
- April 24, 2024 | 30 Times Clearer – Scientists Develop Improved Mid-Infrared Microscope
- April 24, 2024 | Shedding Pounds, Dodging Cancer: The Life-Saving Promise of Bariatric Surgery
- April 24, 2024 | Quantum Computing Meets Genomics: The Dawn of Hyper-Fast DNA Analysis
- April 24, 2024 | Scientists Turn to Venus in the Search for Alien Life
- April 24, 2024 | NASA Astronauts Enter Quarantine As Boeing Starliner Test Flight Approaches

Expanding the Universe’s Habitable Zone: New Research Reshapes Our Search for Alien Worlds
By The Hebrew University of Jerusalem February 1, 2024
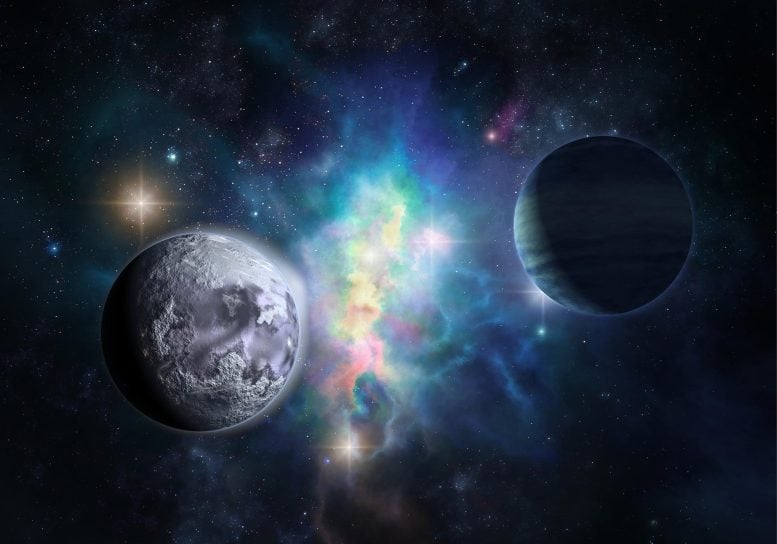
New research redefines the Habitable Zone of exoplanets by considering subglacial liquid water, extending potentially habitable conditions beyond traditional boundaries. Supported by recent JWST observations, this study broadens the scope for finding life on exoplanets, especially those orbiting M-dwarf stars.
Professor Amri Wandel from the Hebrew University of Jerusalem has introduced groundbreaking research with the potential to transform our understanding of habitable exoplanets. His latest study, published in the Astronomical Journal , highlights the importance of subglacial liquid water in expanding the traditional definition of the Habitable Zone .
The classical Habitable Zone, often colloquially referred to as the “Goldilocks Zone,” typically defines the region around a star where conditions allow the presence of surface liquid water and, by extension, life as we understand it. However, Professor Wandel’s research offers a fresh perspective by illustrating that the existence of subglacial liquid water can considerably extend this zone.
Expanding the Habitable Zone Inward and Outward
One of the primary discoveries of this research is the potential to expand the Habitable Zone inwards for tidally locked planets closely orbiting M-dwarf stars, which are frequently regarded as candidates for detecting spectral evidence for life (so-called biosignatures) in exoplanets. The study delineates how an atmosphere and liquid water could coexist on these planets, pushing the limits of the Habitable Zone further than previously assumed.
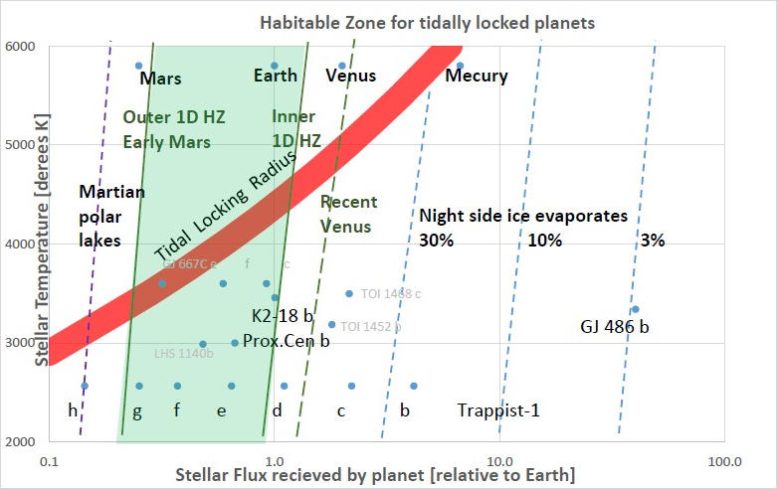
Boundaries of the Habitable Zone under various climate and atmospheric models as a function of radiative flux received from the host star, relative to Earth. Dashed lines represent the extended boundaries due to sub-glacial liquid water suggested in this work. Solid green lines and green shading denote the conservative Habitable Zone boundaries. The red curve marks the tidal locking zone (planets to the right of it are locked). Circles denote the terrestrial planets in the solar system and a few .Earth-sized exoplanets orbiting M-dwarf stars. Credit: Amri Wandel
Moreover, the research postulates that subglacial liquid water can also broaden the Habitable Zone beyond the outer limits of the conservative Habitable Zone. These findings unlock the possibility of liquid water on a more diverse range of exoplanets than previously envisioned, presenting tantalizing opportunities for the search for extraterrestrial life.
Connection with Recent Observations
A noteworthy implication of this research is its connection to recent observations made by the James Webb Space Telescope (JWST). The potential identification of atmospheric water vapor on GJ 486 b, a rocky Earth-sized exoplanet , and the evidence for an ocean on K2-18b, a Super-Earth exoplanet, hint at the existence of liquid water, possibly organic chemistry, and the potential for life on such celestial bodies. This discovery provides empirical substantiation to address the long-standing question of whether exoplanets orbiting M-dwarf stars can sustain habitable conditions.
Professor Wandel remarked, “This work demonstrates that the Habitable Zone of red dwarfs is likely significantly broader than previously assumed, and planets within it have the capacity to maintain water and an atmosphere. The latter conclusion is empirically supported by recent findings of water on such exoplanets by the Webb Telescope, particularly in K2-18 b, as predicted in the article submitted two months prior. In particular, it may optimize the target allocation and priority for biosignature research by JWST.”
Professor Wandel’s research elucidates how water on terrestrial planets closely orbiting M-dwarf stars may endure within a subglacial melting layer, presenting a unique perspective on the sustainability of liquid water. The study further explores how the detection of water on various exoplanets can aid in constraining their atmospheric characteristics.
In conclusion, Professor Amri Wandel’s research spotlights the transformative potential of subglacial liquid water in expanding the Habitable Zone of exoplanets. This discovery not only advances our comprehension of habitable environments in the cosmos but also illuminates the prospect of life beyond our planet.
