Thank you for visiting nature.com. You are using a browser version with limited support for CSS. To obtain the best experience, we recommend you use a more up to date browser (or turn off compatibility mode in Internet Explorer). In the meantime, to ensure continued support, we are displaying the site without styles and JavaScript.
- View all journals
- My Account Login
- Explore content
- About the journal
- Publish with us
- Sign up for alerts
- Open access
- Published: 09 June 2022

Underwater wireless communication via TENG-generated Maxwell’s displacement current
- Hongfa Zhao ORCID: orcid.org/0000-0003-1929-3184 1 , 2 na1 ,
- Minyi Xu ORCID: orcid.org/0000-0002-3772-8340 1 na1 ,
- Mingrui Shu ORCID: orcid.org/0000-0001-9139-5092 1 na1 ,
- Jie An ORCID: orcid.org/0000-0002-0028-0079 3 ,
- Wenbo Ding ORCID: orcid.org/0000-0002-0597-4512 2 ,
- Xiangyu Liu ORCID: orcid.org/0000-0003-0386-9089 1 ,
- Siyuan Wang ORCID: orcid.org/0000-0001-7174-9754 1 ,
- Cong Zhao ORCID: orcid.org/0000-0003-3647-7567 1 ,
- Hongyong Yu ORCID: orcid.org/0000-0002-7195-7139 1 ,
- Hao Wang ORCID: orcid.org/0000-0002-9238-9791 1 ,
- Chuan Wang 1 ,
- Xianping Fu ORCID: orcid.org/0000-0001-9888-9327 1 ,
- Xinxiang Pan ORCID: orcid.org/0000-0003-0460-0620 1 ,
- Guangming Xie ORCID: orcid.org/0000-0001-6504-0087 1 , 4 , 5 &
- Zhong Lin Wang ORCID: orcid.org/0000-0002-5530-0380 3 , 6
Nature Communications volume 13 , Article number: 3325 ( 2022 ) Cite this article
17k Accesses
74 Citations
6 Altmetric
Metrics details
- Applied physics
- Electrical and electronic engineering
- Sensors and biosensors
Underwater communication is a critical and challenging issue, on account of the complex underwater environment. This study introduces an underwater wireless communication approach via Maxwell’s displacement current generated by a triboelectric nanogenerator. Underwater electric field can be generated through a wire connected to a triboelectric nanogenerator, while current signal can be inducted in an underwater receiver certain distance away. The received current signals are basically immune to disturbances from salinity, turbidity and submerged obstacles. Even after passing through a 100 m long spiral water pipe, the electric signals are not distorted in waveform. By modulating and demodulating the current signals generated by a sound driven triboelectric nanogenerator, texts and images can be transmitted in a water tank at 16 bits/s. An underwater lighting system is operated by the triboelectric nanogenerator-based voice-activated controller wirelessly. This triboelectric nanogenerator-based approach can form the basis for an alternative wireless communication in complex underwater environments.
Similar content being viewed by others
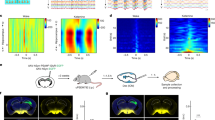
Neuronal dynamics direct cerebrospinal fluid perfusion and brain clearance
Li-Feng Jiang-Xie, Antoine Drieu, … Jonathan Kipnis
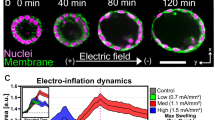
Bioelectric stimulation controls tissue shape and size
Gawoon Shim, Isaac B. Breinyn, … Daniel J. Cohen
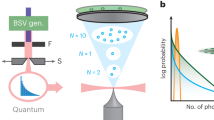
Multiphoton electron emission with non-classical light
Jonas Heimerl, Alexander Mikhaylov, … Peter Hommelhoff
Introduction
In the booming ocean exploration, underwater equipment and technology is attracting more and more attention 1 , 2 , 3 , 4 , 5 . Particularly, obtaining underwater wireless communication has always been a critical challenge. The current underwater communication is achieved through different physical fields, such as acoustic field, optical field, and electromagnetic field 6 , 7 , 8 .
Acoustic communication is most widely used underwater communication as sound wave is not absorbed by water so easily like electromagnetic wave and optical wave. However, acoustic communication has always been accompanied by considerable transmission delays while the transmission is subject to influences from temperature, pressure, and salinity, which leads to multipath effects and Doppler frequency shift. What’s more, echo and reverberation from obstacles could make acoustic communications inaccessible in certain environments (such as confined space, narrow pipes, tunnels, and caves) 9 , 10 . Underwater optical communication can realize large-capacity data transmission, but it is subject to absorption, scattering, beam divergence, and ambient light interruptions 11 , 12 . Compared to the acoustic and optical waves, the electromagnetic waves are not affected by acoustic noise or turbulence. Underwater displacement current communication usually has high transmission rate and low delay 10 . While high-frequency electromagnetic waves will be largely absorbed by water 13 , 14 , low-frequency electromagnetic waves can transmit through an antenna of several kilometers. In sum, complex and sometimes confined underwater space turns out to be a considerable challenge to traditional underwater communication technologies.
Under those conditions, an alternative communication that can work well in underwater space is definitely needed. An inspiration comes to our mind from the Maxwell’s equations, foundation of modern wireless electromagnetic communication. The displacement current, corresponding to ∂ D /∂ t in the Maxwell’s equations, is what unified electricity and magnetism theoratically 15 . Of the two terms in displacement current, the first term ∂ E /∂ t induces electromagnetic waves widely used in information technology, especially in wireless communications. The second term ∂ P /∂ t in the displacement current is induced by the polarization of media 16 , 17 . Previous studies by Prof. Z. L. Wang reveal that the second term ∂ P /∂ t in the Maxwell’s displacement current can be directly related to the output electric current of the nanogenerator 18 , 19 , 20 , 21 , 22 , 23 , 24 , 25 . A few studies have been performed on the energy transmission or communications (in air), based on the Maxwell’s displacement current generated by the triboelectric nanogenerator (TENG). Recently, Zi et al. (2021) used TENGs to generate a rapidly alternating electric field so that wireless communication in air can be realized by the displacement current ∂ P /∂ t 26 . Compared with air, water is of a larger dielectric constant, which is more conducive to the propagation of the polarization electric field. Therefore, based on the second term of the displacement current, i.e., the polarization electric field, underwater communication in complex waters is feasible.
In this study, an underwater wireless communication via Maxwell’s displacement current, ∂ P /∂ t , is proposed. The TENG that converts sound to electricity is connected to a transmitting electrode to generate the time-varying polarization electric field underwater. The corresponding time-varying current is measured with a receiving electrode connected to an electrometer. The study reveals that the current signals generated by the TENG yield good anti-interference ability to underwater disturbances. Through a 100 m long salt water pipe, the peak value of the current signal decreases by 66% from the original signal, while the waveforms of the electric signals are not distorted. With the on-off keying method, texts and images can be successfully transmitted in a water tank. No errors appeared in the continuous transmission for about 20,000 digital signals, and an underwater lighting system has been voice-controlled wirelessly via the TENG. What’s more, the current signals output by a sandwich-like TENG can be transmitted in a 50 m × 30 m × 5 m basin with the signals displayed on screen in real time. Therefore, it is believed that the presented work could become an effective communication approach in underwater environments.
Working principle of the underwater electric field communication
A conceptual diagram of the application of the studied underwater communication is shown in Fig. 1 . The TENG converts sound (i.e. sonic waves in air) to electric signals in water (Fig. 1a ), and the electric signals carrying the voice information can be transmitted in water and received by the diver. In this way, an underwater wireless communication is established via Maxwell’s displacement current generated by the TENG. Figure 1b is the flow chart of the underwater communication. It needs to be noted that this method is different from the electric field communication generated from a pair of electric dipoles (see Supplementary Note 1 ).
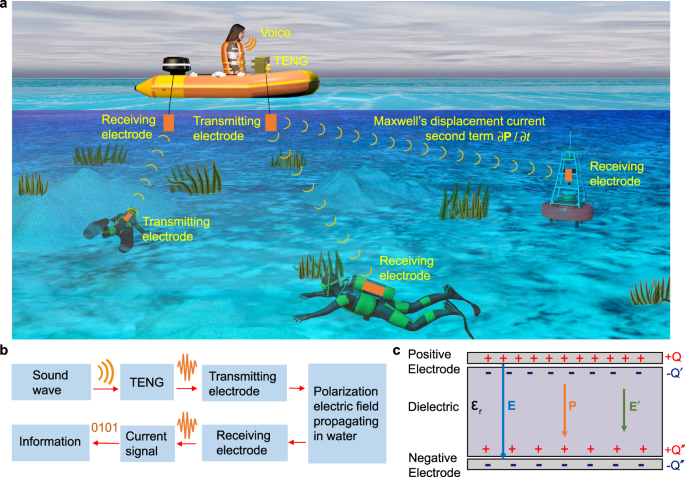
(signals generated by TENG is directly transmitted without amplification by an external power source). a The application and ( b ) the flow chart of the underwater wireless communication. c Schematic diagram of the capacitance model, \({{{\varepsilon }}}_{{{r}}}\) is relative permittivity, E is the original electric field, P is the polarization electric field, E′ is the combined electric field of ( E ) and ( P ), and all about Q are amount of charge.
The working principle of the underwater communication can be understood, approximately, with a capacitance model (Fig. 1c ). The propagation of underwater electric field is analyzed from the perspective of displacement current. The transmitting and receiving electrodes form the positive and negative electrodes of the capacitor, while the water solution is the dielectric. With the presence of electric field E , the dielectric can be polarized where a polarization electric field P can be generated. It should be noted that the polarization electric field P is originated from the negative polarization charge to the positive polarization charge. \({{{{{\bf{E}}}}}}^{\prime}\) is the combined electric field of E and P , If the relative permittivity is defined as \({\varepsilon }_{r}={{{{{\bf{E}}}}}}{{{{{\boldsymbol{/}}}}}}{{{{{\bf{E}}}}}}^{\prime}\) , the relationship between the polarization charge and the charge Q on the transmitting electrode is \({Q}^{\prime} =(1-1/{\varepsilon }_{r})Q\) . Due to the attenuation of the electric field during through propagation medium, the received charge \(Q^{\prime\prime}\) at the receiving electrode is < \(Q^{\prime}\) .
The underwater communication can be demonstrated theoretically with the Maxwell’s equation. Remind that the Gauss’s law of the Maxwell’s equations is
where ρ is the distribution of free charges in space, and D is the electric displacement vector, which can be expressed as
where permittivity in vacuum is \({{{\varepsilon }}}_{{{0}}}\) . The Maxwell’s displacement current density can be defined as
From Eq. ( 3 ), the first term \({{{\varepsilon }}}_{{{0}}}\partial {{{{{\bf{E}}}}}}/\partial {t}\) gives rise to electromagnetic wave. Studies of Prof. Zhonglin Wang reveal that the second term (∂ P /∂t) in the Maxwell’s displacement current can be directly related to the output electric current of the TENG 15 .
It is worth mentioning that the internal circuit in the TENG is dominated by the displacement current, and the observed current in the external circuit is the capacitive conduction current (see Supplementary Note 2 ). The research on the underwater electric field propagation is inspired by the built-in electric field of the TENG. Comparing the TENG-based underwater electric field with electromagnetic waves, the propagation of electromagnetic waves does not require a medium, and the propagation effect is best in vacuum. At this time, ∂ E /∂t reaches the maximum value, and ∂ P /∂t is 0. The propagation of the polarization electric field requires a medium (see Supplementary Note 3 ). As ∂ E /∂t gets significantly reduced in water, the propagation effect of ∂ P /∂t gets improved.
To examine the performance of the underwater communication, an acoustic-driven TENG is applied to convert sound in air to electrical signals in water. The output performance of the acoustic-driven TENG has been investigated systematically in our previous study 27 . As shown in Fig. 2a , the TENG consists of a Helmholtz resonant cavity, an aluminum film with evenly distributed acoustic holes, and a fluorinated ethylene propylene (FEP) film with a conductive ink-printed electrode (details about the TENG is shown in Supplementary Notes 4 and 5 ). The transmitting electrode in water is connected to the aluminum electrode of the TENG, while the other electrode of the TENG is grounded so that the TENG operates in the single-electrode mode. In reaching an electrostatic equilibrium state, higher electrical output can be obtained by acquiring ground charges 28 . It is worth noting that one piece of conduct materials, such as metal and salt water, can serve as a charge reservoir for the TENG.
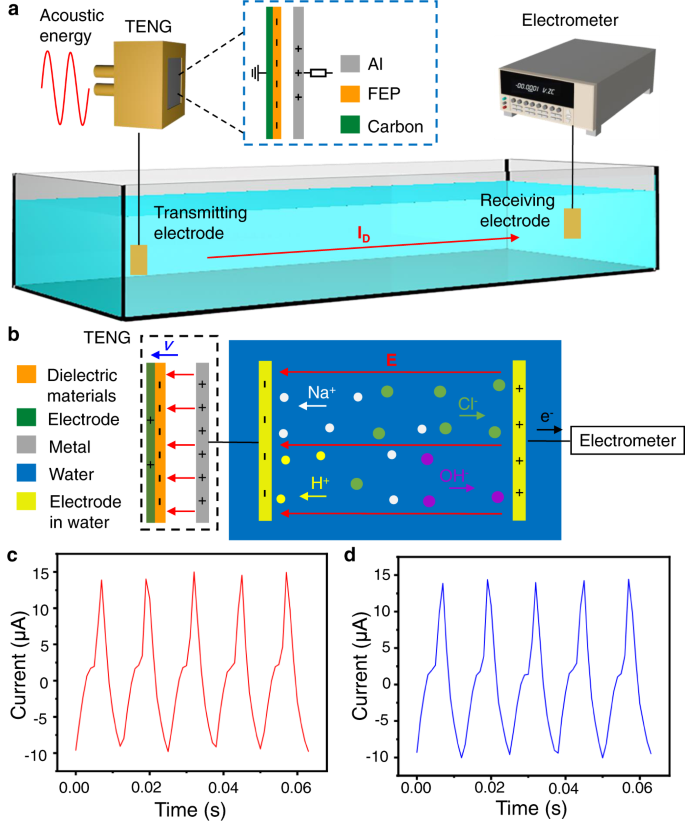
a Schematic diagram of the experimental process. I D represents displacement current in all figures. b Schematic diagram of the working principle. E is the underwater electric field, and v is the speed of the TENG for contact and separation. c The short-circuit current signals (measured by connecting an electrometer to the aluminum electrode). d The short-circuit current signal obtained by connecting the electrometer to the receiving electrode.
Figure 2b shows the working principle of the underwater communication, which is based on the interface polarization from the Maxwell-Wagner effect. The electrical output is generated from the variation of the built-in electric field in the TENG, which is directly related to the second term (∂ P /∂t) in the Maxwell’s displacement current 15 . A transmitting electrode is connected to one electrode of the TENG, thus an electric field \({{{{{{\bf{E}}}}}}}_{{{{{{\bf{0}}}}}}}\) is induced in water as the TENG works (see Fig. 2b ). Corresponding to the variation of electric field \({{{{{{\bf{E}}}}}}}_{{{{{{\bf{0}}}}}}}\) , the positive and negative ions in the water move reciprocally, generating a polarization electric field \({{{{{{\bf{P}}}}}}}_{{{{{{\bf{0}}}}}}}\) . The current in the receiving electrode induced by the polarization electric field can be measured with an electrometer. The electric field \({{{{{{\bf{E}}}}}}}_{{{{{{\bf{0}}}}}}}\) generated by the TENG is related to the charge density \({{{{{{\boldsymbol{\rho }}}}}}}_{{{{{{\boldsymbol{0}}}}}}}\) in the transmitting electrode in the following form:
\({{{{{{\bf{P}}}}}}}_{{{{{{\bf{0}}}}}}}\) is the polarization electric field generated from the electric field \({{{{{{\bf{E}}}}}}}_{{{{{{\bf{0}}}}}}}\) , which is
Therefore, the second term \({{{{{{\bf{J}}}}}}}_{{{{{{\bf{p}}}}}}}\) in the Maxwell’s displacement current (generated by the polarization electric field) is
For the acoustic driven HR-TENG, when the FEP film contacts with the aluminum film, the electron clouds on the surfaces of the two films overlap, and some of the electrons from the aluminum film enter the deeper potential well of the FEP film. Due to the much higher electronegativity of FEP than aluminum, the free electrons on the surface of the aluminum film transfer to the lowest unoccupied molecular orbital of the FEP interface. So the aluminum film becomes positively charged (Supplementary Fig. 1a ). Since the transmitting electrode is connected to the aluminum film, positive charges are also distributed on the surface of the transmitting electrode. Negatively charged ions in the water are attracted by the transmitting electrode, while positively charged ions are repelled to the surroundings. When positive ions contact with the receiving electrode, electrons in the receiving circuit flow to the receiving electrode, so the electrometer detects a positive current. Due to the change in the acoustic pressure difference, the FEP film is separated from the aluminum electrode. At the moment, electrons flow from the ground to the conductive ink electrode to balance the electric field between the FEP film and the conductive ink electrode. Due to the negative charge distributed on the surface of the FEP film, the free electrons on the aluminum film are repelled, so the electrons flow from the aluminum film to the transmitting electrode. Opposite to before, positive charged ions in the water are attracted by the transmitting electrode, while negative charged ions are repelled to the surroundings (Supplementary Fig. 1b ). When negative ions contact with the receiving electrode, electrons in the receiving electrode flow to the receiving circuit, so the electrometer detects a negative current.
Figure 2c, d compares the electric signals in air with those in ordinary water. Under acoustic waves (80 Hz, 80 dB), the corresponding periodic output short-circuit current signals yield the peak value of 14.9 μA (Fig. 2b ), which is directly measured with the electrometer connected to the aluminum electrode. When the (electrometer-connected) receiving electrode is two meters away from the submerged transmitting electrode, the peak value of the current decreases slightly to 14.5 μA while the waveform of electric signals remain constant (Fig. 2c ). The peak value of open-circuit voltage output of the TENG decreases from 28.5 V in air to 13 V in water (see Supplementary Fig. 2 ). When the water tank is grounded by a wire, the output current decreases significantly, but the waveform of electric signals stay consistent with the original signal (Supplementary Fig. 3 ). This can be explained by the tendency that charges from ground would balance the electrical potential field in water. Furthermore, the current signal could still be measured even when the transmitting electrode is insulated from water by a Kapton tape (Supplementary Fig. 4 ), and the electric field generated by the TENG can propagate across both gas and liquid media (Supplementary Fig. 5 ). These prove that the transmission of the signals depends on the electric field radiated by the TENG rather than the direct exchange of electrons between water and electrode plates. Both theoretical analysis and experiments have shown that for the whole system, the propagation of underwater electric field has demonstrated the characteristic of displacement current (see Supplementary Note 6 ). Previous study 29 proved that when the electric field propagates in a medium, conduction current dominates when \({{{{{\boldsymbol{\sigma }}}}}}{{{{{\boldsymbol{/}}}}}}{{{{{\boldsymbol{\omega }}}}}}\; > \;{{\varepsilon }}\) and displacement current dominates when \({{\varepsilon }}\, > \,{{{{{\boldsymbol{\sigma }}}}}}{{{{{\boldsymbol{/}}}}}}{{{{{\boldsymbol{\omega }}}}}}\) (σ is conductivity, ω is angular frequency, and ε is permittivity). The Rayleigh distance of the TENG generated electric field can be calculated by \({{{{{\boldsymbol{R}}}}}}=2{{{{{{\boldsymbol{D}}}}}}}^{{{{{{\boldsymbol{2}}}}}}}{{{{{\boldsymbol{/}}}}}}{{{{{\boldsymbol{\lambda }}}}}}\) , where R is Rayleigh distance, D is the maximum size of the transmitting electrode, and λ is the wave length. As a result, R is so small that it can be neglected. According to these theories, for the TENG-based underwater electric field communication, \({{\varepsilon }}\, > \,{{{{{\boldsymbol{\sigma }}}}}}{{{{{\boldsymbol{/}}}}}}{{{{{\boldsymbol{\omega }}}}}}\) and the transmitting distance is larger than the Rayleigh distance. So the displacement current domains the underwater electric field while conduction current only appears in a very short distance.
