. Short term memory is dependent on the function of the frontal and parietal lobes, while long-term memory depends on the function of large areas of the brain (Wood et al., 2000[]).However, total function of memory and the conversion of short term memory to ...
Abstract. This paper proposed the scientific hypothesis that human beings have an "energy system" inside their body and put forward the necessity to propose the hypothesis. In details, this paper explained the hypothesis from its existence, composition, functions, and possible verifying methods. This paper argues that the human body ...
The Human Reference Atlas (HRA) aims to map all of the cells of the human body to advance biomedical research and clinical practice. This Perspective presents collaborative work by members of 16 ...
Human tissues used in research may involve a variety of cell types and preparations and they may be collected in a number of different ways. In addition to solid tissues, human biospecimens that are often collected and used for research include whole blood and blood components, such as serum, plasma, and buffy coat.
The human body constantly exchanges heat with the environment. Temperature regulation is a homeostatic feedback control system that ensures deep body temperature is maintained within narrow limits despite wide variations in environmental conditions and activity-related elevations in metabolic heat production. Extensive research has been performed to study the physiological regulation of deep ...
Metrics. The human body supports a thriving diversity of microbes which comprise a dynamic, ancillary, functional system that synergistically develops in lock-step with physiological development ...
Protein Science, the flagship journal of The Protein Society, serves an international forum for publishing original reports on all scientific aspects of protein molecules. The Journal publishes papers by leading scientists from all over the world that report on advances in the understanding of proteins in the broadest sense. Protein Science aims to unify this field by cutting across ...
Read the paper: A molecular and cellular perspective on human brain evolution and tempo The 1964 Declaration of Helsinki is the basis of research ethics for studies involving humans.
Feature papers represent the most advanced research with significant potential for high impact in the field. A Feature Paper should be a substantial original Article that involves several techniques or approaches, provides an outlook for future research directions and describes possible research applications. ... Human body structures from a ...
The human body contains many hidden and enigmatic features that humankind has not yet fully understood. A total of 7539 neoplasm cases were reported from 1 January 2021 to 31 December 2021. Of ...
Body Image is an international, peer-reviewed journal that publishes high-quality, scientific articles on body image and human physical appearance. Body image represents a person's "inside view" of their body-that is, their feelings, perceptions, thoughts, and beliefs about their body that impact …. View full aims & scope.
Although extensive research has been carried out on the numerical simulation of human-structure interaction systems using a single-degree-of-freedom human body model [47,48,49], less research has been carried out to obtain human body parameters from this coupled model via a full-scale structural model. Furthermore, the methods commonly used in ...
increase or lose of hepatitis, muscle tension in neck face or shoulders, problems sleeping, raising. heart, cold, sweaty palms, tiredness, trembling or shacking or weight gain or lose, upset ...
A total of 70 yearling rams of Akkaraman breed, 50 of which were classified as growth-failure with an average body weight of 37.30 ± 1.1 kg (test) and the remaining 20 were classified as normal ...
The body of literature that has developed since then focuses exclusively on experimentation with human subjects 'as a whole', that is, persons and their (entire) bodies [15,19]. Much less attention has been given to biomedical research (medical experimentation) with human body parts. For example, the Declaration of Helsinki contains no ...
Human adults were administered 25 mg of zinc (as Zinc sulfate [ZnSO4]) in water solution, and iron was added at 25, 50 or 75 mg. Plasma zinc was reduced significantly with increasing dose of iron. Lonnerdal used a dose of zinc similar to that obtained from most meals and studied zinc absorption by using radiolabeled zinc and whole-body counting.
The aim of this paper is to discuss the problem of the human body as to whether it is wholly and directly influenced by the rational forces of the soul, or it contains something instinctive or unconscious that can exert a determinative... more. Download. by Studia Gilsoniana. 7.
Introduction. The interconnected nature of the human body has long been the subject of both scientific inquiry and superstitious beliefs. From the ancient humors linking heart, liver, spleen, and brain with courage, calm, and hope [] to the modern appreciation of the gut-brain connection [], humans tend to search for interconnections between disparate parts of the body to explain complex ...
Human Body Systems Project By Eva McLanahan. Students will work in groups to research one of the eleven body systems as found in Holt, Rinehart, and Winston Modern Biology (2002). Research will focus on the structure and function of the major organs in the assigned body system. Each group will be responsible for a visual aid to be used in a ...
Human Tissue for Research Diagram. A diagram detailing the tissues and organs that are available through IIAM for research. Download File 3521_IIAM_Tissues-Available-Diagram_2017.pdf - 163.05 KB. 800-486-IIAM (4426) Virtually every organ, tissue and system of the human body is needed for medical research, and nearly every disease that plagues ...
The present paper describes the effects on human body by music and vibroacoustic stimuli. The experiments were carried out six times for 3 subjects and have investigated the electroencephalogram ...
Introduction. During the last century, research has been increasingly drawn toward understanding the human-nature relationship (1, 2) and has revealed the many ways humans are linked with the natural environment ().Some examples of these include humans' preference for scenes dominated by natural elements (), the sustainability of natural resources (5, 6), and the health benefits associated ...
This essay is about the concept of "body farms" and their crucial role in forensic science and anthropology. It explains how these research facilities, first established by Dr. William Bass in 1981, help study human decomposition under various conditions to improve the accuracy of post-mortem interval (PMI) estimations.
Recent Graduate's Paper Published in PNAS June 6, 2024 June 12, 2024 Hongru Zhu (PhD '23) published a paper with Prof. Daniel Kersten (UMN) on Natural scenes reveal diverse representations of 2D and 3D body pose in the human brain in Proceedings of the National Academy of Sciences based on research he started as a student in our program.
HUMAN REQUIREMENTS. During early infancy, iron requirements are met by the little iron contained in the human milk. The need for iron rises markedly 4-6 months after birth and amounts to about 0.7-0.9 mg/day during the remaining part of the first year. Between 1 and 6 years of age, the body iron content is again doubled. Iron requirements are ...