Reference: “Extended Habitability of Exoplanets Due to Subglacial Water” by Amri Wandel, 3 November 2023, The Astronomical Journal . DOI: 10.3847/1538-3881/ad0045
More on SciTechDaily
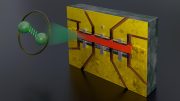
UTe2 Unleashes New Superconductivity Secrets
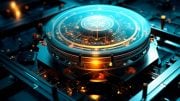
Quantum Control Breakthrough a Game Changer for Next-Gen Electronics and Computers
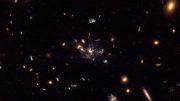
CSIRO Detects Raw Materials Used in the Making of the First Stars
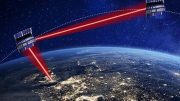
UK Space Agency Funds Development of New Laser-Based Satellite Communications System
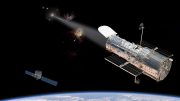
Emission Spectrum From Blazar PKS 1424+240 Deviates From Expectations
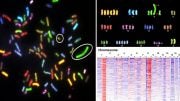
UCLA Study Shows DNA Patterns Can Unlock How Glucose Metabolism Drives Cancer
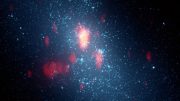
Young Star Cluster Displays Highly Efficient Star Formation
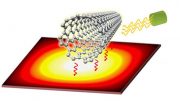
Researchers Develop Spaser Made of Graphene and Carbon Nanotubes
1 comment on "expanding the universe’s habitable zone: new research reshapes our search for alien worlds".
After much work on extra-terrestial life, I’m delighted to announce the completion of this project and the success of obtaining a conclusive result and I’m afraid to say that we are all alone in this sheer, vast and expansive waste of matter and space. I’m also happy to say that new light has been thrown, through our findings, on this very fascinating subject. The project involed an ultra-modern star-tracker capable of detecting life millions of billions of light years away. It’s construction and operation is grounded on the fact that aliens can’t be in the cosmos and get away without leaving trails. We suspended a microphone in outer space and waited for over 20years for signs but found none. Therefore, there are no such things as aliens. Thanks
Leave a comment Cancel reply
Email address is optional. If provided, your email will not be published or shared.
Save my name, email, and website in this browser for the next time I comment.
Thank you for visiting nature.com. You are using a browser version with limited support for CSS. To obtain the best experience, we recommend you use a more up to date browser (or turn off compatibility mode in Internet Explorer). In the meantime, to ensure continued support, we are displaying the site without styles and JavaScript.
- View all journals
- Explore content
- About the journal
- Publish with us
- Sign up for alerts
- Published: 01 November 2017
Exoplanet hunters rethink search for alien life
- Alexandra Witze
Nature volume 551 , pages 421–422 ( 2017 ) Cite this article
1919 Accesses
1 Citations
248 Altmetric
Metrics details
- Astronomy and astrophysics
- Planetary science
This article has been updated
Astronomers expand ideas of how chemistry and geology could affect chances for life on other worlds.
Laramie, Wyoming
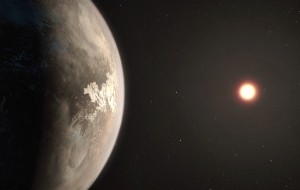
Steve Desch can see the future of exoplanet research, and it’s not pretty. Imagine, he says, that astronomers use NASA’s upcoming James Webb Space Telescope to scour the atmosphere of an Earth-mass world for signs of life . Then imagine that they chase hints of atmospheric oxygen for years — before realizing that those were false positives produced by geological activity instead of living things.
Desch, an astrophysicist at Arizona State University in Tempe, and other planet hunters met from 13-17 November in Laramie, Wyoming, to plot better ways to scout for life beyond Earth. Many are starting to argue that the standard definition of habitability — having liquid water on a planet’s surface — is not the factor that should guide exoplanet exploration. Instead, the scientists say, the field should focus on the chances of detecting alien life , should it exist.
“Planets can be habitable and not have life with any impact,” Desch told researchers at the meeting.
It turns out that water worlds may be some of the worst places to look for living things. One study presented at the meeting shows how a planet covered in oceans could be starved of phosphorus, a nutrient without which earthly life cannot thrive. Other work concludes that a planet swamped in even deeper water would be geologically dead, lacking any of the planetary processes that nurture life on Earth.
“Habitability is not only about finding the signature of an alien life form taking a deep breath,” says Elizabeth Tasker, an astronomer and exoplanet researcher at the Japan Aerospace Exploration Agency's Institute for Space and Aeronautical Sciences in Sagamihara. It’s also about how a planet’s geology and chemistry interconnect to create a welcoming or hostile environment, she says — complicating the search for extraterrestrial life.
Surf and turf
Astronomers have catalogued thousands of exoplanets, of which more than a dozen are potentially habitable. The most recent, announced on 15 November, is Ross 128b, which is 3.4 parsecs (11 light years) away from Earth. It resembles the target that scientists have spent decades hunting: an Earth-sized planet orbiting a nearby star, probably at the right distance to allow liquid water.
Most of these planets have some qualities that stop them from being true Earth twins. Ross 128b orbits a cool dwarf star rather than a Sun-like host, for instance. But Tasker says the usual metrics that scientists use to rank how habitable a world is, such as its location relative to its star or how closely it resembles Earth, are misguided 1 .
To figure out how to parcel out valuable observing time, some scientists suggest targeting planets that, like Earth, are thought to have a mix of ocean and land. That's because worlds with nothing but water on their surfaces may not have key nutrients available in forms that can support life — if it is based on the same chemistry as life on Earth.
“We have this stereotype that if we have oceans, we have life,” says Tessa Fisher, a microbial ecologist at Arizona State. But her recent work contradicts this idea. Fisher and her colleagues studied what would happen on an “aqua planet” with a surface that is almost or completely covered by enough water to fill Earth’s oceans five times.
On Earth, rainwater hitting rocks washes phosphorus and other nutrients into the oceans. But without any exposed land, there is no way for phosphorus to enrich water on an aqua planet over time, Fisher reported at the Laramie meeting. There would be no ocean organisms, such as plankton, to build up oxygen in the planet’s atmosphere, she says — making this type of world a terrible place to find life.
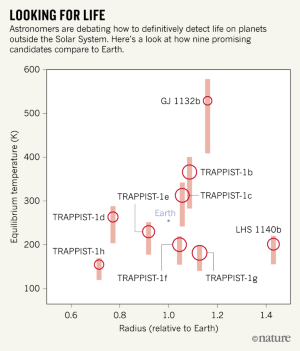
Wet blanket
The wettest planets would run into a different sort of trouble, says Cayman Unterborn, a geologist at Arizona State who analysed the planet-wide effects of having as much as 50 Earth oceans’ worth of water. The sheer weight of all that liquid would exert so much pressure on the sea floor that the planet’s interior would not melt at all, Unterborn found.