It is worth mentioning that underwater communication can also be realized by various types of TENGs, such as the TENGs that harvest wave energy and vibration energy (see Supplementary Fig. 6 , and the first and second items in Supplementary Table 1 ). The development of the electric field communication depends on the development of TENG technology. The techniques for designing TENGs with high frequency and good output performance (see Supplementary Table 1 ) provides good potential for the application of the TENG in underwater communication 30 , 31 , 32 , 33 , 34 , 35 , 36 , 37 .
Transmission performance of the underwater electric field
The characteristics of the underwater electric field under different parameters such as water volume, electrode position/size, salinity, water turbidity, and underwater obstacles, have been studied. Figure 3 shows the attenuation of the underwater electric field. In a 3 m × 2 m × 0.4 m water tank (Fig. 3a ), the receiving electrode is placed certain distance away from the transmitting electrode. As the distance increases from 1 m to 3 m, the current signals remain almost unchanged (Fig. 3b ). This is also verified by the simulation of underwater polarization electric field shown in Supplementary Fig. 7 . Furthermore, the complete signals output by the TENG and received signals underwater, and their Fourier transforms are shown in Supplementary Figs. 8 – 9 , showing that the frequency-domain features of the signals remain unchanged underwater. As more water is added into the tank, the peak value of the current decreases. Actually, the peak value decreases by 30% from the original signals as the water volume reaches 6 m 3 (Fig. 3c ). This can be understood, as the electric field energy W in water is
According to Eq. ( 7 ), the energy density of the electric field decreases with the water volume V while using a TENG with constant power output. Therefore, the peak value of the current decreases with larger water volume.
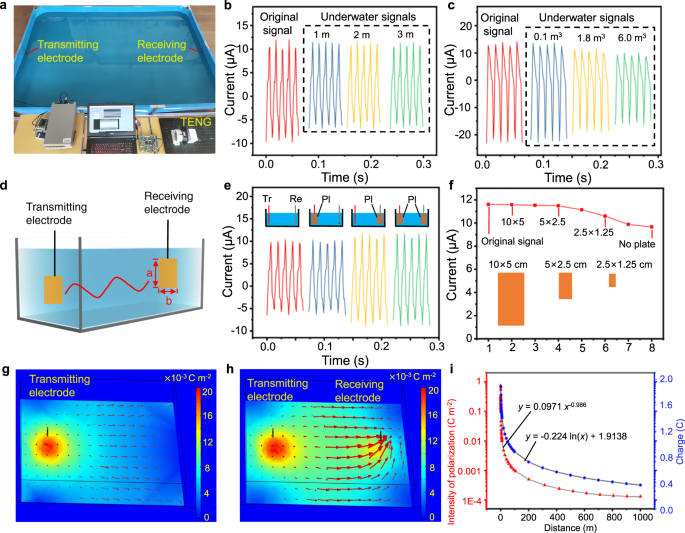
a A photo of the experiment. b Comparison between the original TENG short-circuit current and received current signals in water. c Comparison between the original short-circuit current of the TENG and received signals under different water volume. d Schematic diagram of the electrode. e Comparison of the current signals received in water with different transmitting and receiving electrodes. Tr, Re, and Pl represents transmitting electrode, receiving electrode, and electrode plate respectively. f Comparison of the peak values of the received current signals with different receiving electrodes. Simulation diagram of the distribution of polarization electric field ( g ) without and ( h ) with the receiving electrode. Color represents polarization intensity. i Variation of polarization electric field and charges on the receiving electrode with the distance between the two electrodes.
As shown in Fig. 3d–f , the current can be enhanced by using a receiving electrode plate of larger area. With a 10 cm × 5 cm electrode plate, the peak value of the current signal increases by 18%, compared to that with a thin electric wire. This means more ions in water contacting with the electrode, which is verified by the underwater polarization electric field simulation shown in Supplementary Fig. 10 . In addition, the peak value of the current is subject to the angle between the transmitting and receiving electrode plates, but the effect caused by the angle is very small in the water tank (see Supplementary Figs. 11 – 12 ), which is quite different from the case in a bipolar electric field 38 .
By comparing the distribution of the polarization electric fields without and with the receiving electrode (Fig. 3g, h ), it is found that the receiving electrode can change the distribution of the polarization electric field. This can be explained by the fact that the electrode is equivalent to a terminal with a potential of zero voltage. The variation of the polarization electric field and the variation of the terminal charges in the receiving electrode are shown in Fig. 3i . A 2D simulation is performed and the polarization electric field distribution corresponding to Fig. 3i is shown in Supplementary Figs. 13 and 14 . The attenuation of the polarization electric field and terminal charges at the receiving electrode with distance can be fitted respectively
k 1 , k 2 , k 3 and a are parameters in the fitted curves of the output power of the TENG (Supplementary Figs. 15 and 16 ). According to the simulation results, the exponent of x is close to −1 for two dimension simulations (Supplementary Figs. 15 and 16 ), and close to −2 for three dimension simulations (Supplementary Figs. 17 and 18 ), which is consistence with the Gauss’s law.
The dependence of the underwater electric field on disturbances in water is shown in Fig. 4 . It is found that the peak value of the current signal in salty water (5 g L −1 , adjusted by adding salt to water) increases by 40% on top of that in pure water (Fig. 4a ). This indicates that the ions in salt water can enhance the polarization electric field. However, water salinity increase beyond 15 g L −1 will not further promote the current signals. Similarly, when acid or alkali is added to the pure water to change the pH, the ion concentration in the water will change, indicating that as the pH of water deviates from 7, the received current signals will increase (Supplementary Fig. 19 ). This may be attributed to the improved relative permittivity of the aqueous solution. Figure 4b, c reveal that the waveform of the received signals identical to original ones, regardless of obstacles or turbidity in the water tank. In this sense, the polarization electric field has shown robustness to obstacles and water turbidity.
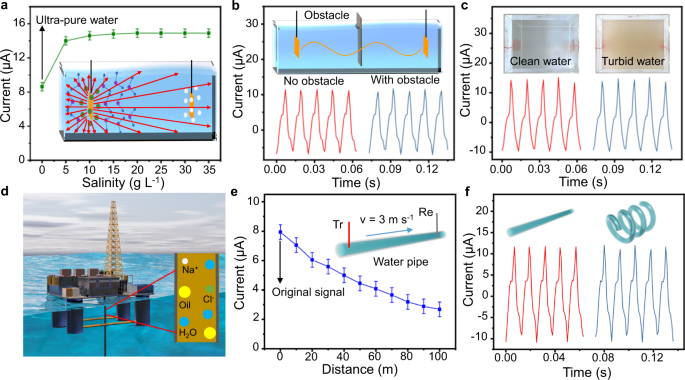
a Effect of water salinity on the peak value of the current. Error bars indicate standard deviations, with all values ≤0.53. b Influence of an obstacle on the current signals. c Comparison between the received current signals in clean water and those in turbid water. d Schematic diagram of the drilling platform with oil pipeline. e Variation of the peak value of short-circuit current output transmitted in a water pipe of 100 meters. Tr and Re represent transmitting and receiving electrodes respectively. v is the liquid flowing speed. Error bars indicate standard deviations, with all values ≤0.46. f Comparison between the short-circuit current signals transmitted in a straight and those in a curved water pipe.
As achieving reliable communication across the pipe is very important for the pipeline robot system 39 , 40 , the performance of the polarization electric field in liquid pipes is investigated (Fig. 4d–f ). Figure 4d is a schematic diagram of the drilling platform with the oil pipeline. In a pipe filled with salt water, the peak value of the current is also found to decreases with the distance between the transmitting electrode and receiving electrode. In fact, the value decreases by 66% when the distance in-between is 100 m (Fig. 4e ), which is consistent with the simulation result of the polarization electric field in the pipe (as shown in Supplementary Fig. 20 ).
In fact, the collision between ions and water molecules may influence the performance of the electric field. In addition, it is interesting to find that the received signals in a spiral pipe are the same with those in a straight pipe (Fig. 4f ). From Supplementary Fig. 21 , it is found that independent of the flow status, the current signals can also be obtained in the mixture of oil and water, which means the polarization electric field communication can be applied in complex pipelines.
It is worth noting that the electric field communication is also insensitive to water temperature and ambient lightness (Supplementary Fig. 22 ). Further comparisons between acoustic, optical, and electromagnetic waves methods are shown in Supplementary Table 2 . What’s more, by studying the effect of the ground on the electric field, it is theoretically proved that this system may work in open water area as shown in Supplementary Note 7 .
Modulation and demodulation of the underwater electric field communication
The modulation and demodulation process of current signals for data transmission in water is shown in Fig. 5 . The current signals converted from sound waves by the TENG can be modulated to digital signals containing the information of texts or images in water via the electric field communication (Fig. 5a ). The signal modulation method is based on the on-off keying (OOK), in which longer signals with time intervals of 50 ms is set as “1”, and shorter signals with time intervals of 25 ms is set as “0”. A 25 ms interval is inserted between each digital signal to separate adjacent digital signals. After transmission in water, the modulated digital signals can be received by the electrometer (Fig. 5b ). The fundamental frequency of the signals generated by the TENG is 80 Hz, and the frequency of the modulated digital signals is 16 Hz. Alternatively, the digital signals can be modulated with other frequencies or other methods (see Supplementary Fig. 23a, b ). Higher frequency yields a fast information transmission rate, while lower frequency yields a strong anti-interference ability.
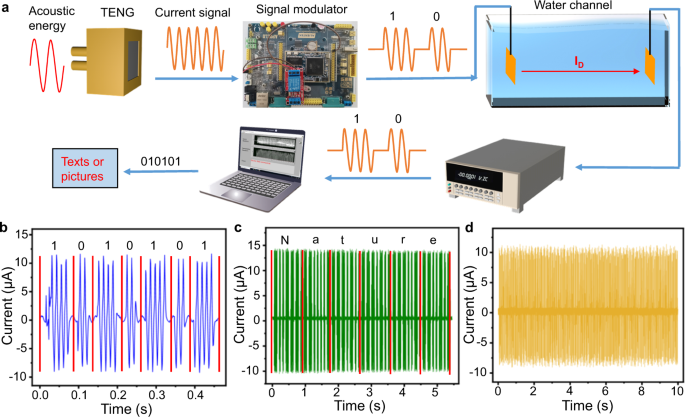
a Schematic diagram of the modulation and demodulation process. b The modulated digital signals transmitted in water. c The demodulated current signals to a word after transmitting in water. d Part of current signals transmitted for an image.
By demodulating the received signal (with the MATLAB codes), the signals of “0” and “1” can be identified accurately (Fig. 5b and Supplementary Fig. 23c ). The current signals can be modulated into text by the standard encoding. The received signals can be accurately demodulated into the original text (Fig. 5c ). Supplementary Movie 1 shows that the real-time current signals generated by the TENG is modulated, transmitted, and demodulated, and the text obtained after demodulation is displayed on a computer screen. This electric field communication can also be used for image transmission. Figure 5d shows part of the received current signals, and the complete signals are shown in Supplementary Fig. 23d . A 2.7-KB image is transmitted within 1353 s at 16 bits/s (owing to the low fundamental frequency of the TENG). There is no error signal in the continuous transmission of ~20,000 digital signals (100,000 working cycles of the TENG). In addition, by applying an external alternating current on the dielectric material’s electrode, the electric signals with higher frequencies (up to kilohertz) can be modulated and transmitted in water, demonstrating that this approach can be used for high frequencies communication (see Supplementary Note 8 ).
It should be noted that the current signals output by the TENG and received signals underwater at a range of 60–200 Hz are compared. It turns out that the signals received underwater are always consistent in waveforms with the signals output by the TENG (see Supplementary Fig. 24 ). What’s more, the power spectrum is obtained by performing Fourier transform to the modulated digital signals and noise. The power spectrum shows that energy is evenly distributed in the frequency range from 40 kHz to 85 kHz (see Supplementary Fig. 25 ), proving that the bandwidth of the system with water channel is greater than 85 kHz.
Realization of wireless control using the underwater electric field communication
To further study the ability of the underwater wireless communication, a demo voice control of an underwater lighting system is performed (Fig. 6 ). A microphone-style TENG that converts voice to electrical signals is to control the underwater lighting system wirelessly (Fig. 6a ). The signals containing the voice information (e.g. “red” and “green”) are transmitted in water and received by the electrometer (Fig. 6b ). By performing short-time Fourier transform to the signals, the words “red” and “green” can be distinguished with a neural network algorithm (see Fig. 6c and Supplementary Fig. 26 ). Subsequently, the words are converted into digital signals to control the lights. This approach can be applied in the real-time voice control of underwater lights (Fig. 6d and Supplementary Movie 2 ). It is worth mentioning that the entire underwater communication realized by the TENG is self-powered.
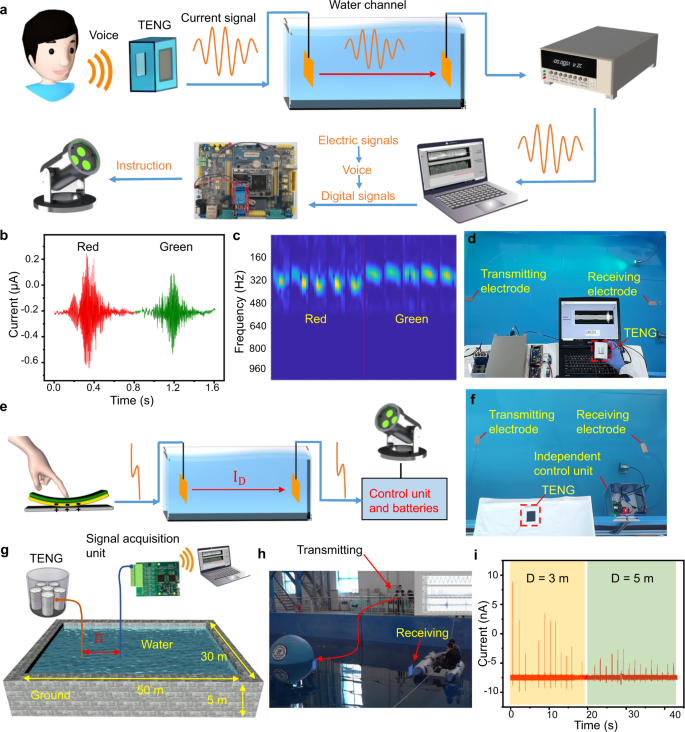
a The schematic diagram of underwater light wirelessly controlled by voice. b The received signals of “red” and “green”. c The short-time Fourier transform of “red” and “green”. Colors represent amplitudes. d The photo of the voice control experiment setup. e The experiment of the button-type TENG controlling an independent system. f The photo of the touch control experiment. g The schematic diagram and ( h ) The photo of the experiment in a 50 m × 30 m × 5 m basin. D is the distance between two electrodes. i The received current signals underwater.
At the same time, the signals receiving and controlling device in the water can be designed independently. By touching a (contact-separation mode) button-type TENG, people can use the electric to control the independent working system in water (see Fig. 6e, f and Supplementary Movie 3 ). The independent working system consists of a weak current acquisition board, a single-chip microcomputer, batteries, a relay, and an underwater working light. The pulse signals generated by the TENG are collected by the weak current acquisition board, and the analog signals are converted into digital signals sent to the microcontroller. The single-chip microcomputer processed the digital signals and controlled the underwater working light.
In another demo experiment, a sandwich-like TENG (S-TENG) with an output current of 60 μA is deployed in a 50 m × 30 m × 5 m basin (with all boundaries connected to the ground, see Fig. 6g, h . When the S-TENG is shaken, the current signals outputted by the S-TENG can be transmitted in water and received by the receiving electrode 5 m away from the transmitting electrode. The signals are detected by a current acquisition board (Supplementary Note 9 ), which sends the signals to a computer through WiFi and then the waveforms are displayed on the screen (Fig. 6i and Supplementary Movie 4 ).
In summary, an underwater communication via Maxwell’s displacement current is proposed and investigated. In the Maxwell’s displacement current, the first term ∂ E /∂ t gives rise to electromagnetic waves. However, in underwater environments, the high-frequency electromagnetic waves can be easily absorbed, and the low-frequency electromagnetic waves can only be transmitted through an antenna of several kilometers. In this study, the second term (∂ P /∂ t ) in the Maxwell’s displacement current is utilized for underwater communication. An acoustic-driven TENG connected to a transmitting electrode is applied to generate alternating electric field in water, so that the sound in air can be converted into underwater electrical signals, which can be measured with a receiving electrode connected to an electrometer. Through a salt water pipe of 100 m length, the peak value of the current signal decreases by 66% compared to the original signal, while the electric signals are not distorted in waveform during transmission.
Based on the on-off keying method, texts and images have been successfully transmitted by modulated current signals in a water tank at 16 bits/s. Throughout the continuous transmission of about 20,000 digital signals, no error appears. By successfully converting voices into current signals, the TENG is capable of controlling an underwater lighting system wirelessly. What’s more, the current signals output by a sandwich-like TENG can be transmitted in a 50 m × 30 m × 5 m basin with the signals displayed on screen in real time. Compared to traditional sonic, optical, and electromagnetic communications, the underwater communication via Maxwell’s displacement current appears to be less vulnerable to disturbances, which exhibits considerable potential for applications in complex underwater environments.
Fabrication of the TENGs
The HR-TENG in the experiments consists of a Helmholtz resonant cavity, an aluminum film with acoustic holes, and an FEP film with a conductive ink-printed electrode. The resonant cavity has a dimension of 73 mm × 73 mm × 40 mm. Two tubes with an inner diameter of 5.0 mm and a length of 32 mm are fixed on the resonant cavity. The aluminum film with 440 uniformly distributed acoustic holes acts as the electropositive triboelectric layer. The length, width, and thickness of the film are 45 mm, 45 mm, and 0.1 mm, respectively and the diameter of the holes is 0.5 mm. The FEP film is used as the electronegative triboelectric layer on observation of its strong electronegativity and good flexibility. It has a thickness of 12.5 μm and a working area of 45 mm × 45 mm. Given that the FEP material is insulated, a conductive ink electrode with a micron thickness is attached to the other side of the FEP film to transfer electrons. A screen printing device (Tou) is used to print the conductive ink (CH-8(MOD2)) on the FEP film (WitLan). The shell is printed by a 3D printer with PLA material.
The TENG to recognize voice is similar to the HR-TENG, except that it has no dule-tube structure but has a 45 mm × 45 mm opening on one side of the resonance cavity. The contact separation distance between the FEP and aluminum film is ~0.2 mm. The membrane structure of the button-type TENG is the same as HR-TENG without a cavity.