Planets need at least some internal melting to sustain geological activity, such as plate tectonics, and to provide the right geochemical environment for life. In this case, Unterborn says, “too much water is too much of a good thing.”
Water-rich worlds are easy to make. Many planets are likely to have formed far from their parent star, Tasker says, in chilly temperatures where they could have coalesced from fragments of rock and lots of ice. If such a planet later migrated closer to its star, the ice would melt and cover the surface in vast oceans. Some of the seven small planets orbiting the star TRAPPIST-1 , which is 12.6 parsecs (41 light years) from Earth, are thought to have substantial water on their surfaces 2 .
Instead of instinctively studying such water worlds, Tasker says, astronomers need to think more deeply about how planets have evolved through time. “We need to look carefully at picking the right planet,” she says.
The James Webb Space Telescope is set to launch in 2019. Once in space, the telescope will spend much of its time studying potentially Earth-like worlds . Researchers have already begun to analyse how oxygen, methane or other ‘biosignature’ gases in exoplanet atmospheres might appear to the telescope’s view 3 .
Towards the end of the Laramie meeting, attendees voted on whether scientists will find evidence of life on an exoplanet by 2040. They were not optimistic: 47 said no and 29 said yes. But a greater share was willing to bet that life would be found on another world in the 2050s or 2060s.
That's presumably enough time to work through the debate over which worlds are the best to target.
Change history
20 november 2017.
This story misstated the outcome of the vote at the Laramie meeting as 47% no to 29% yes. In fact, the result was 47 votes yes, 29 votes no.
Tasker, E. et al. Nature Astron. 1 , 0042 (2017).
Article Google Scholar
Bourrier, V. et al. Astron. J. 154 , 121 (2017).
Article ADS Google Scholar
Morley, C. V., Kreidberg, L., Rustamkulov, Z., Robinson, T. & Fortney, J. J. Preprint at http://arxiv.org/abs/1708.04239 (2017).
Download references
You can also search for this author in PubMed Google Scholar
Related links
Related links in nature research.
These seven alien worlds could help explain how planets form 2017-Feb-22
Earth-sized planet around nearby star is astronomy dream come true 2016-Aug-24
Planet hunters seek new ways to detect alien life 2016-Jul-27
The truth about exoplanets 2016-Feb-17
The exoplanet files 2015-Nov-18
Climate scientists join search for alien Earths 2015-Apr-17
Related external links
Nexus for Exoplanet System Science
Rights and permissions
Reprints and permissions
About this article
Cite this article.
Witze, A. Exoplanet hunters rethink search for alien life. Nature 551 , 421–422 (2017). https://doi.org/10.1038/nature.2017.23023
Download citation
Published : 01 November 2017
Issue Date : 23 November 2017
DOI : https://doi.org/10.1038/nature.2017.23023
Share this article
Anyone you share the following link with will be able to read this content:
Sorry, a shareable link is not currently available for this article.
Provided by the Springer Nature SharedIt content-sharing initiative
Quick links
- Explore articles by subject
- Guide to authors
- Editorial policies
Sign up for the Nature Briefing newsletter — what matters in science, free to your inbox daily.

- International edition
- Australia edition
- Europe edition
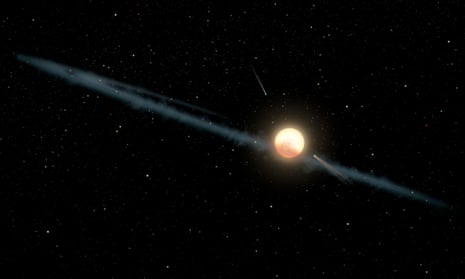
‘Is it aliens?’: how a mysterious star could help the search for extraterrestrial life
Scientists hope studies into Boyajian’s star could lead to enhanced techniques for identifying distant planetary civilisations
I t is our galaxy’s strangest star, a flickering globe of light whose sporadic and unpredictable output has baffled astronomers for years. But now the study of Boyajian’s star is being promoted as a research model that could help in one of the most intriguing of all scientific quests: finding intelligent life on other worlds.
This is the argument that Oxford University astrophysicist Prof Chris Lintott will make at a public lecture – Is it Aliens? The Most Unusual Star in the Galaxy – at a Gresham College lecture in Conway Hall, central London on Monday. His prime target will be Boyajian’s star, sometimes nicknamed Tabby’s star after scientist Tabetha Boyajian, in the constellation Cygnus whose odd dimming and brightening has been the subject of intense study by space probes and observatories in recent years.
“Its behaviour is extraordinary,” Lintott told the Observer . “It has rapid, random bursts where its brightness drops dramatically and then returns. There is no pattern to it. It flickers as if somebody was playing with its dimmer switch. There is no other star like this in our galaxy.”
Boyajian’s star was studied in detail by the Kepler space observatory in 2012 when its erratic behaviour was first uncovered. These observations indicated that a huge mass of matter circles the star in tight formation and sporadically blocks its light.
But what was the nature of this vast mass of material? Dust rings, disintegrating comets and swarms of asteroids were all put forward as explanations. However, most attention went to the theory, proposed by scientists at Penn State University, that the eclipsing mass could be a huge alien megastructure.
Such constructions were proposed by physicist Freeman Dyson, who argued that some alien civilisations might be advanced enough to build vast arrays of solar panels around their home stars to capture its heat and light. Dubbed Dyson spheres or swarms by astronomers, the great orbiting edifices would be used to power these distant civilisations.
The idea that astronomers had stumbled on such a structure triggered headlines round the globe – though not for long. Subsequent research has now undermined the idea . “We have found that light of different wavelengths is blocked in different amounts: exactly what you would expect from starlight passing through a dust cloud,” said Lintott.
Boyajian’s eclipsing mass is probably a dust cloud produced when a planet grazed too close to its surface and was torn apart. Nevertheless, the study of the strange object is important because it highlights techniques that are destined to become increasingly important as attempts to pinpoint alien civilisations intensify in coming years, Lintott argues.
Humanity could become radio-quiet in about 50 years as a result – and that will probably be true for civilisations on other worlds“The search for extraterrestrial intelligence [Seti] is changing,” he said. “We have relied in the past almost exclusively on radio telescopes to detect broadcasts from alien civilisations just as our radio and TV transmissions could reveal our presence to them. However, to date, we have heard absolutely nothing.”
Nor should we be surprised, Lintott argues. “Humanity has already passed its peak radio wave output because we are increasingly using narrow beam communications and fibre-optic cables, rather than beaming out TV and radio signals into the general environment.”
Humanity could become radio-quiet in about 50 years as a result – and that will probably be true for civilisations on other worlds, he added. “They will have gone radio silent after a while, like us. So Seti radio telescopes will need to be augmented with other ways of seeking aliens . We are going to have to be more creative about what we’re searching for in the data and find unusual things that reveal they are the handiwork of aliens.”
after newsletter promotion
Searching for Dyson spheres – huge planet-sized panels of solar arrays – will be one key route, though other ways of pinpointing the handiwork of aliens will also be needed. An example would be provided by an alien civilization that mined asteroids near their home planet, an endeavour that would create clouds of interplanetary dust that would reveal themselves from their infrared radiation.