The acrylic plate of a single layer S-TENG is of 5 mm thickness and 10 cm diameter. Two aluminum electrodes with a thickness of 50 µm and an area of 6 cm × 4 cm are parallel attached onto two sides of the acrylic plate. PTFE balls with 10.5 mm diameter are filled between two acrylic plates and they are produced by 3 M company. Each S-TENG unit consists of 10 layers stacked S-TENG in parallel connection and acrylic block shell. The acrylic block shell has 10 cm diameter and 20 cm height. There are four AC output copper ends in an S-TENG unit, one pair at the top and the other pair at the bottom. The buoy consists of 5 S-TENG units as the power module and an acrylic shell as the frame structure. The S- TENG units integrated inside are in parallel connection to make the AC electrical output in-phase and the they are fixed through packing tape.
Experimental process and measuring equipment
The output signals are measured with a Keithley 6514 electrometer. The HR-TENG is mounted on an optical plate with a loudspeaker (JBL), driven by sinusoidal waves from a function generator (YE1311). One electrode of the TENG is grounded and the other electrode is immersed in water. The wires used for electrodes has a 0.3 mm copper core, and the electrode plates are copper films with the size of 100 × 50 × 0.06 mm. The water pipe used in the experiment is an ordinary PVC rubber water pipe with an inner diameter of 13 mm. A 12 V DC motor is used to control the flow of liquid in the pipe. The signal modulator consists of a microcontroller development board (STM32F7) and a relay. The MATLAB interface in LABVIEW is used to demodulate and display the real-time signals measured with the electrometer. The signals generated by the voice-driven TENG need to be filtered at 50 Hz and its harmonics after being received.
The transmitting electrode in water and the aluminum electrode of the TENG is connected by an ordinary copper wire. The HR-TENG is applied to convert sound in air to electrical signals in water. In this way, acoustic waves with specific frequencies can be got by controlling the signal generator and a loudspeaker. Furthermore, under the excitation of the acoustic waves, electrical signals with specific frequencies can be generated by the HR-TENG to examine the performance of the underwater communication. Button type TENG is a basic and commonly used TENG with the simplest structure. The application of the button type TENG prove that underwater communication can be realized by general TENGs, demonstrating the application potential of this approach.
Numerical simulations
In order to verify the accuracy of the derived theory and experimental results, COMSOL Multiphysics software has been used for numerical simulations. The AD/DC modules, electrostatic interfaces, and transient state analysis are used in simulations. The distribution of the polarization electric field and terminal charge of the receiving electrode has been simulated. As the element size influences the calculation results, the ultra-fineness meshing option has been adopted in the simulation. For 3-D simulations, the size of the model is limited to 15 m. The 2-D simulation is used to analyze the attenuation of the electric field through longer distance. The error of the 3D simulation depends on the mesh size and model scale.
Data availability
The data supporting this study are available within the article and the Supporting Information . Source data are provided with this paper.
Ijspeert, A. J. Biorobotics: using robots to emulate and investigate agile locomotion. Science 346 , 196–203 (2014).
Article ADS CAS Google Scholar
Chen, Y., Doshi, N., Goldberg, B., Wang, H. & Wood, R. J. Controllable water surface to underwater transition through electrowetting in a hybrid terrestrial-aquatic microrobot. Nat. Commun. 9 , 2495 (2018).
Article ADS Google Scholar
Krieg, M., Nelson, K. & Mohseni, K. Distributed sensing for fluid disturbance compensation and motion control of intelligent robots. Nat. Mach. Intell. 1 , 216–224 (2019).
Article Google Scholar
Zou, Y. et al. A bionic stretchable nanogenerator for underwater sensing and energy harvesting. Nat. Commun. 10 , 2695 (2019).
Jaffe, J. S. et al. A swarm of autonomous miniature underwater robot drifters for exploring submesoscale ocean dynamics. Nat. Commun. 8 , 14189 (2017).
Katzschmann, R. K., Delpreto, J., Maccurdy, R. & Rus, D. J. S. R. Exploration of underwater life with an acoustically controlled soft robotic fish. Sci. Robot. 3 , eaar3449 (2018).
Hattab, G., El-Tarhuni, M., Al-Ali, M., Joudeh, T. & Qaddoumi, N. An underwater wireless sensor network with realistic radio frequency path loss model. Int. J. Distrib. Sens. Netw. 9 , 188–192 (2013).
Saeed, N., Celik, A., Al-Naffouri, T. Y. & Alouini, M.-S. Underwater optical wireless communications, networking, and localization: a survey. Ad Hoc Netw. 94 , 101935 (2019).
Kilfoyle, D. B. & Baggeroer, A. B. The state of the art in underwater acoustic telemetry. IEEE J. Ocean. Eng. 25 , 4–27 (2000).
Wang, W., Liu, J., Xie, G., Wen, L. & Zhang, J. A bio-inspired electrocommunication system for small underwater robots. Bioinspir Biomim. 12 , 036002 (2017).
Simpson, J. A., Hughes, B. L. & Muth, J. F. Smart transmitters and receivers for underwater free-space optical communication. IEEE J. Sel. Areas Commun. 30 , 964–974 (2012).
Chen, Y. T., Wang, W., Li, L., Kelly, R. & Xie, G. Obstacle effects on electrocommunication with applications to object detection of underwater robots. Bioinspir Biomim. 14 , 056011 (2019).
Akyildiz, I. F., Wang, P. & Sun, Z. Realizing underwater communication through magnetic induction. IEEE Commun. Mag. 53 , 42–48 (2015).
Che, X., Wells, I., Dickers, G., Kear, P. & Gong, X. Re-evaluation of RF electromagnetic communication in underwater sensor networks. IEEE Commun. Mag. 48 , 143–151 (2010).
Wang, Z. L. On the first principle theory of nanogenerators from Maxwell’s equations. Nano Energy 68 , 104272 (2020).
Article CAS Google Scholar
Wang, Z. L. Triboelectric nanogenerator (TENG)—sparking an energy and sensor revolution. Adv. Energy Mater. 10 , 2000137 (2020).
Wang, Z. L. Nanogenerators, self-powered systems, blue energy, piezotronics and piezo-phototronics – a recall on the original thoughts for coining these fields. Nano Energy 54 , 477–483 (2018).
Wang, Z. L. On Maxwell’s displacement current for energy and sensors: the origin of nanogenerators. Mater. Today 20 , 74–82 (2017).
Qiu, C., Wu, F., Lee, C. & Yuce, M. R. Self-powered control interface based on Gray code with hybrid triboelectric and photovoltaics energy harvesting for IoT smart home and access control applications. Nano Energy 70 , 104456 (2020).
Wu, Z. et al. A hybridized triboelectric-electromagnetic water wave energy harvester based on a magnetic sphere. ACS nano 13 , 2349–2356 (2019).
CAS PubMed Google Scholar
Xiao, T. X. et al. Silicone-based triboelectric nanogenerator for water wave energy harvesting. ACS Appl. Mater. Interfaces 10 , 3616–3623 (2018).
Wang, Y. et al. Multi-functional wind barrier based on triboelectric nanogenerator for power generation, self-powered wind speed sensing and highly efficient windshield. Nano Energy 73 , 104736 (2020).
Wang, F. et al. Windmill-inspired hybridized triboelectric nanogenerators integrated with power management circuit for harvesting wind and acoustic energy. Nano Energy 78 , 105244 (2020).
Wang, Z. L. Triboelectric nanogenerators as new energy technology for self-powered systems and as active mechanical and chemical sensors. ACS Nano 7 , 9533–9557 (2013).
Yang, H. et al. Triboelectric micromotors actuated by ultralow frequency mechanical stimuli. Nat. Commun. 10 , 2309 (2019).
Wang, H. et al. A paradigm shift fully self-powered long-distance wireless sensing solution enabled by discharge-induced displacement current. Sci. Adv. 7 , eabi6751 (2021).
Zhao, H. et al. Dual‐tube helmholtz resonator‐based triboelectric nanogenerator for highly efficient harvesting of acoustic energy. Adv. Energy Mater. 9 , 1902824 (2019).
Yi, H. & Xiong, L. The effect of the electric field on the output performance of triboelectric nanogenerators. J. Comput. Electron. 19 , 1670–1677 (2020).
Al-Shamma’a, A. I., Shaw, A. & Saman, S. Propagation of electromagnetic waves at MHz frequencies through seawater. IEEE Trans. Antennas Propag. 52 , 2843–2849 (2004).
Xu, M. et al. High power density tower-like triboelectric nanogenerator for harvesting arbitrary directional water wave energy. ACS nano 13 , 1932–1939 (2019).
Xiao, X. et al. Honeycomb structure inspired triboelectric nanogenerator for highly effective vibration energy harvesting and self‐powered engine condition monitoring. Adv. Energy Mater. 9 , 1902460 (2019).
Wang, H. et al. Sandwich-like triboelectric nanogenerators integrated self-powered buoy for navigation safety. Nano Energy 84 , 105920 (2021).
Xi, Y. et al. High efficient harvesting of underwater ultrasonic wave energy by triboelectric nanogenerator. Nano Energy 38 , 101–108 (2017).
Chen, C. et al. Micro triboelectric ultrasonic device for acoustic energy transfer and signal communication. Nat. Commun. 11 , 4143 (2020).
Vivekananthan, V., Chandrasekhar, A., Alluri, N. R., Purusothaman, Y. & Kim, S.-J. A highly reliable, impervious and sustainable triboelectric nanogenerator as a zero-power consuming active pressure sensor. Nanoscale Adv. 2 , 746–754 (2020).
Sahu, M. et al. Synergetic enhancement of energy harvesting performance in triboelectric nanogenerator using ferroelectric polarization for self-powered IR signaling and body activity monitoring. J. Mater. Chem. A 8 , 22257–22268 (2020).
Chandrasekhar, A., Alluri, N. R., Vivekananthan, V., Park, J. H. & Kim, S.-J. Sustainable biomechanical energy scavenger toward self-reliant kids’ interactive battery-free smart puzzle. ACS Sustain. Chem. Eng. 5 , 7310–7316 (2017).
Joe J., Toh S. H. OCEANS 2007 - Europe (Aberdeen, UK, 2007).
Qi, H., Zhang, X., Chen, H. & Ye, J. Tracing and localization system for pipeline robot. Mechatronics 19 , 76–84 (2009).
Lanbo, L., Shengli, Z. & Jun-Hong, C. Prospects and problems of wireless communication for underwater sensor networks. Wirel. Commun. Mob. Comput. 8 , 977–994 (2008).
Download references
Acknowledgements
The authors are grateful for the joint support from the National Key R & D Project from Minister of Science and Technology (2021YFA1201600, Z.L.W.), the National Natural Science Foundation of China (Grant Nos. 51879022, 51979045, M.Y.X.), the Fundamental Research Funds for the Central Universities, China (Grant No. 3132019330, M.Y.X.), and Tsinghua-Foshan Innovation Special Fund (TFISF, Grant No. 2020THFS0109, W.B.D.).
Author information
These authors contributed equally: Hongfa Zhao, Minyi Xu, Mingrui Shu.
Authors and Affiliations
Marine Engineering College, Dalian Maritime University, 116026, Dalian, China
Hongfa Zhao, Minyi Xu, Mingrui Shu, Xiangyu Liu, Siyuan Wang, Cong Zhao, Hongyong Yu, Hao Wang, Chuan Wang, Xianping Fu, Xinxiang Pan & Guangming Xie
Tsinghua-Berkeley Shenzhen Institute, Tsinghua Shenzhen International Graduate School, Tsinghua University, 518055, Shenzhen, China
Hongfa Zhao & Wenbo Ding
Beijing Institute of Nanoenergy and Nanosystems, Chinese Academy of Sciences, 100085, Beijing, China
Jie An & Zhong Lin Wang
Southern Marine Science and Engineering Guangdong Laboratory (Guangzhou), Guangzhou, 511458, P. R. China
Guangming Xie
College of Engineering, Peking University, Beijing, 100871, P.R. China
School of Materials Science and Engineering, Georgia Institute of Technology, Atlanta, GA, 30332-0245, USA
- Zhong Lin Wang
You can also search for this author in PubMed Google Scholar
Contributions
M.X., Z.L.W., and G.X. supervised and guided the project; H.Z., M.X., M.S., and H.W. conceived the idea and designed the experiment. H.Z., J.A., C.Z., S.W., and H.Y. fabricated the devices and performed the experiments; H.Z., X.L., and C.W. did the theoretical calculation; H.Z, W.D., X.F., and X.P. discussed the experiment and results; H.W., M.X., and H.Z. wrote the manuscript. All authors discussed and reviewed the manuscript.
Corresponding authors
Correspondence to Minyi Xu , Guangming Xie or Zhong Lin Wang .
Ethics declarations
Competing interests.
The authors declare no competing interests.
Peer review
Peer review information.
Nature Communications thanks Xuhui Sun, Muhammad Tahir and the other, anonymous, reviewer(s) for their contribution to the peer review of this work.
Additional information
Publisher’s note Springer Nature remains neutral with regard to jurisdictional claims in published maps and institutional affiliations.
Supplementary information
Supplementary information, description of additional supplementary files, supplementary movie 1, supplementary movie 2, supplementary movie 3, supplementary movie 4, source data, source data, rights and permissions.
Open Access This article is licensed under a Creative Commons Attribution 4.0 International License, which permits use, sharing, adaptation, distribution and reproduction in any medium or format, as long as you give appropriate credit to the original author(s) and the source, provide a link to the Creative Commons license, and indicate if changes were made. The images or other third party material in this article are included in the article’s Creative Commons license, unless indicated otherwise in a credit line to the material. If material is not included in the article’s Creative Commons license and your intended use is not permitted by statutory regulation or exceeds the permitted use, you will need to obtain permission directly from the copyright holder. To view a copy of this license, visit http://creativecommons.org/licenses/by/4.0/ .
Reprints and permissions
About this article
Cite this article.
Zhao, H., Xu, M., Shu, M. et al. Underwater wireless communication via TENG-generated Maxwell’s displacement current. Nat Commun 13 , 3325 (2022). https://doi.org/10.1038/s41467-022-31042-8
Download citation
Received : 24 March 2021
Accepted : 31 May 2022
Published : 09 June 2022
DOI : https://doi.org/10.1038/s41467-022-31042-8
Share this article
Anyone you share the following link with will be able to read this content:
Sorry, a shareable link is not currently available for this article.
Provided by the Springer Nature SharedIt content-sharing initiative
This article is cited by
Superlensing enables radio communication and imaging underwater.
- Igor I. Smolyaninov
- Quirino Balzano
- Dendy Young
Scientific Reports (2023)
Triboelectric nanogenerators
- Tinghai Cheng
- Jiajia Shao
Nature Reviews Methods Primers (2023)
A Broad Range Triboelectric Stiffness Sensor for Variable Inclusions Recognition
- Zhentan Quan
Nano-Micro Letters (2023)
By submitting a comment you agree to abide by our Terms and Community Guidelines . If you find something abusive or that does not comply with our terms or guidelines please flag it as inappropriate.
Quick links
- Explore articles by subject
- Guide to authors
- Editorial policies
Sign up for the Nature Briefing newsletter — what matters in science, free to your inbox daily.

Suggestions or feedback?
MIT News | Massachusetts Institute of Technology
- Machine learning
- Social justice
- Black holes
- Classes and programs
Departments
- Aeronautics and Astronautics
- Brain and Cognitive Sciences
- Architecture
- Political Science
- Mechanical Engineering
Centers, Labs, & Programs
- Abdul Latif Jameel Poverty Action Lab (J-PAL)
- Picower Institute for Learning and Memory
- Lincoln Laboratory
- School of Architecture + Planning
- School of Engineering
- School of Humanities, Arts, and Social Sciences
- Sloan School of Management
- School of Science
- MIT Schwarzman College of Computing
Device offers long-distance, low-power underwater communication
Press contact :, media download.
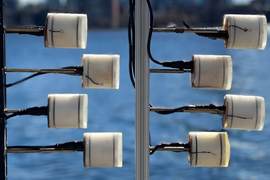
*Terms of Use:
Images for download on the MIT News office website are made available to non-commercial entities, press and the general public under a Creative Commons Attribution Non-Commercial No Derivatives license . You may not alter the images provided, other than to crop them to size. A credit line must be used when reproducing images; if one is not provided below, credit the images to "MIT."
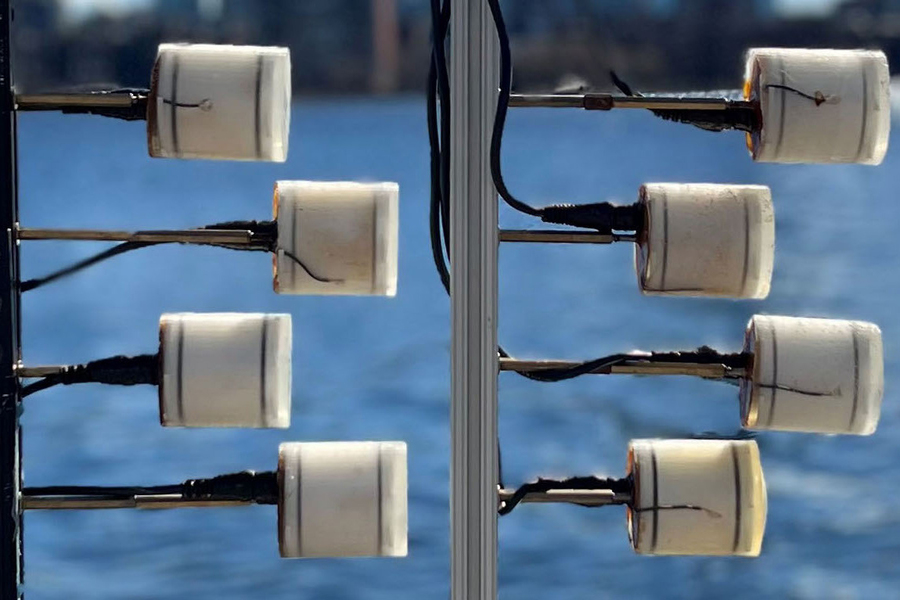
Previous image Next image
MIT researchers have demonstrated the first system for ultra-low-power underwater networking and communication, which can transmit signals across kilometer-scale distances.
This technique, which the researchers began developing several years ago, uses about one-millionth the power that existing underwater communication methods use. By expanding their battery-free system’s communication range, the researchers have made the technology more feasible for applications such as aquaculture, coastal hurricane prediction, and climate change modeling.
“What started as a very exciting intellectual idea a few years ago — underwater communication with a million times lower power — is now practical and realistic. There are still a few interesting technical challenges to address, but there is a clear path from where we are now to deployment,” says Fadel Adib, associate professor in the Department of Electrical Engineering and Computer Science and director of the Signal Kinetics group in the MIT Media Lab.
Underwater backscatter enables low-power communication by encoding data in sound waves that it reflects, or scatters, back toward a receiver. These innovations enable reflected signals to be more precisely directed at their source.
Due to this “retrodirectivity,” less signal scatters in the wrong directions, allowing for more efficient and longer-range communication.
When tested in a river and an ocean, the retrodirective device exhibited a communication range that was more than 15 times farther than previous devices. However, the experiments were limited by the length of the docks available to the researchers.
To better understand the limits of underwater backscatter, the team also developed an analytical model to predict the technology’s maximum range. The model, which they validated using experimental data, showed that their retrodirective system could communicate across kilometer-scale distances.
The researchers shared these findings in two papers which will be presented at this year’s ACM SIGCOMM and MobiCom conferences. Adib, senior author on both papers, is joined on the SIGCOMM paper by co-lead authors Aline Eid, a former postdoc who is now an assistant professor at the University of Michigan, and Jack Rademacher, a research assistant; as well as research assistants Waleed Akbar and Purui Wang, and postdoc Ahmed Allam. The MobiCom paper is also written by co-lead authors Akbar and Allam.