“And while aliens may not reveal themselves through radio transmissions, they could easily betray their presence from their radar emissions that they use to guide their aircraft and spaceships,” added Lintott. “Again, we need to look at these wavelengths for signs of their existence.”
Such work will require analyses of colossal stores of data and the story of Boyajian’s star also provides a key demonstration of how they would be done. Its secrets were revealed by an army of citizen scientists, members of the public who collected and analysed the vast reams of data from the instruments that probed the star, said Lintott.
“It was their combined analyses of data about Boyajian’s that showed it was behaving in a very odd manner – and it is very likely they will be involved in fingering other weird stars in our galaxy in future projects. And you never know, the next time they might hit pay dirt.”
- The Observer
- Seti (Search for Extraterrestrial Intelligence)
Most viewed

An official website of the United States government
The .gov means it’s official. Federal government websites often end in .gov or .mil. Before sharing sensitive information, make sure you’re on a federal government site.
The site is secure. The https:// ensures that you are connecting to the official website and that any information you provide is encrypted and transmitted securely.
- Publications
- Account settings
Preview improvements coming to the PMC website in October 2024. Learn More or Try it out now .
- Advanced Search
- Journal List
- Astrobiology

Alien Mindscapes—A Perspective on the Search for Extraterrestrial Intelligence
Advances in planetary and space sciences, astrobiology, and life and cognitive sciences, combined with developments in communication theory, bioneural computing, machine learning, and big data analysis, create new opportunities to explore the probabilistic nature of alien life. Brought together in a multidisciplinary approach, they have the potential to support an integrated and expanded Search for Extraterrestrial Intelligence (SETI 1 ), a search that includes looking for life as we do not know it. This approach will augment the odds of detecting a signal by broadening our understanding of the evolutionary and systemic components in the search for extraterrestrial intelligence (ETI), provide more targets for radio and optical SETI, and identify new ways of decoding and coding messages using universal markers. Key Words: SETI—Astrobiology—Coevolution of Earth and life—Planetary habitability and biosignatures. Astrobiology 16, 661–676.
1. Introduction
T he Kepler mission and ground-based observatories have revealed thousands of exoplanets in small sectors of our galaxy alone, thus providing powerful evidence that our solar system is not an exception but simply one out of countless others in the Universe ( e.g. , Borucki et al. , 2010 ; Kopparapu et al. , 2013 ; Seager, 2013 ; Batalha, 2014 ; Gaidos et al. , 2014 ; Hatzes, 2014 ; Quintana et al. , 2014 ; Macintosh et al. , 2015 ). What remains unanswered, however, is whether life—simple or complex—exists beyond Earth.
For nearly 20 years, astrobiology has brought a multidisciplinary vision to this quest through three fundamental science questions: (1) How does life begin and evolve? (2) Does life exist elsewhere in the Universe? (3) What is the future of life on Earth and beyond? In the 2008 version of its roadmap (Des Marais et al. , 2008 ), astrobiology proposed a global approach to these questions through a broad research program with the goals to understand the formation and evolution of habitable planets, to explore and characterize the evolution of planetary environments favorable to life's development in the Solar System and beyond, and to find methods to detect the signatures of life on early Earth and other worlds.
Thirty-five years before the creation of astrobiology, a very similar intellectual approach had been articulated by Frank Drake in the “Drake equation” ( Section 2 ) but with a different intent. The Drake equation provided a probabilistic model to estimate the number of actively communicating extraterrestrial civilizations in the Milky Way, and was formulated around a technological imperative (radio astronomy) and a philosophical question: Are we alone?
Decades of perspective on both astrobiology and the Search for Extraterrestrial Intelligence (SETI) show how the former has blossomed into a dynamic and self-regenerating field that continues to create new research areas with time, whereas funding struggles (Garber, 1999 ) have left the latter starved of young researchers and in search of both a long-term vision and a development program. A more foundational reason may be that, from the outset, SETI is an all-or-nothing venture where finding a signal would be a world-changing discovery, while astrobiology is associated with related fields of inquiry in which incremental progress is always being made.
Yet in the same way astrobiology approaches the understanding of life in the Universe, SETI carries in its quest fundamental and unique questions that are central to the understanding of who, what, and where intelligent life is, and how to find it. While Are we alone? is their philosophical and popular expression (Zuckerman and Tarter, 1979 ; Tarter and Chyba, 1999 ), their scientific formulation can be expressed in these questions: How abundant and diverse is intelligent life in the Universe? How does intelligent life communicate? How can we detect it? If understanding life is the thread woven through the astrobiology roadmap, understanding how intelligent life interacts with its environment and communicates information is central to SETI.
In the following sections, we examine how scientific and technological advances are now allowing us to explore these questions in ways that were not previously possible, and to build the foundation of a long-term, multidisciplinary, and integrated vision for SETI. Here, we offer a perspective on how we could scientifically augment the odds of finding extraterrestrial life (ET) and an invitation to change our mind-set in doing so.
2. Historical Pathway to ET
While the scientific foundation for a living universe was established in the 16 th century with the Copernican revolution, the nature of advanced civilizations remained the domain of philosophers and fiction writers for a few more centuries ( e.g. , Descartes, 1644 ; de Fontenelle, 1686 ; Voltaire, 1752/2002 ; Kant, 1755/2009 ; Swendenborg, 1758 ; Flammarion, 1872 —reviews by Dick, 1984 ; Crowe, 2011 ). The latter populated the Universe and our psyche with beings and worlds that were no more than idyllic or nightmarish versions of ourselves, our society, and our biosphere. By the end of the 19 th century, the advent of new technologies opened a different epistemological chapter for SETI. At that point, the quest for alien civilizations started to transition from a justifiable belief to a technology-based endeavor. Electricity and radio were first proposed as means to communicate with our close neighbor (Seifer, 1996 ) after Schiaparelli described channels— canali , in Italian—on Mars that later, Lowell ( 1906 ) erroneously claimed were artificial canals constructed by Martians. Over a half-century later, Cocconi and Morrison ( 1959 ) became the first to point out the possibility of searching for aliens in the microwave spectrum. Their expectation was that extraterrestrial signals could be pulse-modulated ( e.g., Shostak, 2011a ), and they proposed to examine solar-mass and red dwarf stars within 15–50 light-years of Earth.
The conclusion of their article in Nature contained key comments that still resonate today. These comments include the realization that technology was finally available to test scientific hypotheses consistent with observational and theoretical astronomy, an understanding of the transformative philosophical and practical implications of a potential contact with an alien civilization ( e.g., Almár and Tarter, 2011 ; Eliott, 2011a ), and a concern that their work would be consigned to science fiction by many.
Independently from Cocconi and Morrison, astronomer Frank Drake had been formulating similar plans to conduct an actual search, and within a year of the publication, Project Ozma was launched at Green Bank (Schuch, 2011 ). Modern SETI was born and set out to search for ET's presence through narrowband radio astronomy. Broadband optical astronomy became an additional search tool in 1998 with OSETI (Ansbro, 2001 ), although the idea was previously suggested by physics Nobel laureate Charles Townes, whose research led to the maser and the laser ( e.g., Schwartz and Townes, 1961 ; Wright et al. , 2014 ).
Since 1961, SETI's intellectual framework has been centered on a probabilistic argument, the now-famous Drake equation:

where N is the number of civilizations in the Milky Way whose electromagnetic emissions are detectable; R * is the average rate of star formation in our galaxy; f p is the fraction of those stars with planetary systems; n e is the number of planets, per solar system, with an environment suitable for life; f l is the fraction of these planets that actually develop life; f i is the fraction of life-bearing planets that develop intelligent life; f c is the fraction of civilizations that develop a technology that releases detectable signs of their existence into space; and L is the length of time such civilizations release detectable signals into space. For reviews and discussions about the Drake equation, see, for example, the works of Shostak ( 1998 ), Vakoch and Harrison ( 2011 ), Maccone ( 2012 ), Vakoch ( 2014 ), Vakoch and Dowd ( 2015 ), and Darling and Schulze-Makuch ( 2016 ).
Both lauded and criticized from the day it was formulated, the Drake equation clearly represented a watershed moment in science that gave humanity a glyph with which to spell alien life for the first time. Included as a forethought to the 1961 Green Bank Search for Extraterrestrial Intelligence meeting agenda (Drake, 2011 ), the so-called equation proved to be anything but. Consisting of a combination of quantitative and speculative factors that were solely meant to engage a discussion within the scientific community on the potential number of extraterrestrial civilizations willing and able to communicate in our galaxy, it quickly became SETI's signature. In hindsight, it was much more.
3. A Roadmap
The term equation is misleading in this context. Nor does the label probabilistic argument fully capture what the formulation truly represents, and the succession of some of its variables could be criticized. For instance, between f i and f c the notion of intelligence abruptly gives way to that of civilization, when they are, in fact, distinct notions. On our planet, one has not been demonstrated to necessarily lead to the other. Earth has developed a number of intelligent species, some of which use tools, while others are organized in hierarchical societies. Ultimately, the term technological civilization is what differentiates humankind, that is, an advanced stage of social development and organization, where scientific knowledge is applied for practical purposes on an industrial scale. In our case, this technology allows our species to scientifically explore and characterize our planet, the Solar System, and the Universe—and, on Earth, this is unique to the human species.
Nevertheless, the Drake equation was essentially the first roadmap and the first holistic vision of the search for life in the Universe (Tarter, 2007 )—an approach that would become the trademark of the nascent field of astrobiology 35 years later (Des Marais et al. , 2008 ). Even at this seminal time, it captured in one expression the notions of extrasolar planetary systems, habitable zones, habitable planets and environments, the transition from chemistry to biology, life on and beyond Earth, and the evolution of intelligence, civilization, and technology.
In the epistemological sense, the Drake equation provided a reductionist approach by scientifically breaking down the question of technologically advanced civilizations into simpler and smaller parts (the variables). Ultimately, it overlooked its own revolutionary vision as SETI focused on the application of one technology to search for Sun-like stars and red dwarfs ( e.g. , Tarter, 1979 , 2004 ; Billingham et al. , 1980 ; Gulkis et al. , 1981 ; Heath et al. , 1998 ; Turnbull and Tarter, 2003 ; Tarter et al. , 2007 ). This rationale remains largely unchanged today despite advances in exploration and science that are relevant to the factors of the equation. As it stands, SETI does not search for all life or for all intelligent life. It focuses exclusively on technologically advanced life.
At the time of its formulation, the Drake equation was simply meant to be a tool to gauge how many technologically advanced civilizations might be “out there.” The focus was clearly on the end-number ( N ) to evaluate whether radio astronomy and astrophysics could provide successful strategies to intercept ET's signal across interstellar distances (Tarter, 2001a ). The focus was not on trying to understand or quantify the processes taking place within each of the factors that lead to the next. Drake himself stated that his equation was not aimed at searching for primordial or primitive life-forms (Drake, 2011 ). Rather, it was aimed at the radio search. This explains SETI's path from the moment the Tatel Telescope at Green Bank was turned toward Tau Ceti and Epsilon Eridani for the first time. This might also explain why the radio receivers have remained silent since (see Sections 4 and 5 ).
Over the years, and with the exception of a clear connection to exoplanet detection (Tarter, 2001a , 2001b ; Harp et al. , 2015 ) and other astronomical fields, SETI around the world has evolved, for most, independently from core disciplines that rightly should be considered central to its understanding of what alien life could be and, therefore, to an understanding of how to optimize a detection strategy. While individual efforts exist, they have yet to be integrated into one vision and effectively support each other and existing projects ( e.g., Fig. 1 ). It could be argued, but with only partial justification, that the reasons for SETI's all-or-nothing venture are grounded in historical circumstances. Very little data were available to quantify the factors of the Drake equation at the time it was formulated, and most knowledge about the Solar System and the Universe was still primarily coming from ground-based astronomy and astrophysics. President Kennedy was a year away from declaring the race to the Moon, and orbiters were sent to Mars and Venus for the first time. It was a time of discoveries to come, in which the young science of radio astronomy was viewed as a unique tool to help humanity make what would be a world-altering discovery.

Example of an integrated path to ET detection showing basic science questions ( e.g. , who and what are we searching for, and where? ), key multidisciplinary science concepts, and relevant exploration methods ( how do we investigate? ). The combination of questions and approaches results in a directed and scientifically rich vision to ET detection, whose output is a reasoned exploration target. Since 1960, SETI's exploration strategy has prioritized the detection method (radio and optical searches) without addressing the fundamental nature of who and what ET could be, which has fundamental implications for where and how to search for it. The science concepts and methods shown here are discussed in the text.
4. The Evolution of N in the Past Decades
By contrast to this time, large databases are now available in many relevant fields—and not necessarily only in astronomy—but their integration to SETI's approach has been slow in coming. Yet the means are currently in place to substantially increase the odds of finding ET through the development of an expanded search strategy that reflects this new knowledge.
4.1. Searching for ourselves
Part of this expanded vision starts by acknowledging that so far, in our quest to find ET, we have only been searching for other versions of ourselves, making the odds of success possibly more daunting than already dictated by nature. While the first variables of the equation are astronomical, observable, measurable, and most likely universal, as we move to the right, they become local, and the transition from life to intelligence to civilization and technology does not even characterize the average life-form on Earth. It characterizes us humans, a statistical outlier on our own planet.