Communicating with sound waves
Underwater backscatter communication devices utilize an array of nodes made from “piezoelectric” materials to receive and reflect sound waves. These materials produce an electric signal when mechanical force is applied to them.
When sound waves strike the nodes, they vibrate and convert the mechanical energy to an electric charge. The nodes use that charge to scatter some of the acoustic energy back to the source, transmitting data that a receiver decodes based on the sequence of reflections.
But because the backscattered signal travels in all directions, only a small fraction reaches the source, reducing the signal strength and limiting the communication range.
To overcome this challenge, the researchers leveraged a 70-year-old radio device called a Van Atta array, in which symmetric pairs of antennas are connected in such a way that the array reflects energy back in the direction it came from.
But connecting piezoelectric nodes to make a Van Atta array reduces their efficiency. The researchers avoided this problem by placing a transformer between pairs of connected nodes. The transformer, which transfers electric energy from one circuit to another, allows the nodes to reflect the maximum amount of energy back to the source.
“Both nodes are receiving and both nodes are reflecting, so it is a very interesting system. As you increase the number of elements in that system, you build an array that allows you to achieve much longer communication ranges,” Eid explains.
In addition, they used a technique called cross-polarity switching to encode binary data in the reflected signal. Each node has a positive and a negative terminal (like a car battery), so when the positive terminals of two nodes are connected and the negative terminals of two nodes are connected, that reflected signal is a “bit one.”
But if the researchers switch the polarity, and the negative and positive terminals are connected to each other instead, then the reflection is a “bit zero.”
“Just connecting the piezoelectric nodes together is not enough. By alternating the polarities between the two nodes, we are able to transmit data back to the remote receiver,” Rademacher explains.
When building the Van Atta array, the researchers found that if the connected nodes were too close, they would block each other’s signals. They devised a new design with staggered nodes that enables signals to reach the array from any direction. With this scalable design, the more nodes an array has, the greater its communication range.
They tested the array in more than 1,500 experimental trials in the Charles River in Cambridge, Massachusetts, and in the Atlantic Ocean, off the coast of Falmouth, Massachusetts, in collaboration with the Woods Hole Oceanographic Institution. The device achieved communication ranges of 300 meters, more than 15 times longer than they previously demonstrated.
However, they had to cut the experiments short because they ran out of space on the dock.
Modeling the maximum
That inspired the researchers to build an analytical model to determine the theoretical and practical communication limits of this new underwater backscatter technology.
Building off their group’s work on RFIDs, the team carefully crafted a model that captured the impact of system parameters, like the size of the piezoelectric nodes and the input power of the signal, on the underwater operation range of the device.
“It is not a traditional communication technology, so you need to understand how you can quantify the reflection. What are the roles of the different components in that process?” Akbar says.
For instance, the researchers needed to derive a function that captures the amount of signal reflected out of an underwater piezoelectric node with a specific size, which was among the biggest challenges of developing the model, he adds.
They used these insights to create a plug-and-play model into a which a user could enter information like input power and piezoelectric node dimensions and receive an output that shows the expected range of the system.
They evaluated the model on data from their experimental trials and found that it could accurately predict the range of retrodirected acoustic signals with an average error of less than one decibel.
Using this model, they showed that an underwater backscatter array can potentially achieve kilometer-long communication ranges.
“We are creating a new ocean technology and propelling it into the realm of the things we have been doing for 6G cellular networks. For us, it is very rewarding because we are starting to see this now very close to reality,” Adib says.
The researchers plan to continue studying underwater backscatter Van Atta arrays, perhaps using boats so they could evaluate longer communication ranges. Along the way, they intend to release tools and datasets so other researchers can build on their work. At the same time, they are beginning to move toward commercialization of this technology.
“Limited range has been an open problem in underwater backscatter networks, preventing them from being used in real-world applications. This paper takes a significant step forward in the future of underwater communication, by enabling them to operate on minimum energy while achieving long range,” says Omid Abari, assistant professor of computer science at the University of California at Los Angeles, who was not involved with this work. “The paper is the first to bring Van Atta Reflector array technique into underwater backscatter settings and demonstrate its benefits in improving the communication range by orders of magnitude. This can take battery-free underwater communication one step closer to reality, enabling applications such as underwater climate change monitoring and coastal monitoring.”
This research was funded, in part, by the Office of Naval Research, the Sloan Research Fellowship, the National Science Foundation, the MIT Media Lab, and the Doherty Chair in Ocean Utilization.
Share this news article on:
Press mentions, ieee spectrum.
MIT researchers have developed a new underwater system that could enable long-range and low-power underwater communication, reports Edd Gent for IEEE Spectrum . “The reason why this is really exciting is because now you start opening up many of the coastal monitoring applications,” says Prof. Fadel Adib. “It’s a turning point from this being a technology that is intellectually super interesting that we hope will work, to saying we know that this works and we have a path to deployment.”
Popular Science
Popular Science reporter Andrew Paul writes that MIT researchers have developed a new long-range, low-power underwater communication system. Installing underwater communication networks “could help continuously measure a variety of oceanic datasets such as pressure, CO2, and temperature to refine climate change modeling,” writes Paul, “as well as analyze the efficacy of certain carbon capture technologies.”
Previous item Next item
Related Links
- Signal Kinetics Group
- Department of Electrical Engineering and Computer Science
- School of Architecture and Planning
Related Topics
- Electronics
- Ocean science
- Climate change
- Oceanography and ocean engineering
- Electrical Engineering & Computer Science (eecs)
- National Science Foundation (NSF)
Related Articles
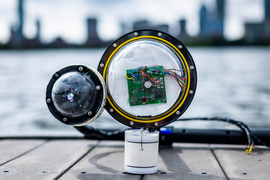
MIT engineers build a battery-free, wireless underwater camera
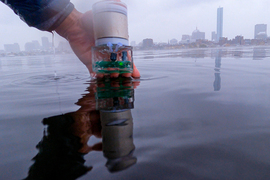
An underwater navigation system powered by sound
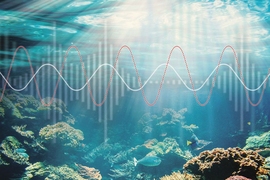
A battery-free sensor for underwater exploration
More mit news.
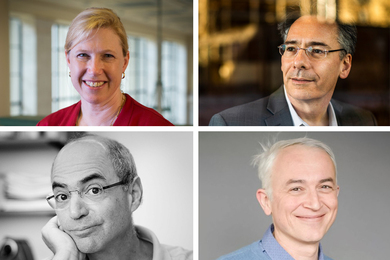
Four MIT faculty named 2023 AAAS Fellows
Read full story →
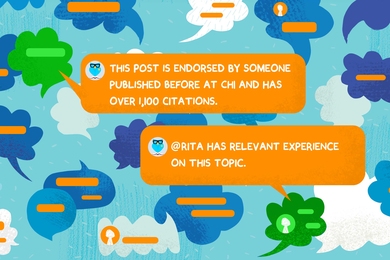
For more open and equitable public discussions on social media, try “meronymity”
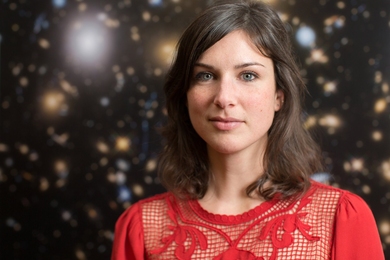
Erin Kara named Edgerton Award winner
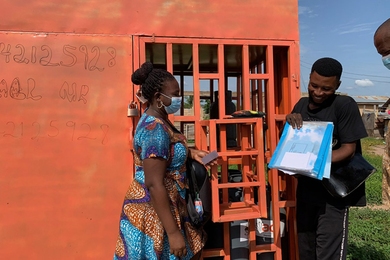

Q&A: Claire Walsh on how J-PAL’s King Climate Action Initiative tackles the twin climate and poverty crises
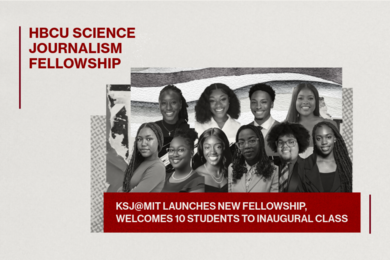
Knight Science Journalism Program launches HBCU Science Journalism Fellowship
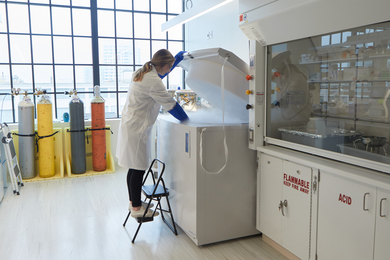
A home where world-changing innovations take flight
- More news on MIT News homepage →
Massachusetts Institute of Technology 77 Massachusetts Avenue, Cambridge, MA, USA
- Map (opens in new window)
- Events (opens in new window)
- People (opens in new window)
- Careers (opens in new window)
- Accessibility
- Social Media Hub
- MIT on Facebook
- MIT on YouTube
- MIT on Instagram
- Reference Manager
- Simple TEXT file
People also looked at
Brief research report article, research on underwater wireless dynamic optical communication system based on ppm modulation.
- 1 National and Local Joint Engineering Research Center of Space Optoelectronics Technology, Changchun University of Science and Technology, Changchun, China
- 2 College of Optoelectronic Engineering, Changchun University of Science and Technology, Changchun, China
In order to achieve underwater wireless dynamic optical communication, a laser communication system is proposed based on Pulse Position Modulation (PPM). In order to achieve underwater laser communication accurately, the mathematical model of underwater laser communication was constructed with small angle analysis. The pulse position modulation demodulation algorithm is designed, and the workflow of modulation and demodulation is given in the transmit module and the receive module. In the experiment, Lumileds-470 nm light source was selected for data communication for testing at a communication rate of 15 Mbp/s. In the servo control process, the square wave signal used for stepping motor drive had a stable amplitude output and a stable time width. It can well simulate the testing process of underwater dynamic scanning. In the experiment, laser light spots were obtained under different attenuation states, and the characteristics of the light spot distribution were analyzed. The numerical reconstruction of the light spot energy was completed in MATLAB. Three types of light attenuators, 1.0%, 0.1%, and 0.01%, were used to simulate different light attenuations underwater. The test results show that the system error rate is better than 10 −6 when attenuation chip is 1.0%. When attenuation chip is 0.1%, the error rate of the system is reduced to 10 −4 . When attenuation chip is 0.01%, a valid signal cannot be obtained by the system. The feasibility of the system is verified.
1 Introduction
Underwater wireless communication technology is widely used in resource exploration [ 1 ], abnormal sea state monitoring [ 2 ], sensor monitoring [ 3 ], underwater imaging [ 4 ], underwater robots [ 5 ], and airspace integration [ 6 ]. It is important advantages for blue or green laser, such as good penetration ability, strong anti-interference, large communication depth, and high transmission rate in seawater. It has become a research hotspot.
The characteristics of underwater laser communication include: a) high transmission rate. Due to the use of high-frequency information transmission, the transmission rate can reach Mbit/s, far due to traditional acoustic equipment. b) Large information capacity. The blue light of laser communication is about 620 THz, which can support the construction of large capacity link systems. It has the underwater transmission requirements of image signals and multi-channel video signals, and its high bit rate is generally around the GHz level. c) Strong anti-interference. The laser transmission process is not affected by electromagnetic interference. It is not significantly affected by seawater temperature, concentration, etc. d) High safety and density. Light wave has strong directionality and small beam divergence angle. If the communication signal is truncated, the receiving end will lose the signal, which is easy to be detected in a timely manner, so its security and confidentiality are very good. e) The system structure is compact. Underwater laser communication systems have a compact structure and low power, making them more concealable.
Duntley [ 7 ] found that lasers with a wavelength range of 470–525 nm can effectively reduce the light wave absorption of seawater. Shimura S [ 8 ] had experimentally verified that the visible light band has a low loss window, and its propagation loss was only 1% of that of other light waves. This discovery will have greatly advanced for the underwater laser communication research. Tivey et al. [ 9 ] developed an underwater wireless communication system. It still had the ability to transmit data underwater. The system achieves effective communication of signals within a range of 5 m. Huang A P et al. [ 10 ] designed an optical communication system with acoustic systems. This system used low-power receivers and it could achieve underwater data transmission of 10–20 Mbit/s within 100 m. Campagnaro F et al. [ 11 ] used a 405 nm wavelength laser to conduct communication tests in 4.8 m long clear water, when the communication rate is 1.45 Gbit/s, the bit error rate is only 9.1 × 10 −4 . Fu et al. [ 12 ] studied the impact of communication aperture on the bit error rate of underwater wireless optical communication systems under medium intensity turbulence. Han et al. [ 13 ] used electro-optical crystal modulation to generate communication signals, and completed the extinction characteristics of underwater communication systems on modulated signals by Monte Carlo method. Based on the analysis of transmission link span and laser divergence angle, Vali et al. [ 14 ] analyzed and discussed the effects of system aperture and field of view on underwater communication under turbulent conditions.
In summary, this paper studies an underwater laser communication system based on PPM (Pulse Position Modulation [ 15 ]) modulation. The system uses a servo mechanism to align the optical path at both ends of the laser communication, then uses PPM modulation technology to complete data communication, and simulates underwater communication by using an attenuator.
2 Underwater laser communication system design
In this paper, a blue laser communication experimental system based on PPM modulation and demodulation is constructed. The overall structure of the system is shown in Figure 1 .
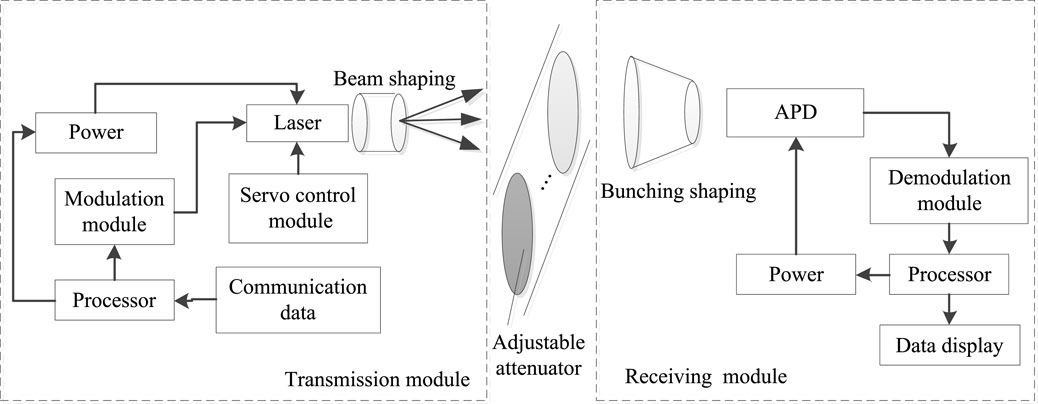
FIGURE 1 . Receiving and transmitting module of underwater laser communication system.
The entire laser communication system consists of a transmitter and a receiver. The transmitter is mainly composed of a data transmission module, a PPM modulator, and a laser. The data transmission module is used to input the data to be transmitted and transmit it to the PPM modulator through USB interface. The PPM modulator converts the modulated data into an output signal and drives the laser to emit light through the laser interface. A laser beam emits a signal in the form of a carrier wave. The laser adopts a tunable semiconductor blue laser with a central wavelength of 470 nm and a maximum communication rate of 15 Mbps. In the experiment, in order to simulate the optical attenuation effect from 10 to 20 m underwater, variable optical attenuators were added to the communication path, achieving 30%, 50%, and 80% optical attenuation, respectively. In actual experiments, the distance between the two laser communication modules after using the attenuator will be set to be 2–4 m.
The receiving module is mainly composed of a receiving optical path, a PPM demodulator, and a data receiving terminal. After the certain attenuation, the laser pulse containing modulation information is transmitted to the receiving optical path. After photoelectric conversion is completed by the APD (Avalanche Photodetector [ 16 ]) detector, it is sent to the PPM demodulation module. The demodulated data can be displayed on the LCD panel of the PPM demodulator. Data can also be transferred to a computer through a USB port to complete operations such as storing and recording.
3 Small angle analysis mathematical model
Small angle analysis is a typical method for studying underwater laser propagation [ 17 ]. During communication, the laser transmitter and receiver can achieve good position alignment through a servo mechanism, so their transmission divergence angle is small. The mathematical model is constructed from the particle nature of light, and the scattering process between photons and suspended particles is described using a small angle approximation method, which is more consistent with the actual test situation.
The laser beam is transmitted in water along the z -axis, and the light source coordinates are set to the origin (0, 0, 0). During transmission, seawater molecules and impurity molecules can cause laser light to scatter, assuming this scattering angle is θ i .
The movement displacement L per unit time is
x, y, z represents the numerical value on the corresponding coordinate axis, and t represents time. According to the scattering theory, assume that the single scattering rate is w, the unilateral scattering angle is θ , and the optical thickness is τ . There are
Then r is the photon scattering projection angle.
Due to the small value of r , the above formula can be simplified and approximated as follows:
According to the principle of optical communication [ 18 ], it is possible to calculate the time delay of the small angle analysis method as follows:
Where c is the speed of light and n is the refractive index of the water transmitting medium [ 19 ]. Therefore, the optical power at the receiving end of the system can be expressed as
Then E L is the energy value of the received light. In communication systems, the width of laser pulses affects time domain broadening. After the laser pulse passes through the water, since only the pulse broadening is considered, the shock response of the initial time domain waveform p (t) can be assumed to be P c (t). After the transmission distance z of the initial pulse p (t), the broadened waveform can be expressed as
I(t) represents the energy value that varies with time t. From this energy value, the energy distribution of the communication laser spot at the receiving end can be calculated, thereby completing the inversion of communication efficiency.
4 PPM demodulation algorithm
The PPM communication pulse sequence is composed of several frames, each frame being divided into protection segments and information segments. When a pulse is included in the K time slots of the communication period, the pulse width is set to ΔT. One frame period is equivalent to one optical pulse period, which is also the repetition frequency of the laser.
The protection segment is related to the characteristics of the laser and is the minimum time interval for the laser to reach the threshold condition again. Use J light pulse lengths JΔT represents. K determines the number of bits k of modulated information, which can be expressed as:
In PPM modulation, the transmitted information corresponds to the position of the laser pulse one by one. The analysis of the pulse position can calculate the transmitted data value, which belongs to a phase modulation.
PPM modulation transmits information using a laser pulse from K time slots in the information segment. In a system, a frame period is the period of a light pulse, i.e., (K + J)ΔT. When the energy of a single laser pulse is high, the average power over the entire cycle is very small, reducing the need for average power in signal transmission. In terms of transmission rate, PPM modulation achieves log2M bit data transmission, and its speed can be expressed as
To further improve speed, you can increase the information time slot or reduce the protection time slot. However, this increase and decrease is not arbitrary, and is limited by the characteristics of the laser.
4.1 Transmission module
The laser emission module with a microprocessor as its core achieves timing output through a high-speed timer. Controllable output at a certain port is achieved by controlling the timer amount. Specific process: First, the timer operates in a high-speed timing state, and counts with the minimum resolution time slot. Then, when the algorithm controls the time corresponding to the modulated information data, a pulse is generated, and the rising edge of the pulse is its modulation information. Next, a similar rising edge is generated through the PPM conversion module to control this narrow pulse and complete the PPM modulation output. Finally, the output signal can be connected to the modulation interface of the laser to control the laser to emit a modulated optical pulse sequence.