Searching for other versions of ourselves is not unique to SETI. This is equally true of astrobiology, and it makes perfect sense as a starting point. After all, this is the only model of life we know, and a model that has been proven on our planet. Such an exploration strategy is further vindicated by the fact that the basic elements of life as we know it (CHONPS) are common in the Universe (Bada et al. , 1994 ; Dyson, 1999 ; Seckbach et al. , 2004 ; Brack, 2005 ; Mandell, 2008 ; Gibb, 2013 ), and habitable environments for life as we know them seem plentiful. Moreover, the Kepler mission and ground-based data suggest that there could be as many as 40 billion Earth-like planets in our galaxy alone (Petigura et al. , 2013 ), 25% of them around Sun-like stars. Other models suggest that there could be up to 700 trillion planets in the Universe, but the vast majority would be far older than Earth (Zackrisson et al. , 2016 ). Using a Biological Complexity Index, Irwin et al. ( 2014 ) proposed the existence of ∼100 million planets in the Milky Way where complex life could have evolved. However, as they note, complex life does not necessarily mean technologically advanced life.
Although encouraging, large numbers do not tell the whole story. Zackrisson's study suggests that these worlds are likely to be very different from our planet, while other studies take the extreme position that Earth could be unique (Ward and Brownlee, 2003 ). In addition to the possible issues of age and composition, fundamental questions have now emerged from space, planetary, and earth sciences that directly bear on the question of planetary habitability as we know it, life beyond Earth, and its potential evolutionary tracks. These questions include the following:
Is there a generational aspect to the rise of life in the Universe depending on elements delivered by dying stars ( e.g., Cowan and Sneden, 2006 )? Did the configuration of the Solar System play a role in the successful development and survival of life on Earth ( e.g., star, planets, their composition, size, and position)? In particular, did planets the size of Jupiter and Saturn protect life on Earth by attracting most cosmic debris (Horner et al. , 2013 ) or, alternatively, foster the development of life on Earth by helping the delivery of volatile materials from the outer Solar System needed for life to form (Grazier, 2016 )? Did our moon play a critical role in the rise of biology by stabilizing Earth's climate (Laskar et al. , 1993 ; Lissauer et al. , 2011 )? How did Earth transition from prebiotic chemistry to life (Lazcano and Miller, 1996 ; Pascal et al. , 2006 ; Fitz et al. , 2007 ; McCollom, 2013 ; Ruiz-Mirazo et al. , 2013 ; Imari Walker, 2014 ; Walker, 2014 )? Does environmental stability foster or inhibit life's inception, survival, and evolution; or does it take a mix of stability and chaos to stimulate biochemistry and biodiversity (Rosenfeld, 2011 )? Do plate tectonics and oceans play a critical role in prebiotic and biotic processes (Spohn, 1991 ; Heller et al. , 2011 ; Stern, 2015 )—and are they critical individually or collectively (Kasting, 1993 ; Martin et al. , 2008 ; Golding and Glikson, 2011 ; Lyons et al. , 2014 )? The most basic of all these questions remains today without an answer: What is life?
These are just a few examples, but the answer to each question has the power to deeply shape the vision of what the potential for an “Earth-like” planet to harbor life really means, and will affect the probability for such a planet to develop advanced and technological life.
4.2. Evolutionary and systemic approach to N
Progressing to the right of the Drake equation, astronomy and biology are linked through two concepts: habitable zone and environmental habitability ( e.g. , Cockell et al. , 2016 ). Both notions are mutable in space and time, the latter even more so than the former; and on Earth, climate change, extreme terrestrial environments, and genomics demonstrate how fast environmental changes can happen and how much the evolution of life and environment are intertwined (Golding and Glikson, 2011 ). While the initial astronomical and physicochemical conditions may drive the type of prebiotic chemistry possible on a planet, life, as demonstrated on Earth, becomes a primary player in shaping its environment as soon as it takes hold ( e.g. , atmosphere, landscape, mineralogy). This is the fundamental concept of coevolution of life and environment ( e.g., Watson, 1999 ; Kooijman, 2004 ; Dietrich et al. , 2006 ; Knoll, 2009 ; Grenfell et al. , 2010 ; Kolb, 2014 ). This coevolution will dictate the uniqueness of each planetary experiment (Irwin and Schulze-Makuch, 2001 ; Schulze-Makuch et al. , 2013 ) and will do so not only when (or if) life reaches the stage of technological advancement. It will start from the very first moment, as it did on Earth.
Earth's biosphere, and all events that led to us, and the way we perceive the environment and the Universe are the result of over 4 billion years of reciprocal influences between environmental and biological processes (Fabbro et al. , 2015 ; Houri-Ze'evi et al. , 2016 ). They are the result of evolutionary bottlenecks induced by geological, climatic, and cosmic events (Lynch and Lande, 1993 ; Bürger and Lynch, 1995 ; Bijlsma et al. , 2013 ). An asteroid impacting instead of missing Earth would completely alter the course of evolution at any time.
Ultimately, biological evolution on Earth has been partially dictated by somewhat predictable cycles and events (astronomical, climate) but drastically more so by stochastic events, both geological and biological in nature ( Fig. 2 ) (Pigliucci and Müller, 2010 ), by when and where they occurred and at what stage of evolution they happened (Gould, 1998 ; Raup, 1999 ; Schulze-Makuch and Irwin, 2001 ; Schulze-Makuch et al. , 2013 ; Keller and Kerr, 2014 ; Lehman and Miikkulainen, 2015 ). It follows that, even considering panspermia and planetary exchange as primary seeding mechanisms ( e.g. , Arrhenius, 1907 ; Arrhenius and Mojzsis, 1996 ; Melosh, 1996 ; Burchell et al. , 2004 ; Napier, 2004 ; Gladman et al. , 2005 ; Owen, 2008 ; Worth et al. , 2013 ), two planets with absolutely similar environments and receiving absolutely similar seeding material may have two very distinct biological destinies depending on when extinctions and evolutionary bottlenecks take place, and whether these events have transient or long-lasting effects on the environment. Stochastic events will give each planet a unique fingerprint. If life arises through abiogenesis of simple organic compounds (Des Marais, 1999 ; Popa, 2004 ; Shapiro, 2006 ; Pross, 2012 ; Pross and Pascal, 2013 ), this fingerprinting will be even more uniquely connected to its planet of origin, and cosmic chances are that both exogenic and endogenic processes are acting together in proportions specific to each planetary system.