4.2 Receiving module
The PPM demodulator, as the core device of the reception section, converts the received pulses containing modulation information into slot positions and demodulates the information. Firstly, it is implemented using the comparison and capture module of high-speed timers. As long as the received signal meets the trigger conditions after processing, it can accurately capture the pulse jump edge and achieve accurate timing. Secondly, a high-speed clock is used for timing decisions, and the positions of each pulse are recorded in the form of time counts. Finally, the modulation information is obtained through the corresponding demodulation algorithm.
5 Experiments
5.1 experimental system.
The main structure of the system includes a transmitting light module and a receiving light module. In the receiving module, the computer controls the modulation module to complete the uploading and modulation of data. In the receiving module, the computer controls the demodulation module to complete data download and demodulation. Lumileds-470 nm type light source is selected as the emission laser. At a communication rate of 15 Mbps, a single LED has a luminous power of about 200 mW and a divergence angle of 120°. The output power of the entire LED array is above 1 W, which can meet the system requirements. In the receiving module, the APD detector selected by Hamamatsu Company is C12702-12. The processing part uses ALINX’s AN108 A/D module and FPGA development board to work together. The system is shown in Figure 2 .
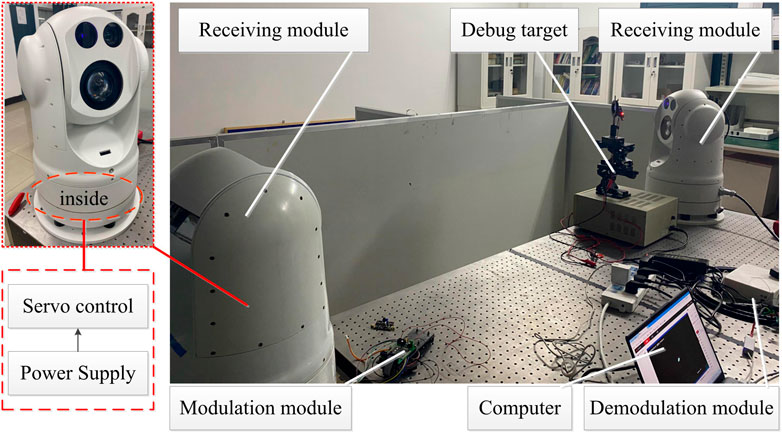
FIGURE 2 . Laser communication experimental system.
Before the experimental test, first use a test target to calibrate the position of the receiving module and the transmitting module, and then add attenuation pads to the communication link to complete the simulation of underwater communication attenuation. The emitting laser spot is collected by the receiving end after passing through the medium. The original spot signal is restored through demodulation processing. Processing system is used to calculate the energy loss of the light spot, thereby analyzing the communication efficiency of the system.
5.2 Servo control
Servo control modules are installed in the transmitting and receiving parts respectively, which are used to adjust the laser and detector by step scanning, thereby achieving effective alignment of communication angles. The servo control module is shown in Figure 3A , and its control response signal is shown in Figure 3B .
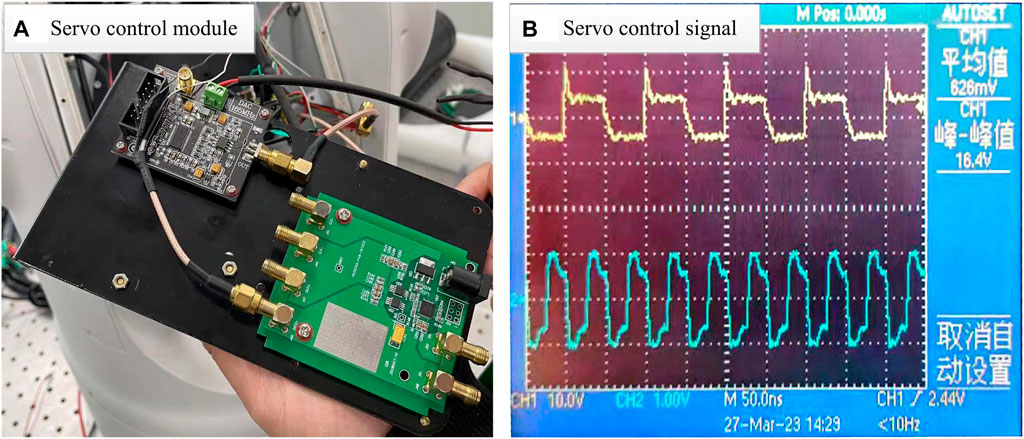
FIGURE 3 . Wavelength offset values at different positions. (A) Servo control module. (B) Servo control signal
The system drives the stepper motor through a servo control module to achieve adjustable control of the position and angle of the optical module, achieving alignment of the irradiation position and posture during communication. By Figure 3B , the maximum signal value is 0.75 V, and the minimum signal value is 0.14 V in Ch1 channel. This is a control command issued by the control module that completes stepping control through a square wave level signal. After adjustment by the servo module, the corresponding command signal is displayed on the Ch2 channel. The maximum value of the signal in Ch2 channel is 2.41 V, and the minimum value is 1.23 V. After amplifying the step signal, the amplitude has significantly increased, and the time interval has not changed. This control module can effectively control the scanning system through a stepper motor. In the servo system, a stepper motor is used to complete the scanning of the communication module, simulating the characteristics of underwater dynamic communication.
5.3 Receiving spot energy test
In underwater laser communication, the spot energy of the communication laser is an important indicator to judge the communication quality. The attenuation and turbulent disturbance of water on the laser spot can significantly affect the energy distribution characteristics of the laser spot. Therefore, it is necessary to measure the energy of laser spots with energy attenuation and turbulence disturbances. In the experiment, 50% attenuation plates were used to simulate the attenuation effect of seawater medium on laser light. The energy of the actual test laser spot obtained by the APD detector is shown in Figure 4A . The energy distribution is reconstructed using MATLAB software, as shown in Figure 4B .
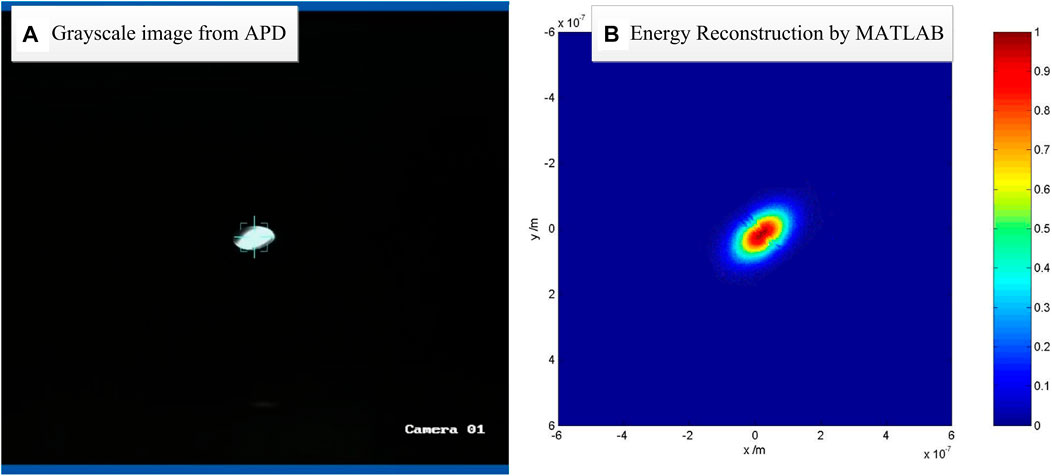
FIGURE 4 . Laser spot acquisition and energy distribution reconstruction. (A) Grayscale image from APD. (B) Energy Reconstruction by MATLAB
As shown in Figure 4A , over a communication distance of 10 m, when 1.0% attenuation chip was used, the laser spot still undergoes significant deformation. When 0.1% attenuation chip was used, the energy peak decreases significantly and the signal-to-noise ratio decreases. When 0.01% attenuation chip was used, the laser communication receiver cannot obtain a valid signal. The deformation of the laser spot tends to be elliptical, and the analysis shows that its deviation angle is caused by alignment deviation between the receiving module and the transmitting module. This situation will exist in actual laser communication. On this basis, the energy distribution of the light spot is simulated and reconstructed, and the reconstruction results are shown in Figure 4B . The energy map shows that the energy distribution of the light spot is elliptical, consistent with the actual test results. The intensity of energy meets the energy requirements of laser communication, which can achieve effective information transmission.
As the pass rate of the attenuator decreases, the input power decreases. The laser spot energy becomes weaker, and the packet loss of communication data gradually increases. Under the condition of using 1.0% attenuator, the test results show that the error rate of the system is better than 10 −6 . Under the condition of using 0.1% attenuator, the test results show that the error rate of the system is better than 10 −4 . It can be seen that when the energy attenuation exceeds an order of magnitude, the bit error rate significantly increases. With a 0.01% attenuator, the effective signal is submerged in noise.
6 Conclusion
An underwater laser communication system was proposed based on PPM modulation. The system is mainly composed of a transmitting module and a receiving module. The system applies PPM modulation and demodulation technology to the transmitting module and the receiving module. The control of step scanning in the servo module was tested and analyzed in the experiment, for verifying the stability of clock matching. In the spot energy analysis test, the laser spot intensity at different attenuation states was obtained. The system communication error rate under different laser spot energy intensities was tested and studied. The feasibility of this system has been verified, and it has certain application prospects in the field of underwater laser communication.
Data availability statement
The original contributions presented in the study are included in the article/supplementary material, further inquiries can be directed to the corresponding authors.
Author contributions
BD completed the paper. ST completed the system theoretical analysis. PZ completed the simulation calculation. JW completed the experimental test.
This work was supported in part by Natural Science Foundation of Jilin Province (YDZJ202301ZYTS394); Science and Technology Research Project of Jilin Provincial Department of Education (JJKH20230814KJ).
Conflict of interest
The authors declare that the research was conducted in the absence of any commercial or financial relationships that could be construed as a potential conflict of interest.
Publisher’s note
All claims expressed in this article are solely those of the authors and do not necessarily represent those of their affiliated organizations, or those of the publisher, the editors and the reviewers. Any product that may be evaluated in this article, or claim that may be made by its manufacturer, is not guaranteed or endorsed by the publisher.
1. Zhao Z, Bai Z, Jin D, Qi Y, Ding J, Yan B, et al. Narrow laser-linewidth measurement using short delay self-heterodyne interferometry. Opt Express (2022) 30(17):30600–10. doi:10.1364/OE.455028
PubMed Abstract | CrossRef Full Text | Google Scholar
2. Zhao Z, Bai Z, Jin D, Chen X, Qi Y, Ding J, et al. The influence of noise floor on the measurement of laser linewidth using short-delay-length self-heterodyne/homodyne techniques. Micromachines (2022) 13(8):1311. doi:10.3390/mi13081311
3. Xiang WD, Yang P, Wang S, Xu B, Liu H. Underwater image enhancement based on red channel weighted compensation and gamma correction model. Opto-Electronic Adv (2018) 1(10):18002401–9. doi:10.29026/oea.2018.180024
CrossRef Full Text | Google Scholar
4. Sui MH. The key technology research on underwater wireless optical communication systems . Qingdao: Ocean University of China (2009).
Google Scholar
5. Kim J, Joe H, Yu SC, Lee JS, Kim M. Time-delay controller design for position control of autonomous underwater vehicle under disturbances. IEEE Trans Ind Electro (2016) 63(2):1052–61. doi:10.1109/tie.2015.2477270
6. Zhang Y, Li X, Lv W, Chen J, Zheng M, Xu J, et al. Link structure of underwater wireless optical communication and progress on performance optimization. Opto-Electronic Eng (2020) 47(9):190734.
7. Duntley SQ. Light in the sea. J Opt Soc America (1963) 53(2):214–536. doi:10.1364/josa.53.000214
8. Shimura S, Ichimura SE. Selective transmission of light in the ocean waters and its relation to phytoplankton photosynthesis. J Oceanography (1973) 29(6):257–66. doi:10.1007/bf02108845
9. Tivey M, Fucile P, Sichel E. A low power, low cost, underwater optical communication system. Ridge (2000) 2(1):1–32.
10. Huang AP, Tao LW. Monte Carlo based channel characteristics for underwater optical wireless communications. Ieice Trans Commun (2017) 100(4):612–8. doi:10.1587/transcom.2016ebp3266
11. Gampagnaro F, Favaro F, Guerra F, Sanjuan V, Casari P Simulation of multimodal optical and acoustic communications in underwater networks. In: OCEANS 2015 ; May 18-21, 2015 ; Genoa, Italy. New York . IEEE (2015). p. 15473725.
12. Fu YQ, Huang CT, Du YZ. Effect of aperture averaging on mean bit error rate for UWOC system over moderate to strong oceanic turbulence. Opt Commun (2019) 451:6–12. doi:10.1016/j.optcom.2019.06.030
13. Han B, Wang W, Zheng Y, Shi K, Zhao W Influence of electro-optical crystal flatness on indirect modulation signal for underwater blue-green laser communication. Proc SPIE (2019) 11068:1106823.
14. Vali Z, Gholami A, Ghassemlooy Z, Michelson DG. System parameters effect on the turbulent underwater optical wireless communications link. Optik (2019) 198:163153. doi:10.1016/j.ijleo.2019.163153
15. Shen P, Yang L, Chen YS. Characteristics and development status of underwater laser communication technology. China Plant Eng (2018)(4) 214–5.
16. Wu T-C, Chi Y-C, Wang HY, Tsai CT, Lin GR. Blue laser diode enables underwater communication at 12.4 Gbps. Nature (2017) 25(13):40480–14765. doi:10.1038/srep40480
17. ValiGholami ZASGHAR, Ghassemlooy Z, Michelson DG, Omoomi M, Noori H. Modeling turbulence in underwater wireless optical communications based on Monte Carlo simulation. J Opt Soc America, A. Opt image Sci Vis (2021) 34(8):1187. doi:10.1364/josaa.34.001187
18. Chen H, Bai Z, Cai Y, Yang X, Ding J, Qi Y, et al. Order controllable enhanced stimulated Brillouin scattering utilizing cascaded diamond Raman conversion. Appl Phys Lett (2023) 122(9):092202. doi:10.1063/5.0137542
19. Jin D, Bai Z, Li M, Yang X, Wang Y, Mildren RP, et al. Modeling and characterization of high-power single frequency free-space Brillouin lasers. Opt Express (2023) 31(2):2942–55. doi:10.1364/oe.476759
Keywords: laser communication, underwater communication, servo control, dynamic testing, PPM
Citation: Dong B, Tong S, Zhang P and Wang J (2023) Research on underwater wireless dynamic optical communication system based on PPM modulation. Front. Phys. 11:1195052. doi: 10.3389/fphy.2023.1195052
Received: 28 March 2023; Accepted: 19 April 2023; Published: 05 May 2023.
Reviewed by:
Copyright © 2023 Dong, Tong, Zhang and Wang. This is an open-access article distributed under the terms of the Creative Commons Attribution License (CC BY). The use, distribution or reproduction in other forums is permitted, provided the original author(s) and the copyright owner(s) are credited and that the original publication in this journal is cited, in accordance with accepted academic practice. No use, distribution or reproduction is permitted which does not comply with these terms.
*Correspondence: Shoufeng Tong, [email protected] ; Peng Zhang, [email protected]
This article is part of the Research Topic
Advances in High-Power Lasers for Interdisciplinary Applications
Review Article: Underwater Voice Communication
Ieee account.
- Change Username/Password
- Update Address
Purchase Details
- Payment Options
- Order History
- View Purchased Documents
Profile Information
- Communications Preferences
- Profession and Education
- Technical Interests
- US & Canada: +1 800 678 4333
- Worldwide: +1 732 981 0060
- Contact & Support
- About IEEE Xplore
- Accessibility
- Terms of Use
- Nondiscrimination Policy
- Privacy & Opting Out of Cookies
A not-for-profit organization, IEEE is the world's largest technical professional organization dedicated to advancing technology for the benefit of humanity. © Copyright 2024 IEEE - All rights reserved. Use of this web site signifies your agreement to the terms and conditions.
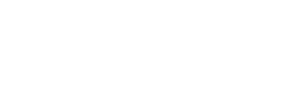
The Fifth IEEE OES Underwater Communications and Networking (UComms21) Conference
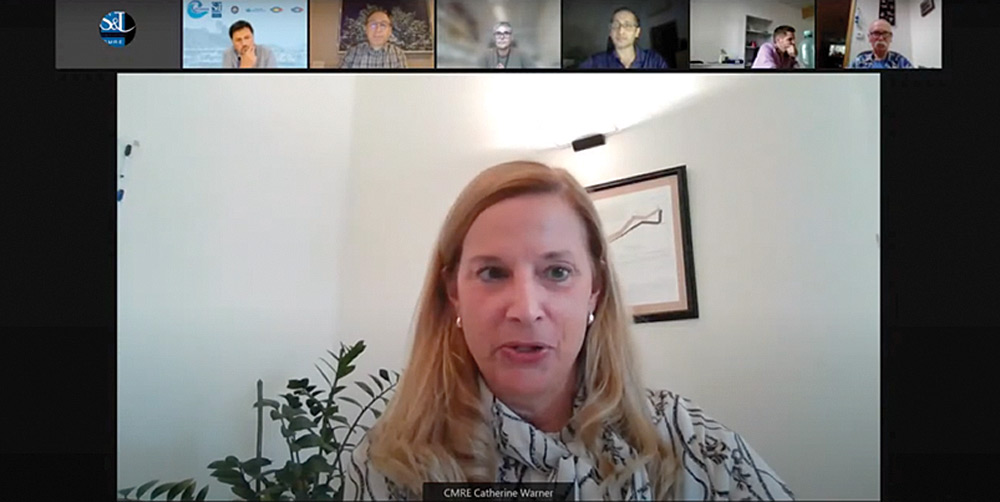
João Alves (UComms 2021 General Chair), OES AdCom member
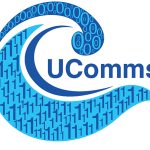
This highly regarded, academic conference has been held every other year since 2012 and was set to have its fifth edition in early September 2020.
With the new world context imposed by the COVID-19 pandemic, on May 2020, the UComms organising team issued a survey targeted at UComms’ authors and community of interest (including the technical committee members and UComms 2018 attendees). The intention of the survey was to collect the feedback of such interested parties with the ultimate goal of educating a decision for the 2020 edition of UComms.
The answers collected showed a clear preference for postponing the conference. This came with little surprise as UComms has always been highly regarded for its ability to remain specialised, attract the best researchers and promote face-to-face discussions that move the field forward.
Following such clear indication, and after close consultation with the conference sponsors, a decision was taken to postpone the conference until September 2021.
UComms Webinar 2020
In order to maintain community engagement, CMRE, the IEEE OES and the NATO Allied Command Transformation organised the first UComms Webinar series. The Webinar series, which was held between the 1 st and 4 th of December, 2020, consisted of four invited talks broadcast live though CMRE’s social media channels. The line-up of the talks was as follows:
Milica Stojanovic, Northeastern University (USA): “Underwater Acoustic Communications: Is Transmit Beamforming a Possibility?”