Coevolution of life and environment. Environmental perception and neural systems of the species living on Earth are intimately linked to the coevolution of life and environment, a process that has been subjected to random probability events all along its continuum. This process started with accretion and the specific elements that contributed to planetary formation. As prebiotic chemistry transitioned to life, the period of heavy bombardment continued to spatially randomly deliver material to Earth, contributing equally to the destruction of a nascent life and the development of a new one. Since life took hold, stochastic events have continued in the physical world as environmental and cosmic catastrophes, and in the biological world as adaptive evolution and epigenetic changes. While the notions of habitable zone and environmental habitability are critical in trying to predict whether a planet could host life as we know it, the very random nature of the coevolution of life and environment renders each planet and the life it may bear a unique experiment, which questions the anthropocentric principle underlying the Drake equation.
Moreover, if Earth is any indication of universal planetary laws of evolution, nature produces simple systems in overwhelming numbers compared to complex systems (Mandelbrot, 1982 ). Statistically, microbial life still dominates today, and for 70% of Earth's history was the only form of life on our planet (Margulis, 1996 ; Nealson and Conrad, 1999 ; Wolf et al. , 2002 ). Taking inventory, global species richness estimates suggest that up to 10 million species of eukaryotes and up to 100 million to a billion prokaryote species exist today on Earth (Colwell and Coddington, 1994 ; Gaston, 2000 ; Colwell, 2009 ; Mora et al. , 2011 ; McGinley, 2014 ), while the most recent studies model up to one trillion species (Locey and Lennon, 2016 ). In this inventory of all terrestrial life, through competition, only humans have reached technology. However, this statistical snapshot alone does not provide an accurate estimate of a realistic ratio, as it does not reflect the (unknown) total number of species produced by our planet over time. Those include evolutionary dead ends and species that went extinct as a result of climatic or catastrophic events but still played their part in shaping the global biosphere from which we, the human species, emerged. It does not account for the shadow biosphere hypothesis, either (Cleland and Copley, 2005 ; Davies et al. , 2009 ).
A systemic approach to N shows that it took 4 billion years of symbioses, competition, biodiversification, and the development of all species that did—and did not—survive for us to be here. Some key evolutionary leaps have yet to be completely understood. Only very recently, the common ancestral gene that may have enabled the evolution of complex life over a billion years ago was identified. Without this gene, life on Earth might not have evolved beyond the stage of slime (Lai et al. , 2016 ). We also have yet to understand what led one species of apes to separate itself from the others just a few million years ago (Arsuaga, 2010 ). Understanding these transformative processes may bear considerable weight on N . It is certainly not necessary to wait for all the answers to start searching for ET, but we must acknowledge that these questions exist, and develop search strategies adapted to the complexity they underscore in order to augment the chances of success.
We are, indeed, the product of local astronomical and planetary factors. However, it would be unreasonable to suggest that similar evolutionary convergence never happened with seemingly so many planets already discovered in the small spatiotemporal window of the Kepler telescope. Somewhere out there, based solely on numbers and probabilities, life may have evolved to bear some resemblance to us—if only fortuitously. It might interact with its planetary environment as we do, and evolve to produce biological forms with logical minds presenting similarities to us who may be willing to communicate in ways we can understand. However, the numbers are unlikely to be in the billions or even the millions in our galaxy. There may be just a handful scattered across vast distances and time.
Taking life's evolution on Earth as a guide, there is likely a universal probabilistic law of evolutionary convergence that is inversely proportional to life's complexity; that is, the simpler life is, the greater chances are that similar life-forms will be abundant throughout the Universe. The more complex life is, the more rare convergence is likely to be. Complexity in life-forms is an integration of temporal evolution and probabilistic events. The longer life is around, the greater chance it has to adapt through regular cycles and, at any given time, to mutate through stochastic events. Looking back at ourselves, it took 70% of Earth's time in the habitable zone and an incredible amount of “chance and necessity” (Monod, 1972 ) for one species in a complex tree of life to reach civilization and technology.
The longer evolution takes, the greater the chances are that species will be wiped out and ecosystems profoundly transformed ( e.g. , Alvarez and Asaro, 1990 ), but with the rise of technology, some of the endogenic and exogenic risks to life can also be offset ( e.g. , asteroid monitoring, Yeomans, 2013 ). Conversely, human evolution shows that technology brings its own sets of risks: the natural dynamics are upset (Holocene extinction: Barnosky et al. , 2011 ), the environment modified (Anthropocene: Grinspoon, 2012 ; Waters et al. , 2016 ), and the terms of the coevolution of life and environment that led to the rise of the dominant species deeply altered.
At this point in time, humans have generated an environmental disequilibrium that reverberates across the biosphere globally and endangers the conditions of planetary habitability that were favorable to its emergence. The notion of self-engineered destruction is certainly present in the last factor of the Drake equation. L reflects on how long a technological civilization might be willing and able to communicate. More than duration, this factor focuses on the odds of detecting a signal; that is, the longer an alien civilization broadcasts its presence, the more chances we have to detect it. Assuming the anthropocentric view of a technological civilization presenting similarities with ours, willingness to communicate may depend on a host of reasons ( e.g. , political, scientific, technological, philosophical, religious, and social). How long such a civilization would continue to communicate is a more complex issue. Duration can relate to a civilization's ability to avoid self-inflicted—or other—destruction, scientific advances, and interest. It could also relate to a cosmologic perspective we have not yet reached, including a sense of place and responsibility as a member of a universal community ( e.g. , the Fermi paradox).
As expressed, L examines for how long such a civilization would broadcast a signal in ways we can detect, which are primarily focused on radio and optical astronomy. We could assume, however, that a technological civilization may communicate or broadcast in ways so advanced compared to ours that we simply cannot imagine what they are and are thus unable to detect its signals. Or this civilization may have long since disappeared. These scenarios are implicitly present in the current definition of L . However, there is also an evolutionary dimension to this factor that transcends the existing formulation.
5. Expanding the N Horizon
The evolutionary pathways that lead to complex life on Earth strongly suggest that advanced life as we know it may be rare in the Universe and unlikely to be in a state of advancement that is temporally synchronous with us. However, that does not mean that other types of advanced intelligences are as rare. Limiting our search to something we know and can de facto comprehend is, probabilistically, a constraining proposition, one that leaves no room for an epistemological and scientific foundation to explore alternate hypotheses. To find ET, we must expand our minds beyond a deeply rooted Earth-centric perspective and reevaluate concepts that are taken for granted .
5.1. Becoming aliens
Rather than constraining the search, SETI efforts must involve the most expansive exploration tool kit possible. If we unbind our minds, it should not matter whether ET looks or thinks like us, has a logic that makes any sense to us, or uses familiar technology for interstellar communication. ET is likely to be very different from us and completely alien to our evolutionary processes and thought processes, which may be deeply connected (see Section 5.2.3 ). Ultimately, to find aliens, we must become the aliens and understand the many ways they could manifest themselves in their environment and communicate their presence.