Ian Akyildiz, Technology Innovation Institute (Abu Dhabi): “ Next Decade Challenges for Underwater Wireless Communication Networks ”
Mandar Chitre, National University of Singapore (Singapore): “ Underwater Acoustics in the Age of Differentiable and Probabilistic Programming ”
João Alves, NATO STO CMRE (Italy): “ JANUS: Why It Matters and the Critical Path into the Future ”
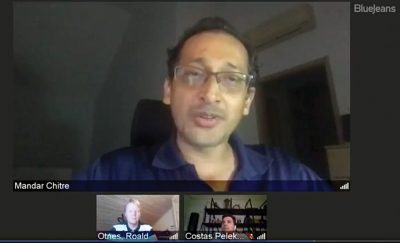
Thanks to the sponsoring organizations and the speakers who generously agreed to take part in this event, the webinar was free and open to all. This format proved to be very effective at engaging wide participation and promoting open discussion.
All materials from the webinar (abstracts, bios and the lectures) remain available through the UComms website ( www.ucomms.net ), navigating to “UComms Webinar 2020”.
UComms 2021
Given the prolonged effects of the pandemic and the restrictions still in place at the time, there was no possibility to organise an in-person conference in Italy in September 2021. The conference still had a commitment to the authors to publish their manuscripts (originally submitted for the conference in 2020) and for that reason it was decided to hold a remote conference on the dates of the planned in-person event: from August 31 st to September 2 nd .
Just like with all UComms editions, this conference was organised in a series of structured sessions that were coordinated by key researchers in the field, aiming at gathering top quality contributions that could lead to a vibrant exchange of knowledge and increase common understanding of the state-of-the-art. The multiple sessions were handled in a single track, giving the opportunity to all participants to follow all the talks and take part in all discussions.
The session organisers took the role of inviting papers, managed the reviews, ranked and selected the final presentations in coordination with the general chairs and conducted their session at the conference.
For UComms 2021 a total of eight sessions were set up:
- “Machine Learning for Adaptive Underwater Communications” (this session was eventually dropped and papers absorbed in other sessions);
- “The Roles of Deterministic and Statistical Methods in Acoustic Propagation Modelling for Underwater Acoustic Communications Simulation and Performance Prediction”;
- “Networking, Localisation and Scheduling”;
- “Next-generation Adaptive Modem Architectures and Cognitive Networking Strategies”;
- “Interoperability and Standards”;
- “Non-Acoustic Underwater Communications”;
- “New Applications Enabled by Next-generation Underwater Acoustic Comms”;
- “Channel-aware Security and Protocol Design.”
A total of 30 short papers (strictly four pages plus references) were accepted for presentation and publication after the usual peer review process of UComms. The program was then arranged to fit a daily time window of approximately four hours to accommodate as much as possible the different time zones taking part in the remote presence conference (14:00-18:00 CEST).
The conference was open by CMRE’s director, Dr. Catherine Warner, and proceeded with live presentations of all accepted manuscripts with Q&A sessions moderated by the respective session organisers.
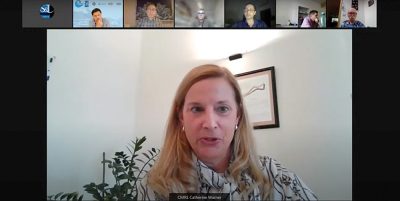
Thanks to the sponsorship structure of the conference and the reduced organizational costs when compared to in-person editions, CMRE was able to conduct UComms free-of-charge for participants and authors, contributing to a significant knowledge sharing opportunity for those interested in the field. A total of 195 delegates registered from around the globe to join the UComms technical presentations.
The book of abstracts of the conference is available in the UComms website ( https://www.ucomms.net/docs/UComms21_BookOfAbstracts.pdf ) and the video presentations are available in CMRE’s YouTube channel (www.youtube.com/user/PAOCMRE) for the scientific community for a-posteriori viewing.
A Special edition of the JOE will be setup with a selection of articles to be expanded and published in full article form.
Future UComms Conferences
UComms is now a well-established international biennial conference series, bringing together participants from around the globe to share their latest developments in the ever-evolving topic of underwater communications. UComms success was achieved through the recurring participation of the top scholars in the field, the strict peer review process, the focused topic, and the opportunities created for networking and discussions.
The current plan is to hold the next edition of UComms in 2022, returning to a full in-presence format for what will be its 10 th anniversary. The call for papers is expected to be issued before the end of 2021.
Written by Matthew Gelis
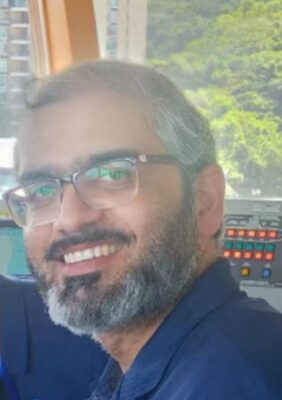
Dr. Potter has 45 years’ experience in marine and glaciological research, holding academic University positions (NUS, NTNU), research institute roles (BAS, SIO, NUS, NTNU) and NATO research and senior management positions (Saclant ASW Centre, NURC, CMRE) across Europe, the US and Asia. He is now a Full Professor at NTNU in Norway. His scientific specialisations are glaciology, polar oceanography, acoustics, ambient noise, marine mammals, autonomous vehicles, machine intelligence, communications, distributed sensing networks, ocean advocacy and environmental conservation.
Frustrated by two decades of failure to promote ecosystem and climate awareness, in 2004 he took a 14-month ‘seabbatical’ with his family to circumnavigate the Indian Ocean by sailboat on a sponsored voyage of research, public outreach and education with a focus on the increasing degradation of the marine environment and mankind’s failed stewardship of this unique and beautiful blue planet.
Dr. Potter has 10 years’ experience facilitating, coaching & training personal performance & leadership skills, change-management, team building, relationship management & corporate strategy. Dr. Potter co-founded the Tropical Marine Science Institute and Founded the Acoustic Research Laboratory in the National University of Singapore, leading it from inception, through growth, to a sustainable internationally-recognized centre of excellence.
Dr. Potter has ~170 peer-reviewed publications with ~3400 citations, h-index = 26, i10-index = 66. He is a Fellow of the IEEE and MTS, International Fellow of the Explorers Club, Associate Editor for the IEEE Journal of Oceanic Engineering, Lifetime Member Marine Mammal Society, PADI Master Scuba Diver Trainer, TDI Nitrox and ECC Rebreather Diver and sometime General Aviation Pilot (Gliders and Gyrocopters).
It is no longer true that he neither owns nor operates a television.
Here is how to get your paper copies of the OES Beacon in the future.
1. OES members need to contact the IEEE Contact Center at 1-800-678-4333 or 1-732-981-0060- Monday thru Friday- 8:00 AM- 4:30 PM EST.
2. Or . . . send the IEEE Contact Center an email at [email protected] with your name, IEEE member number and your request to receive your paper copy of the OES Beacon.
A new initiative has been added to the mission of the IEEE Oceanic Engineering Standards Committee. It recognizes that the systems engineering and oceanic engineering missions are in symbiosis with each other and that there will be close cooperation now between the IEEE Oceanic Engineering Society Standards Committee and the IEEE Systems Council Standards Committee. More details appear at: https://ieeeoes.org/technical-activities/standards-activities/ “

DISTINGUISHED LECTURER TOPICS: Synthetic Aperture Radar for oil spill observation Marine oil pollution monitoring is a topic of great applicative and scientific relevance. Use of remotely sensed measurements is of special interest and, in particular, the SAR because of its almost all-weather and all-day imaging capability at fine spatial resolution is the most effective tool. Conventional single-polarization SAR oil spill monitoring techniques are limited in their capability to detect oil slicks since they strongly rely on suitable thresholds, training samples, and ancillary information. Hence, an expert image analyst is due. The launch of a number of polarimetric SAR missions, along with the understanding of the peculiar physical mechanisms governing the scattering by an oil slick, led to a new paradigm (known as physical processing) that fostered a set of polarimetric algorithms particularly robust and efficient. Hence, suitable polarimetric models that exploit the departure from the slick-free sea Bragg scattering have been developed to effectively address oil slick monitoring. A set of polarimetric features extracted following such electromagnetic models have been proved to be reliable for oil slick monitoring. Polarimetric SAR observations led to a significant improvement in sea oil slick observation since they allow distinguishing oil slicks from a broad class of lookalikes in an unsupervised way.
Wind speed estimation by Synthetic Aperture Radar The oceans cover over 70% of the Earth’s surface, carrying out about 50% of global primary production and hosting the widest biodiversity on the planet. Ocean monitoring plays a key role in all World Meteorological Organization (WMO) programs. Within such a framework, sea-surface wind field is attracting growing attention from engineers and in order to boost the sustainable development by exploiting new “clean” energy sources (e.g., to plan and implement offshore wind energy farms). In this seminar the SAR, a microwave narrowband coherent imaging system, is analyzed as sensor for sea surface wind estimation. The critical analysis of three general procedures is presented along with a physical background.
Man-made targets at sea observation by polarimetric SAR Sea man-made targets usually appear as bright spots over a dark background, this is due to some concurring physical factor: the large size of the target compared to the SAR spatial resolution, the metallic nature of the target that ensures a strong electromagnetic return and a low return of the sea surface that occurs in low-to-moderate wind regimes. When some of the aforementioned physical hypothesis do not occur the detection of man-made targets becomes a much more challenging task. In the seminar a physical-driven approach is presented along with a number of examples. In this seminar the supporting role of SAR polarimetry and physical processing for man-made target at sea detection is illustrated.
Tamaki Ura is Professor Emeritus of the University of Tokyo, where he is a world leader in the development of Autonomous Underwater Vehicles.
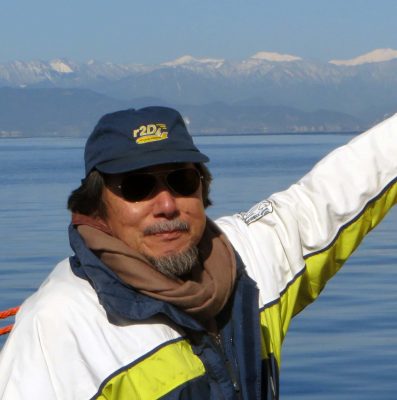
Professor Ura has dedicated himself to the activities of international societies by establishing IEEE/OES Japan Chapter, where he served as its first chair from 1995 to 2000. He organized the International Symposium on Underwater Technology: UT’98, UT2000, UT’02, UT’07, UT’11, UT’13 in Tokyo and UT’04, UT’19 in Taipei, UT’09 in Wuxi, UT’17 in Busan and UT’15 in Chennai under the IEEE/OES Japan Chapter, and realized the international symposium on OCEANS/Techno-Ocean 2004, Kobe in November 2004. This was the first OCEANS conference held in Asia.
He has contributed on ocean related themes not only for the academic audiences but also for the public. He worked as a Cabinet Councillor for The Headquarters of Ocean Policy of Japanese Government from 2007 to 2018. He was a Commissioned Judge of the High Marine Accidents Inquiry Agency from 1984 to 2008, and he was the chairman of the Ocean Technology Committee of the Society of Naval Architects of Japan from 1998 to 2000.
After retiring from the University of Tokyo, he has been engaged in R&D of field robots such as beach cleaning robots and tomato collection robots. In addition, he organized a team which investigated the sunken ship, and discovered 27 submarines such as I-47, I-58 of Imperial Japanese Navy and U-boat U-511(Ro-500), and the passenger ship “Taiyo Maru”.
Based on these activities, he has received many awards;
2019: Distinguished service award (Robotics and Mechatronics Division) from the Japan Society of Mechanical Engineers (Japan)
2016: The Fujisankei Communications Group Award of the 25 th Grand Prize for the Global Environment Awards from Fujisankei Communications Group (Japan)
2013: Technical Achievement award (Robotics and Mechatronics Division) from the Japan Society of Mechanical Engineers (Japan)
2012: AUV “TUNA SAND” was awarded the 5th Robot Award from METI (Japan)
2010: IEEE Oceanic Engineering Society Distinguished Technical Achievement Award
2007: Nominated as IEEE Fellow, for contributions to autonomous underwater vehicle technologies.
2006: Distinguished Service Award from IEEE/OES Japan Chapter (Japan)
2000: Award from Agency for Science and Technology (Japan)
1999: Award from the Japan Society of Mechanical Engineers (Japan)
1998: Award from High Automation Technology Association (Japan)
1995 and 1997: Awards on Invention from the Society of Naval Architects of Japan (Japan)
1982: Houkou Award on the significant contributions to safety of moored ship (Japan)
1979: Award from the Society of Naval Architects of Japan (Japan)
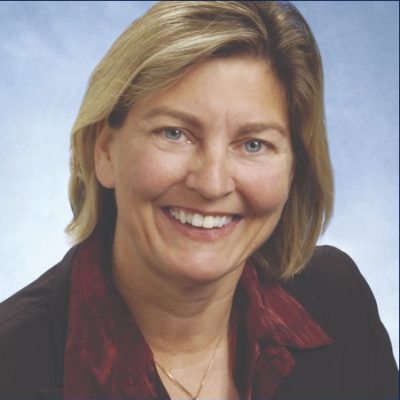
Her raft of Honors and Awards validate her reputation as a leader in her profession with a clear ability to develop innovation seeding projects and new maritime business. She has been invited to speak to a wide range of audiences from school groups to defense think-tanks, on topics from undersea optics technologies to over-views of ocean engineering.
She has over 75 publications which follow a development of her interests from technology topics earlier to more recent papers on the state of technology and the future demands in subsea optics.
Honors and Professional Engagements:
Harris Technical Fellow, 2020
HARRIS Building a Legacy Award for demonstrating pride and accountability by developing next generation of talent through leadership and mentoring (2018)
Elected President of the Marine Technology Society (MTS), 2017-2018
Invited USA Science & Engineering Nifty Fifty Speaker, 2015-2016
SWE Space Coast Outstanding Woman Engineer Award, 2012
Appointed to FL Tech OE & UCF EECS Industry Advisory Boards, 2011
Delegated Senior Member of IEEE Oceanic Engineering Society, 2010
HARRIS Golden Quill (2009, 2016) and Industry Recognition (2012) Awards
Founded/Appointed Chair of the MTS Ocean Observing Systems Committee (2008 – 2015); & Chair of Underwater Imaging Committee (2004-2008)
Appointed to MTS Journal Editorial Board (2008 – Present); MTSJ Guest Editor of 10 special issues including 4 State of Technology volumes (2008 – 2019)
Member of NDIA, MTS, IEEE/OES, SWE and Upsilon Phi Epsilon Honor Society
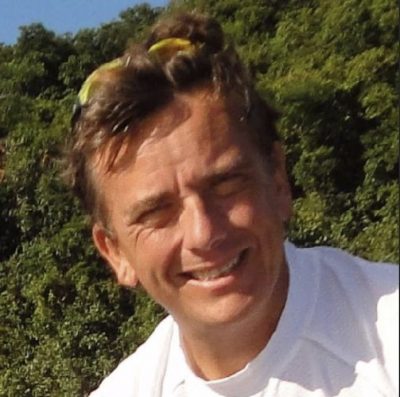
John is a ‘big-picture’ visionary academic professional with experience encompassing strategic business development and award-winning research, including a National Defence Technology Prize in 2006 and the NATO Scientific Achievement Award in 2018. He has a proven track record of establishing research facilities that exemplify standards of excellence, having founded the Acoustic Research Laboratory and co-founded the Tropical Marine Science Institute in Singapore, and is a recognised educational leader with coaching, facilitating and training experience. He is an effective verbal/written communicator, experienced in managing change, building new opportunities, advocating for universities/organisations and interfacing with multi-national governmental, academic, military, and industrial organisations, as demonstrated by his pioneering leadership to establish the first digital underwater communications standard, ‘JANUS’. He is extensively internationally published, with over 2,500 citations. John has also sailed with his family across the Atlantic, Pacific and Indian Oceans, clocking 50,000+ nautical miles of blue-water cruising over a period of 30 years in pursuit of environmental awareness and marine conservation.
Among his achievements are:
- Pioneering work on the impact of climate change on the Antarctic, for which he was awarded the Polar Medal by H.M. Queen Elizabeth II, with his publications from the 80’s still being cited today.
- Leading the project that developed the first Ambient Noise Imaging system (ADONIS), producing passive acoustic video images of silent objects in real time, for which he received an ASA award for best paper.
- Expedition leader for a 10,000 n.m. circumnavigation of the Indian Ocean by sailboat in support of education, public outreach and marine research, leading to publication of the first measurements of persistent organic pollutants in the Indian Ocean in 30 years.
- Leading the project that built the first 2-D digital Ambient Noise Imaging array (ROMANIS), resulting in a National Defence Technology Prize.
- Leading the team that developed the first digital underwater communications standard ‘JANUS’, now adopted by 28 nations and which resulted in the NATO Scientific Achievement Award
- Conceived and wrote the draft Letter of Intent, now adopted as a formal memorandum, signed in October 2018 by Defence Ministers of 13 NATO nations, to collaborate on Maritime Unmanned Systems.
- Founder of the Acoustic Research Laboratory (ARL) in Singapore
- Educational leader and award-winning instructor/trainer with a history of improving instruction methods including use of new technologies and nurturing innovation through experiential learning.
The following selected publications illustrate his diverse experience:
- Gordon, J., Gillespie, D., Potter, J.R., Frantzis, A, Simmonds, M.P., Swift, R., et. al. (2003) A review of the effects of seismic surveys on marine mammals . Marine Technology Society Journal, 37, (4), 16-34. (332 citations)
- Chitre, M., Potter, J.R., Ong, S.H., (2006) Underwater Acoustic Signal Processing-Optimal and Near-Optimal Signal Detection in Snapping Shrimp Dominated Ambient Noise. IEEE Journal of Oceanic Engineering 31 (2), 497-503. (161 citations)
- Potter, J.R., Paren, J.G. (1985) Interaction between ice shelf and ocean in George VI Sound, Oceanology of the Antarctic Continental Shelf 43, 35-58. (108 citations)
- Potter, J.R., Mellinger, D.K., Clark, C.W. (1994) Marine mammal call discrimination using artificial neural networks. The Journal of the Acoustical Society of America 96 (3) , 1255-1262. (95 citations)
- Petrioli, C., Petroccia, R., Potter, J.R., Spaccini, D. (2015) The SUNSET framework for simulation, emulation and at-sea testing of underwater wireless sensor networks. Ad Hoc Networks, 34, 224-238. (82 citations)
- Potter, J.R., Alves, J., Green, D., Zappa, G., Nissen, I., McCoy K. (2014) The JANUS underwater communications standard. Underwater Communications and Networking (80 citations)
- Epifanio, C.L., Potter, J.R., Deane, G.B., Readhead, M.L., Buckingham, M.J. (1999) Imaging in the ocean with ambient noise: the ORB experiments. The Journal of the Acoustical Society of America 106 (6) , 3211-3225. (66 citations)
- Petrioli, C., Petroccia, R., Potter, J.R. (2011) Performance evaluation of underwater mac protocols: From simulation to at-sea testing. IEE/MTS OCEANS 2011 Spain , 1-10. (56 citations)
- Wurl, O., Potter, J.R., Obbard, J.P., Durville, C. (2006) Persistent organic pollutants in the equitorial atmosphere over the open Indian Ocean, Environmental Science and Technology , 40(5) 1454-1461 (53 citations)
- Potter, J.R. (1994) Acoustic imaging using ambient noise: Some theory and simulation results. Journal of the Acoustical Society of America 95(1) 21-33 . (47 citations)
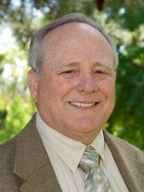
Relatively recent publications have been on the subjects of range compensation for backscattering measurements in the difference-frequency nearfield of a parametric sonar; discriminating between the nearfield and the farfield of acoustic transducers; standard-target calibration of active sonars used to measure scattering. Some other recent, collaborative publications have concerned acousto-optic effect compensation for optical determination of the normal velocity distribution associated with acoustic transducer radiation, and performance prediction of a dual-baseline radar or sonar interferometer based on a Vernier critical value concept.
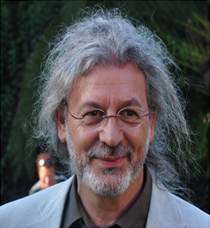
René Garello was born in 1953. He received the Ph.D. degree in Signal Processing at the Institut National Polytechnique de Grenoble (INPG) in 1981. From 1982 to 1984 he worked as a Research Associate at Aeronomy Lab, National Oceanic and Atmospheric Administration (NOAA) at Boulder, Colorado (USA). He joined the Ecole Nationale Supérieure des Télécommunications de Bretagne (ENST Bretagne), Brest, France in 1985. In 1988 he became Professor in this engineering school in the field of signal processing and image processing and in 1995, Prof. Garello obtained his Habilitation (HDR; Habilitation to Supervise Research).
Prof. Garello was an elected OES AdCom member from 1999 to 2001, from 2003 to 2005 and in 2005 for a new three year term. In the beginning of 2001, he headed the Committee on Conference Policies (CoCoPo) which was in charge of defining a new set of Conference Policies and Procedures in order to insure continuity between the successive OCEANS conferences. This committee jointly held with MTS members defined several new approaches and came up with the concept of two Oceans-a-year (every year in Northern America, every other odd year in Europe and every other even year in Asia-Pacific). In order to implement this plan a new committee was formed: the Joint Oceans Advisory Board or JOAB, of which Prof. Garello is the co-chair.
Prof. Garello was the General Chairman of the first OCEANS of the new Two-Oceans-a-year concept: OCEANS’05 held in Brest, France in June 2005. In 2005, he was elected Vice-President Conference Operations and then re-elected in 2006 and 2008. He was elevated to the grade of Fellow of the IEEE, class of 2006, “for contributions to signal processing applied to remote sensing of the ocean”. He received the OES Service Awards in 2006 for developing and implementing the two OCEANS conference policy.
Prof. Garello has authored or co-authored more than 40 papers, a hundred and thirty conference communications and three books.
Prof. Garello is also an active member of the IEEE/GRS (Geoscience and Remote Sensing) Society. His main research interests lie in Remote Sensing, 2D signal processing, statistical and spectral analysis applied to ocean surface features detection and characterization. Prof. Garello has been active in GEO and participated in the 2005 Plenary, organized a GEOSS workshop in Corte in 2006 in addition to his participation with the IEEE Committee on Earth Observation.
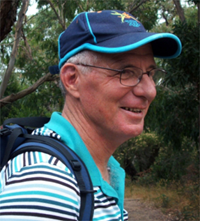
As a Distinguished Lecturer of the IEEE Ocean Engineering Society, Milica is happy to give lectures at varying levels of technical detail in the general area of underwater acoustic communications and networking. The lectures can be tailored to diverse audiences, ranging from general outreach to high-level graduate students and research practitioners with background in digital communications, signal processing or ocean acoustics. Specific lecture topics include the following:
1) Signal processing for acoustic communications (overview of existing systems; methods for synchronization, channel equalization, and diversity combining; experimental results that demonstrate some of the highest bit-rates achieved to-date)
2) Multi-carrier modulation/detection for acoustic channels (overview of the basic concepts of multi-carrier signaling; dealing with the Doppler distortion through dedicated signal processing methods; experimental demonstrations)
3) Statistical characterization of underwater acoustic communication channels (a discussion of general properties of acoustic communication channels and fundamental differences from terrestrial radio channels, followed by the description of recently developed statistical models and their experimental verification; implications for signal processing)
4) Multiple access in underwater acoustic systems (accommodating multiple users transmitting at the same time and in the same frequency band; cellular architectures for acoustic systems)
5) Reliable transmission over acoustic channels (coding for improved link reliability; using the feedback link for efficient power and rate control; automatic repeat request methods for achieving full reliability; multicast and broadcast systems)
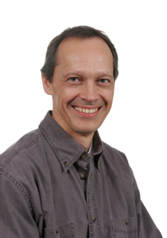
He joined the Defence Research Establishment Atlantic (now Defence R&D Canada – Atlantic), Dartmouth, Canada where he researched towed array self-noise. From 1985-1988, he attended the University of Bath, UK where he received his PhD in Physics. His research on acoustic scattering from ocean boundaries earned him the Chesterman Medal from the University for “Outstanding Research in Physics”. Upon returning to DRDC Atlantic in 1989, he joined the Acoustic Countermeasures group to work on acoustic scattering and time spreading. From 1996 until 2003 he led several research groups that focused on experimentation and modeling to support sonar research. Since 2003 he has managed projects in Rapid Environmental Acoustics and Aural Classification for underwater acoustics and is currently Principal Scientist in the Underwater Sensing section at DRDC.
Dr. Hines is a Fellow of the Acoustical Society of America, a member of IEEE, and an Adjunct Professor at Dalhousie University’s Dept. of Graduate Studies. He is a seasoned experimentalist and has been chief scientist for several collaborative international research trials. His present research interests include acoustic scattering, sound speed dispersion in the seabed, vector sensor processing, and the application of aural perception in humans, to target classification in sonar.
Lecture topics:
1. The application of aural perception in humans to active and passive sonar classification.
Humans have a remarkable ability to aurally classify transient acoustic signals – from a dog’s bark to the slamming of a car door. Sonar experts have always relied to some extent on this ability to aurally classify sounds to assist in identification – this includes active sonars, in which an acoustic wave is transmitted and one listens to the received echo from a target, such as a submarine, and passive sonars in which one simply listens for signals of interest, such as a marine mammal call. This begs the questions, “How do humans discriminate between these sounds and can we develop a computer algorithm to do it?” In this presentation, we examine those questions, and present some rather pleasing results from an automatic aural classifier that was applied to active sonar target classification and to passive classification of marine mammal vocalizations.
2. Vector sensors: Over 40 and Still Hot (a Hot Topics lecture given at the 151st Meeting of the Acoustical Society of America) Acoustic vector sensors and gradient arrays have been in use in underwater acoustics for more than 4 decades so one may wonder why they might be considered a “Hot Topic”. The reason lies in the recent resurgence in their use. This in turn, is due primarily to major advances in engineering and signal processing that have been applied to these devices. Historically, theoretical gains have been difficult to achieve with these sensors due to their susceptibility to uncorrelated noise. That is to say, the very process of making a localized measurement of the vector acoustic field lowers the signal-to-noise ratio, relative to a simple pressure measurement. However, with today’s advances in design, manufacturing, and digital signal processing, high-quality performance can be achieved in a very small package size. Moreover, the current interest in these devices isn’t limited to underwater acoustic applications; rather it extends across a number of technical areas within the acoustics community. This presentation will begin with a gentle introduction to the theoretical foundation and history of these devices. Then some current applications in both underwater and airborne acoustics will be highlighted.
Stefan Williams graduated BASc from The University of Waterloo (1998) in Systems Design Engineering and Ph.D (2002) in Field Robotics from The University of Sydney. His research interests are in the areas of autonomous navigation, high-resolution multispectral stereo photographic imaging and swath mapping to capture 3-D images of the sea floor, AUV planning and machine learning and classification of marine imagery. He works in collaboration with organizations including the Australian Institute for Marine Sciences, CSIRO, the University of Tasmania, TAFI, SARDI and others undertaking AUV surveys of marine habitats around Australia and was appointed head of Australia’s Integrated Marine Observing System (IMOS) Autonomous Underwater Vehicle (AUV) Facility. Collaborates extensively with colleagues at the Woods Hole Oceanographic Institute, MIT, the University of Tokyo, the University of Southampton, the University of Rhode Island, the University of Michigan, St. Andrews University and the University of Hawaii exploring the use of AUV systems in support of studies in marine ecology, archaeology and geoscience.
Rick Spinrad received a BA in Earth & Planetary Sciences from The Johns Hopkins University (1975), and M.S (1978) and Ph.D (1982) in Oceanography from Oregn State University. He is an accomplished researcher in marine environmental studies; demonstrated leadership in design of sophisticated environmental instrumentation; innovator of National Ocean Sciences Bowl, a program for high school students; Successful program manager with significant annual growth in programs of responsibility at both Navy and NOAA; Globally sought public speaker with over 100 speeches and media appearances (print and broadcast including The New York Times, MSNBC, The Washington Post, Huffington Post, USA Today, Discovery Channel, The Weather Channel, BBC); prepared and delivered testimony to Congress and state legislature to increase budgets and improve programs in research and education; established record of program and policy development in economic development (including engagement with venture capital and angel investors)
underwater wireless communication Recently Published Documents
Total documents.
- Latest Documents
- Most Cited Documents
- Contributed Authors
- Related Sources
- Related Keywords
An efficient IDMA-OFDM underwater wireless communication with Reed Solomon and Hamming code
Led-to-led wireless communication between divers.
<p class="Abstract">For military divers, having a robust, secure, and undetectable wireless communication system available is a fundamental element. Wireless intercoms using acoustic waves are currently used. These systems, even if reliable, have the defect of being easily identifiable and detectable. Visible light can pass through sea water. Therefore, light can be used to develop short-range wireless communication systems. To realize secure close-range underwater wireless communication, the Underwater Optical Wireless Communication (UOWC) can be a valid alternative to acoustic wireless communication. UOWC is not a new idea, but the problem of the presence of sunlight and the possibility of using near-ultraviolet radiation (near-UV) has not been adequately addressed in the literature yet. In military applications, the possibility of using invisible optical radiation can be of great interest. In this paper, a feasibility study is carried out to demonstrate that UOWC can be performed using near-ultraviolet radiation. The proposed system can be useful for wireless voice communications between military divers as well as amateur divers.</p>
A Shadow Capture Deep Neural Network for Underwater Forward-Looking Sonar Image Detection
Image sonar is a widely used wireless communication technology for detecting underwater objects, but the detection process often leads to increased difficulty in object identification due to the lack of equipment resolution. In view of the remarkable results achieved by artificial intelligence techniques in the field of underwater wireless communication research, we propose an object detection method based on convolutional neural network (CNN) and shadow information capture to improve the object recognition and localization effect of underwater sonar images by making full use of the shadow information of the object. We design a Shadow Capture Module (SCM) that can capture the shadow information in the feature map and utilize them. SCM is compatible with CNN models that have a small increase in parameters and a certain degree of portability, and it can effectively alleviate the recognition difficulties caused by the lack of device resolution through referencing shadow features. Through extensive experiments on the underwater sonar data set provided by Pengcheng Lab, the proposed method can effectively improve the feature representation of the CNN model and enhance the difference between class and class features. Under the main evaluation standard of PASCAL VOC 2012, the proposed method improved from an average accuracy (mAP) of 69.61% to 75.73% at an IOU threshold of 0.7, which exceeds many existing conventional deep learning models, while the lightweight design of our proposed module is more helpful for the implementation of artificial intelligence technology in the field of underwater wireless communication.
Design and Analysis of Li-fi Underwater Wireless Communication System
Underwater telemetry sensory mechanisms.
There are various different methods and apparatuses in relation to detecting harmful toxins within our ocean and other aquatic environments. The need is for the best approach given the time sensitivity of the matter. Through looking at my past designs, and other buoy deployments, there may be a way to improve detection in regards to signal strength capability. This requires a thorough understanding and overview of software defined networking capabilities centered under underwater wireless communication and telemetry, as well as methods of improvement in regards to real time detection. There also needs to be an emphasis on the real time detection of data and its importance. The likelihood of a noticeable improvement is centered on the idea of a P2P wireless and/or mesh networking implementation to improve signal latency and strength. There shall also be an emphasis on experimental methods as well and protocols of improvement or further implementation. Due to the doppler effect on waves, and the difficulty for traditional signal processing and strength to happen underwater, research within the field of underwater acoustics and wireless communication is crucial.
Performance analysis of patch antenna for underwater wireless communication in seawater
<span lang="EN-US">Underwater wireless communication in seawater is becoming more interesting and challenging in recent years. The development of antenna for underwater wireless communication in seawater at 900 MHz UHF range frequency is implemented by using patch antenna. In this paper, the antennas were designed using FEKO, an electromagnetic simulation software, and a suitable size for rectangular patch antenna for seawater application was developed to study the relevance between λ0 with W and L in seawater. The difference between the patches in free space and seawater was the L size, which was slightly bigger, about 0.9 mm, than free space size. But the gain for patch antenna in seawater was found at -2.51 dBi, lower than patch antenna in free space, which was 5.76 dBi due to the path loss in seawater. This shows that attenuation happened, and a better antenna will be design. The one that has better gain, which is around above 2 dBi in seawater, in order to get better performance antenna in seawater environment.</span>
Full Duplex Physical and MAC Layer-Based Underwater Wireless Communication Systems and Protocols: Opportunities, Challenges, and Future Directions
Underwater wireless communication has gained a great deal of attention in the last couple of decades because of its applications in the military, industrial, and monitoring sectors. Despite the extreme physical and MAC layer difficulties, acoustics are used for various applications among the various modes of underwater communication technologies used. While significant research efforts have been made to address these issues, the bottleneck remains in achieving high bandwidth, high throughputs, and data rate. Researchers have begun to look into full duplex (FD) implementation to improve bandwidth efficiency and increase data rate and throughput. Users can send and receive data simultaneously over the FD links, maximizing bandwidth utilization and increasing throughput. As a result, we thoroughly reviewed various FD physical layered UWAC systems and MAC layered protocols for underwater communication. The various problems that the aforementioned systems and protocols have faced, as well as the solutions suggested in previous works to solve each problem, are also highlighted. Various metrics are used to compare the performance of various physical layered FD systems and FD MAC protocols. We also explore some of the open research questions in these FD-physical layered and MAC layered protocols, as well as future research directions. Based on ample information, we suggest a cross-layered architecture based on various IBFD-SI cancellations, DA-CSMA, and FD-MAC protocols. This review provides a broad view of the current FD physical and MAC layered protocols based on acoustic communication, as well as recommendations.
An Automated Underwater Wireless Communication System Using Li-Fi with IOT Support and GPS Positioning
Iot-based underwater wireless communication, routing strategies for underwater wireless communication: a taxonomy, export citation format, share document.
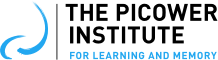
Paper: To understand cognition—and its dysfunction—neuroscientists must learn its rhythms
Thought emerges and is controlled in the brain via the rhythmically and spatially coordinated activity of millions of neurons, scientists argue in a new article. Understanding cognition and its disorders requires studying it at that level.
It could be very informative to observe the pixels on your phone under a microscope, but not if your goal is to understand what a whole video on the screen shows. Cognition is much the same kind of emergent property in the brain . It can only be understood by observing how millions of cells act in coordination, argues a trio of MIT neuroscientists. In a new article , they lay out a framework for understanding how thought arises from the coordination of neural activity driven by oscillating electric fields—also known as brain “waves” or “rhythms.”
Historically dismissed solely as byproducts of neural activity, brain rhythms are actually critical for organizing it, write Picower Professor Earl Miller and research scientists Scott Brincat and Jefferson Roy in Current Opinion in Behavioral Science . And while neuroscientists have gained tremendous knowledge from studying how individual brain cells connect and how and when they emit “spikes” to send impulses through specific circuits, there is also a need to appreciate and apply new concepts at the brain rhythm scale, which can span individual, or even multiple, brain regions.
“Spiking and anatomy are important but there is more going on in the brain above and beyond that,” said senior author Miller, a faculty member in The Picower Institute for Learning and Memory and the Department of Brain and Cognitive Sciences at MIT. “There’s a whole lot of functionality taking place at a higher level, especially cognition.”
The stakes of studying the brain at that scale, the authors write, might not only include understanding healthy higher-level function but also how those functions become disrupted in disease.
“Many neurological and psychiatric disorders, such as schizophrenia, epilepsy and Parkinson’s involve disruption of emergent properties like neural synchrony,” they write. “We anticipate that understanding how to interpret and interface with these emergent properties will be critical for developing effective treatments as well as understanding cognition.”
The emergence of thoughts
The bridge between the scale of individual neurons and the broader-scale coordination of many cells is founded on electric fields, the researchers write. Via a phenomenon called “ephaptic coupling,” the electrical field generated by the activity of a neuron can influence the voltage of neighboring neurons, creating an alignment among them. In this way, electric fields both reflect neural activity but also influence it. In a paper in 2022 , Miller and colleagues showed via experiments and computational modeling that the information encoded in the electric fields generated by ensembles of neurons can be read out more reliably than the information encoded by the spikes of individual cells. In 2023 Miller’s lab provided evidence that rhythmic electrical fields may coordinate memories between regions.
At this larger scale, in which rhythmic electric fields carry information between brain regions, Miller’s lab has published numerous studies showing that lower-frequency rhythms in the so-called “beta” band originate in deeper layers of the brain’s cortex and appear to regulate the power of faster-frequency “gamma” rhythms in more superficial layers. By recording neural activity in the brains of animals engaged in working memory games the lab has shown that beta rhythms carry “top down” signals to control when and where gamma rhythms can encode sensory information, such as the images that the animals need to remember in the game.
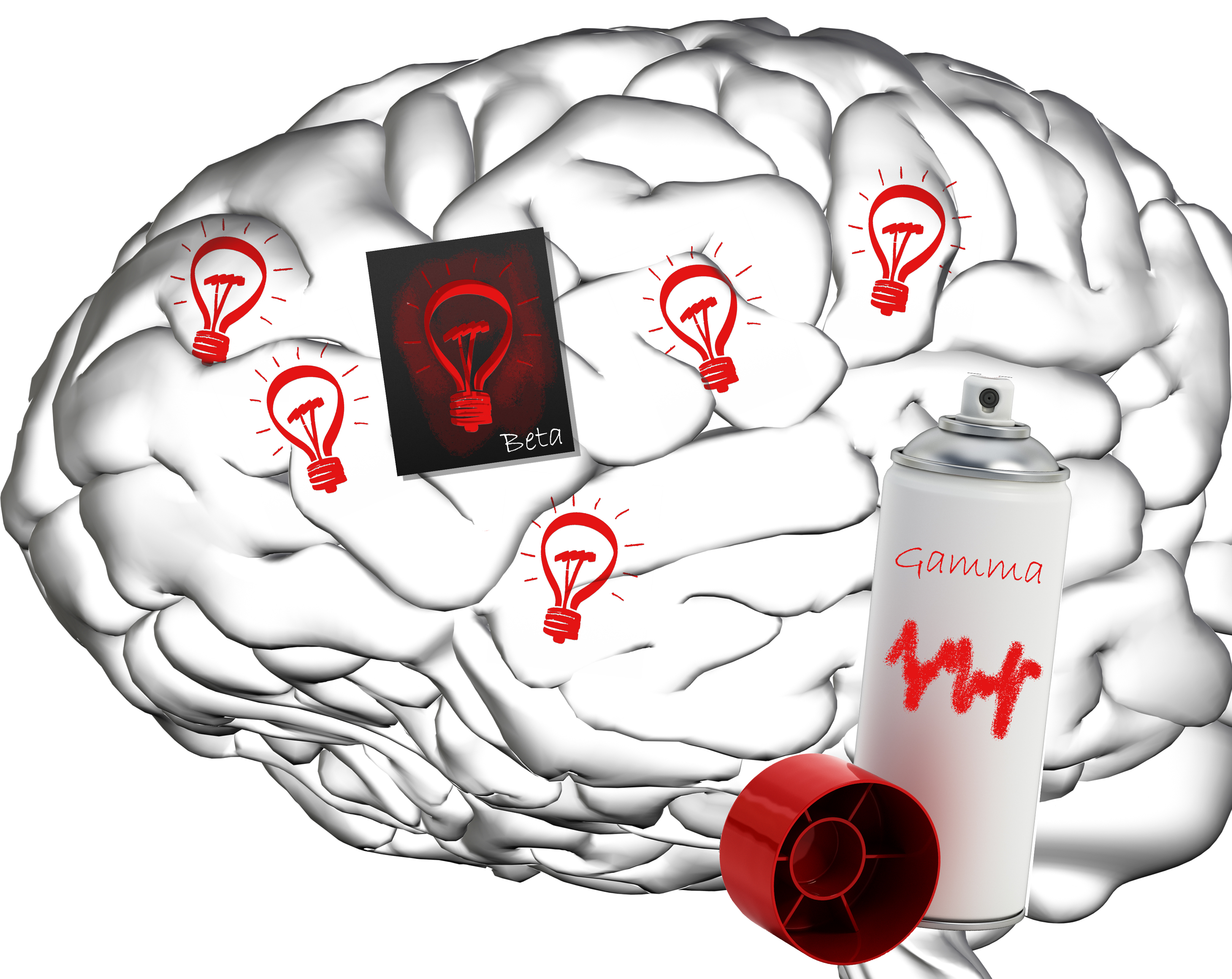
Some of the lab’s latest evidence suggests that beta rhythms apply this control of cognitive processes to physical patches of the cortex, essentially acting like stencils that pattern where and when gamma can encode sensory information into memory, or retrieve it. According to this theory, which Miller calls “ Spatial Computing ,” beta can thereby establish the general rules of a task (for instance, the back and forth turns required to open a combination lock), even as the specific information content may change (for instance, new numbers when the combination changes). More generally, this structure also enables neurons to flexibly encode more than one kind of information at a time, the authors write, a widely observed neural property called “mixed selectivity.” For instance, a neuron encoding a number of the lock combination can also be assigned, based on which beta-stenciled patch it is in, the particular step of the unlocking process that the number matters for.
In the new study Miller, Brincat and Roy suggest another advantage consistent with cognitive control being based on an interplay of large-scale coordinated rhythmic activity: “Subspace coding.” This idea postulates that brain rhythms organize the otherwise massive number of possible outcomes that could result from, say, 1,000 neurons engaging in independent spiking activity. Instead of all the many combinatorial possibilities, many fewer “subspaces” of activity actually arise, because neurons are coordinated, rather than independent. It is as if the spiking of neurons is like a flock of birds coordinating their movements. Different phases and frequencies of brain rhythms provide this coordination, aligned to amplify each other, or offset to prevent interference. For instance, if a piece of sensory information needs to be remembered, neural activity representing it can be protected from interference when new sensory information is perceived.
“Thus the organization of neural responses into subspaces can both segregate and integrate information,” the authors write.
The power of brain rhythms to coordinate and organize information processing in the brain is what enables functional cognition to emerge at that scale, the authors write. Understanding cognition in the brain, therefore, requires studying rhythms.
“Studying individual neural components in isolation—individual neurons and synapses—has made enormous contributions to our understanding of the brain and remains important,” the authors conclude. “However, it’s becoming increasingly clear that, to fully capture the brain’s complexity, those components must be analyzed in concert to identify, study, and relate their emergent properties.”
Related Articles
Study reveals a universal pattern of brain wave frequencies.
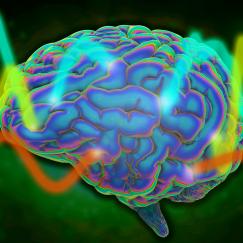
Anesthesia blocks sensation by cutting off communication within the cortex
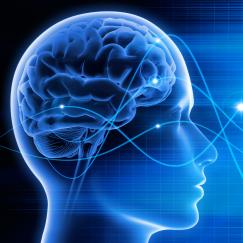
Anesthesia technology precisely controls unconsciousness in animal tests
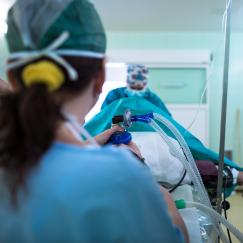
A multifunctional tool for cognitive neuroscience
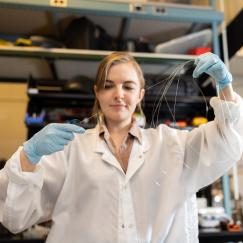
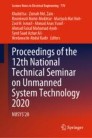
Proceedings of the 12th National Technical Seminar on Unmanned System Technology 2020 pp 93–104 Cite as
Overview of Underwater Communication Technology
- A. N. Jaafar 44 ,
- H. Ja’afar 44 ,
- I. Pasya 45 ,
- R. Abdullah 44 &
- Y. Yamada 46
- Conference paper
- First Online: 25 September 2021
1337 Accesses
7 Citations
Part of the book series: Lecture Notes in Electrical Engineering ((LNEE,volume 770))
Wireless transmission is important either in normal terrestrials or underwater network. It provides connection regardless of the obstacle surrounding the network. This technology can contribute to various benefits in terms of underwater environmental monitoring, oil and gas exploration, surveillance operation and military. In water environment, there are three common techniques that are normally available nowadays which rely on acoustic, optical or radio frequency (RF) channel. Acoustic is the earliest underwater communication technology which is widely used and it allows the longest range of communication, but it has some limitations in term of the low throughput and is also affected with the Doppler effects. Optical and RF technology are able to provide higher data rate compared to acoustic but both technologies have limitation in term of the transmission distance. However, RF technology is more suitable to be considered for short distance transmission with moderate data rate as the optical signal normally requires line-of-sight between transmitter and receiver link that is hard to be achieved in water that has higher particle inside. Therefore, suitable antenna design should be created to establish underwater communication that employs RF technology that can transmit higher data rate in short distance.
This is a preview of subscription content, log in via an institution .
Buying options
- Available as PDF
- Read on any device
- Instant download
- Own it forever
- Available as EPUB and PDF
- Compact, lightweight edition
- Dispatched in 3 to 5 business days
- Free shipping worldwide - see info
- Durable hardcover edition
Tax calculation will be finalised at checkout
Purchases are for personal use only
Lacovara P (2008) High-bandwidth underwater. Communications 42(1):93–102
Google Scholar
Marage J-P, Mori Y (2010) Sonar and Underwater Acoustics. Wiley-ISTE, USA
Barbeau M, Garcia-Alfaro J, Kranakis E, Porretta S (2018) The sound of communication in underwater acoustic sensor networks. In: Zhou Y, Kunz T (eds) Ad hoc networks. Lecture notes of the institute for computer sciences, social informatics and telecommunications engineering, vol 223. Springer, Cham. https://doi.org/10.1007/978-3-319-74439-1_2
Taylor P (2014) An overview of sonar and electromagnetic waves for underwater communication 37–41
Faggio A, Viscardi M, Coppola T, Rizzuto E (2018) A numerical code for underwater noise propagation 05017:1–7
Ainslie M (2010) Principles of sonar performance modelling. Springer Berlin Heidelberg, Netherland
Gkoura LK et al (2017) Underwater optical wireless communication systems: a concise review. Turbul Model Approaches—Curr State Dev Prospect Appl
Pompili D, Akyildiz IF (2009) Overview of networking protocols for underwater wireless communications. IEEE Commun Mag 47(1):97–102
Article Google Scholar
Wen D, Cai W, Pan Y (2016) Design of underwater optical communication system. Ocean. 2016—Shanghai, pp 0–3
Xu J, Song Y, Yu X, Lin A, Kong M, Han J (2016) Underwater wireless transmission of high-speed QAM-OFDM signals using a compact red-light laser, vol 24, no 8, pp 1–4
Schirripa Spagnolo G, Cozzella L, Leccese F (2020) Underwater optical wireless communications: overview. Sensors (Basel) 20(8)
Gussen C, Diniz P, Campos M, Martins W, Costa F, Gois J (2016) A survey of underwater wireless communication technologies. J Commun Inf Syst 31(1). https://doi.org/10.14209/jcis.2016.22
Simpson A, Ludu A, Cho HJ, Liu H, Beach D, Beach D (2014) Experimental and theoretical studies on visible light attenuation in water, pp 1–20
Bogie IS, Limited NC (1972) Conduction and magnetic signalling in the sea A background, vol 42, no 10
Aboderin O, Pessoa LM, Salgado HM (2017) Performance evaluation of antennas for underwater applications. In: 2017 Wireless days, Porto, pp 194–197. https://doi.org/10.1109/WD.2017.7918142
Wu C, Liu B (2018) Seawater electromagnetic propagation between two folded-dipoles at ISM-band. In: 2017 Sixth Asia-Pacific Conference on Antennas and Propagation (APCAP), pp 1–3
Karagianni EA (2015) Electromagnetic waves under sea: Bow-Tie antennas design for Wi-Fi underwater communications. Prog Electromagn Res M 41(January):189–198
Maher SM, Ali ZM, Mahmoud HH, Abdellatif SO, Abdellatif MM (2019) Performance of RF underwater communications operating at 433 MHz and 2.4 GHz. In: 2019 international conference on innovative trends in computer engineering (ITCE), February, pp 334–339 (2019)
Goh JH et al (2009) Underwater wireless communication system. In: Journal of physics: conference series, vol 178, Sensors & Their Applications XV, 5–7 Oct 2009, pp 2–8
Jimenez E et al (2016) Investigation on radio wave propagation in shallow seawater: Simulations and measurements. In: 2016 IEEE third underwater communications and networking conference (UComms), Lerici, pp 1–5. https://doi.org/10.1109/UComms.2016.7583453
Lanzagorta M (2012) Underwater communications. Synth Lect Commun 5(2):1–129
Lloret J, Sendra S, Ardid M, Rodrigues JJPC (2012) Underwater wireless sensor communications in the 2.4 GHz ISM frequency band. Sensors 12:4237–4264
Abdou AA et al (2013) A matched Bow-tie antenna at 433 MHz for use in underwater wireless sensor networks. J Phys Conf Ser 450(1)
Qureshi UM, Shaikh FK, Aziz Z, Shah SM, Sheikh AA, Felemban E, Qaisar SB (2016) RF path and absorption loss estimation for underwater wireless sensor networks in different water environments. Sensors (Basel), June 16 16(6):890. https://doi.org/10.3390/s16060890 . PMID: 27322263; PMCID: PMC4934316
Talkar SB, Fernandes M (2017) Simulation of monopole antenna for over the sea surface communication. In: 2017 international conference on computing methodologies and communication (ICCMC), Erode, pp 543–549. https://doi.org/10.1109/ICCMC.2017.8282524
Download references
Author information
Authors and affiliations.
Faculty of Electrical Engineering, Universiti Teknologi MARA, Cawangan Terengganu Kampus Dungun, 23000, Dungun, Terengganu, Malaysia
A. N. Jaafar, H. Ja’afar & R. Abdullah
Faculty of Electrical Engineering, Universiti Teknologi MARA, 40450, Shah Alam, Selangor, Malaysia
Malaysian-Japan International Institute of Technology (MJIIT), Universiti Teknologi Malaysia, Jalan Sultan Yahya Petra, 54100, Kuala Lumpur, Malaysia
You can also search for this author in PubMed Google Scholar
Corresponding author
Correspondence to A. N. Jaafar .
Editor information
Editors and affiliations.
Faculty of Electrical and Electronic Engineering, Universiti Tun Hussein Onn Malaysia, Parit Raja, Johor, Malaysia
Faculty of Electrical and Electronic Engineering, Universiti Malaysia Pahang, Pekan, Pahang, Malaysia
Zainah Md. Zain
School of Electrical and Electronic Engineering, Universiti Sains Malaysia, Engineering Campus, Nibong Tebal, Pulau Pinang, Malaysia
Rosmiwati Mohd-Mokhtar
Maziyah Mat Noh
Malaysia-Japan International Institute of Technology (MJIIT), Universiti Teknologi Malaysia, Jalan Semarak, Kuala Lumpur, Malaysia
Zool H. Ismail
Faculty of Electrical Engineering, Universiti Teknikal Malaysia Melaka, Durian Tunggal, Melaka, Malaysia
Ahmad Anas Yusof
Faculty of Ocean Engineering Technology and Informatics (FTKKI), Universiti Malaysia Terengganu, Kuala Nerus, Terengganu, Malaysia
Ahmad Faisal Mohamad Ayob
Department of Electrical and Electronic Engineering, Universiti Teknologi Petronas, Bandar Seri Iskandar, Perak, Malaysia
Syed Saad Azhar Ali
Faculty of Electrical and Electronic Engineering, Universiti Tun Hussein Onn, Parit Raja, Johor, Malaysia
Herdawatie Abdul Kadir
Rights and permissions
Reprints and permissions
Copyright information
© 2022 The Author(s), under exclusive license to Springer Nature Singapore Pte Ltd.
About this paper
Cite this paper.
Jaafar, A.N., Ja’afar, H., Pasya, I., Abdullah, R., Yamada, Y. (2022). Overview of Underwater Communication Technology. In: Isa, K., et al. Proceedings of the 12th National Technical Seminar on Unmanned System Technology 2020. Lecture Notes in Electrical Engineering, vol 770. Springer, Singapore. https://doi.org/10.1007/978-981-16-2406-3_8
Download citation
DOI : https://doi.org/10.1007/978-981-16-2406-3_8
Published : 25 September 2021
Publisher Name : Springer, Singapore
Print ISBN : 978-981-16-2405-6
Online ISBN : 978-981-16-2406-3
eBook Packages : Intelligent Technologies and Robotics Intelligent Technologies and Robotics (R0)
Share this paper
Anyone you share the following link with will be able to read this content:
Sorry, a shareable link is not currently available for this article.
Provided by the Springer Nature SharedIt content-sharing initiative
- Publish with us
Policies and ethics
- Find a journal
- Track your research

IMAGES
VIDEO
COMMENTS
Underwater Communications: Recent Advances. March 2019. Conference: International Conference on Emerging Technologies of Information and Communications (ETIC) At: JNEC, Bhutan. Authors: Mohammad ...
The collection and development of marine resources are almost inseparable from underwater communication. Therefore, the research on underwater communication technology is attracting emerging attention. Moreover, underwater wireless communication (UWC) plays a vital role in underwater navigation, and it is also a key technology for underwater ...
The widespread adoption of underwater wireless communications (UWC) has become an important research area to address a variety of military and commercial applications, and there is growing interest in investigating underwater environments in numerous applications. With the advancement of UWC, the underwater Internet of Things (IoUT) has emerged, and various practical applications are expected ...
In the booming ocean exploration, underwater equipment and technology is attracting more and more attention 1,2,3,4,5.Particularly, obtaining underwater wireless communication has always been a ...
1.3 Paper Structure. This paper consists of eleven sections: UWC as an overview and literature material is discussed in Sect. 2.The current project schemes, new paradigms, and their contributions are suggested by Sect. 3.The limited frequency range of electromagnetic communication and main issues during propagation in the underwater medium are widely discussed in Sect. 4.
Underwater Optical Wireless Communication (UOWC) is not a new idea, but it has recently attracted renewed interest since seawater presents a reduced absorption window for blue-green light. Due to its higher bandwidth, underwater optical wireless communications can support higher data rates at low latency levels compared to acoustic and RF counterparts. The paper is aimed at those who want to ...
Therefore, this journal aims to present the latest research results, progress and reviews in the field of underwater communication and networks, and to provide new ideas and new technologies to further promote the research and development of underwater information networks. The scope of this Special Issue includes, but is not limited to:
"The paper is the first to bring Van Atta Reflector array technique into underwater backscatter settings and demonstrate its benefits in improving the communication range by orders of magnitude. This can take battery-free underwater communication one step closer to reality, enabling applications such as underwater climate change monitoring ...
Feature papers represent the most advanced research with significant potential for high impact in the field. ... offshore exploration, and oceanographic studies. It is a promising candidate for underwater communication, specifically using blue and green light. ... secure, and high-speed UOWC, research has focused on developing new communication ...
1 Introduction. Underwater wireless communication technology is widely used in resource exploration [], abnormal sea state monitoring [], sensor monitoring [], underwater imaging [], underwater robots [], and airspace integration [].It is important advantages for blue or green laser, such as good penetration ability, strong anti-interference, large communication depth, and high transmission ...
The 21st paper entitled "State‑of‑the‑Art Underwater Acoustic Communication Modems: Classifications, Analyses and Design Challenges. The main contribution of the article is a comparative analyses of commercial and research modems based on their characteristics and design constraints, in order to describe the current trends and more ...
This survey paper analyzes recent literature on underwater robotics communication, a fundamental enabler for scaling up exploration and intervention with multiple robots [15, 16].First, we provide a brief historical overview of underwater communication; then, we present recent trends of related research together with open problems and future directions.
Therefore, even if submarine optical communication systems are beginning to be commercially available [29], extensive research is being carried out on methodologies and systems for the transfer of broadband optical signals at higher distances. In the future many underwater applications will use optical communication. However, UOWC
Underwater wireless information transfer is of great interest to the military, industry, and the scientific community, as it plays an important role in tactical surveillance, pollution monitoring, oil control and maintenance, offshore explorations, climate change monitoring, and oceanography research. In order to facilitate all these activities, there is an increase in the number of unmanned ...
Together with sensor technology and vehicular technology, wireless communications will enable new applications ranging from environmental monitoring to gathering of oceanographic data, marine archaeology, and search and rescue missions. While wireless communication technology today has become part of our daily life, the idea of wireless undersea communications may still seem far-fetched ...
In the past few decades, need and thus interest in underwater voice communication research is growing due to its application in submarines, deep water manned submersibles, and diver's communication. Compared to the initial Acoustic telephones, performance of the present systems is improved due to the continuous research interest. Very few review papers are available to provide the history of ...
This paper presents a new method for encrypting holographic information based on optical and acoustic signals called a Virtual Optical Holographic Encryption (VOHE) system for underwater communications that can be applicable for communications between deep submergence research vehicles. The transmission medium is composed [...] Read more.
Underwater wireless communication is an advanced research area need to be explored extensively. This topic is highly significant for various purposes, starting from aquatic pollution control, marine life monitoring, and quality of the water and moreover signal transmission. Different sensors can be used under water for the above said applications.
João Alves (UComms 2021 General Chair), OES AdCom member The UComms conference series, Organised by the NATO Centre for Maritime Research and Experimentation (CMRE), is focused on working towards a deep understanding of the propagation of communication signals underwater and the performance of necessary higher-level protocols with the objective of supporting the intelligent choice of […]
Find the latest published documents for underwater wireless communication, Related hot topics, top authors, the most cited documents, and related journals ... In view of the remarkable results achieved by artificial intelligence techniques in the field of underwater wireless communication research, we propose an object detection method based on ...
Historically dismissed solely as byproducts of neural activity, brain rhythms are actually critical for organizing it, write Picower Professor Earl Miller and research scientists Scott Brincat and Jefferson Roy in Current Opinion in Behavioral Science. And while neuroscientists have gained tremendous knowledge from studying how individual brain ...
1.2 Optical Channel. In underwater environment, optical channel can be used for short range communication. It provides higher data rate and better bandwidth as compared to the acoustic technology. Therefore, in the case of short-range communication, this technology can replace the acoustic channel.