Such an intellectual framework not only moves the Drake equation forward toward the existence of drastically different probabilistic civilizations, it also brings us to consider alternate evolutionary pathways, including life as we do not know it and do not yet understand. Further, such a framework allows us to look at evolutionary pathways in our own biosphere and question the emergence of complex, intelligent life with a different set of eyes. For that to happen, we must conceptualize something we do not know, which can be approached in a number of different ways. One is by trying to access unknown concepts and archetypes that are literally alien to us ( i.e. , not part of our own evolutionary heritage) through imagination and discourse. This is what science fiction attempts to do in its depictions of alien worlds and civilizations. Not surprisingly, this process results in more or less elaborate versions of ourselves, since these representations are generated by neural systems wired to our own planetary environment. To conceptualize a different type of life, we have to step out of our brains.
5.2. Universal heritage
A path to finding life we do not know requires us to identify a common universal heritage , one that includes signatures and signals that can be recognized across different evolutionary tracks and across space and time. Research in this direction was initiated by the Communication with Extraterrestrial Intelligence (CETI) and the Arecibo message (Sagan, 1975 ; Vakoch, 2011a ). Advances in astrobiology, life science, and cognitive science are bringing new perspectives and depth to that concept. Some of them are already being explored, while others belong to disciplines that have yet to be involved with SETI and represent a currently untapped potential.
5.2.1. Distant biosignatures
In searching for life in the Solar System and on exoplanets, astrobiology is using an approach based on the concept of “universal heritage” but more narrowly focused on life as we know it (Seckbach, 2006 ). For instance, water and carbon are driving search strategies; the formation, preservation potential, and detection methods of biosignatures that could be similar to Earth's are being investigated for the exploration of extinct and extant life on Mars, Europa, and exoplanets. In situ biosignatures are physicochemical, geological, morphological, and mineralogical in nature (Summons et al. , 2011 ). Remotely detectable biosignatures include gases in planetary atmospheres (Pilcher, 2004 ; Segura et al. , 2005 ; Domagal-Goldman et al. , 2011 ; O'Malley-James et al. , 2014 ; Seager, 2014 ; Krissansen-Totton et al. , 2016 ). Given an environmental analogy, it is conceivable that alien biospheres presenting similarities with ours may have generated and left traces we could recognize. However, none of these signatures are convincingly unambiguous evidence of life's presence as both biological and abiotic processes alike can produce them (Schwieterman et al. , 2016 ). Therefore, it might be difficult to use them as universal markers of life as we know it, let alone for life we do not know.
Astrobiology and Earth sciences show that the systemic disequilibrium generated by the presence of life could be a promising candidate as a universal marker of life (Schwartzman, 2004 ; Branscomb and Russell, 2013 , Russell et al. , 2013 ). Biological activity, from microorganisms to humans, utilizes and modifies its environment, producing traces (physical, chemical, isotopic) not otherwise found in nature in the absence of life. As long as we search for biology with a physicochemical support, such disequilibrium will be generated and measurable across species and planets—although we will have to start by learning how to untangle it from the planetary background. The argument can also be made that some technological civilizations, or civilizations beyond technology, may be so advanced that they have returned to equilibrium and generate living conditions that do not betray their physical presence anymore—or they purposely hide their presence (Kipping and Teachey, 2016 ). In such instances, they will remain stealth to this search method. Planetary biosignatures reflecting the presence of a biosphere will still be visible, but traces of advanced beings on that planet may no longer be detectable.
While this marker falls short of helping the detection of environmentally stealthy civilizations, it can help find those that have not yet reached that stage. It also offers a universal vision to life detection that goes beyond life we know, thereby vastly expanding the statistical planetary pool that can be probed today. Further, and critical for SETI, this approach does not depend on the willingness of aliens to communicate. The coevolution of life and environment creates a physicochemical overprint that, regardless of the stage of life's development, will betray its presence. Learning the range of signatures produced by such disequilibrium from local to global scale should, therefore, become a priority in the development of techniques to search for life of all types, sizes, and advancement stages. Even though each candidate signature might not be produced by an advanced civilization, this method will identify more potential targets for SETI. The current limitation is both the spectral resolution of instruments and the distance of possible targets, but this method should become a critical investigation strategy to survey our galactic neighborhood (Seager and Deming, 2010 ; Deroo et al. , 2012 ; Beichman et al. , 2014 ; Burrows, 2014 ; Seager, 2014 ; Crossfield, 2015 ). A search for systemic disequilibria at a planetary scale is currently one of the most promising methods for detecting life beyond Earth. Taken alone, it might not be enough to inform us of the stages of life's development, but in the coming years, analog missions to our own atmosphere might teach us how to identify technosignatures.
5.2.2. Communication
Ultimately, the evidence SETI is looking for is an unambiguous signal from ET ( e.g. , Tarter, 1983 ; Shostak, 1998 ). To intercept alien signals, SETI has traditionally relied on radio astronomy for passive listening, and more recently on optical methods, and while the idea of active SETI—also known as Messaging Extraterrestrial Intelligence (METI)—remains a subject of controversy (Atri et al. , 2011 ; Vakoch, 2011b ; Vakoch and Matessa, 2011 ; Neal, 2014 ), communication and contact are very much at the core of the SETI concept. But what are we searching for and listening to?
In theory, SETI is searching for coded messages sent through controlled laser emissions or radio signals containing patterns that cannot be readily explained by known natural phenomena, ( e.g., Tarter, 2001b ; Vakoch, 2011a ; Forgan, 2014 ; Wheeler, 2014 ; Heller and Pudritz, 2016 ). Some research areas focus on the type of messages that should be broadcast if/when METI becomes active and on the content of these messages (Eliott, 2011b , 2011c ; Ollongren and Vakoch, 2011 ; Vakoch, 2011b , 2011c ; Musso, 2012 ). Since physical and mathematical principles are universal, they can be considered part of a universal heritage and a good starting point for composing messages (Ilhan and Linscott, 2011 ; Azua-Bustos and Vega-Martinez, 2013 ). Symbols, geometry, visual imageries, music, and sounds have been used in the past as part of the Pioneer and Voyager messages (Rose and Wright, 2004 ; Shostak, 2011b ). They continue to be investigated with the understanding that some might not be universal but biased toward senses humans have that ET might not have developed—or developed differently.
Since the ultimate goal of SETI is to identify alien signals, it should follow that understanding the origin, structures, and various forms and supports of communication (natural and artificial) is a fundamental step in the development and success of the search (Witzany, 2015 ). Research in marine and land species has already revealed common patterns that may be clues to laws of communication in the languages of some of Earth's intelligent species (Doyle et al. , 2011 ). This research area shows important promises, and these patterns might help us identify and decrypt alien messages coming our way one day. However, an important question—whose answer may lie in genomics and neuroscience—is whether these shared patterns are Earth-specific and the result of common environmental context and evolution, or actually universal in nature.
Further, perception and communication are severely constrained by sensory range ( e.g. , wavelengths, amplitude, reach) and by words in humans. The complexity and subtlety of a thought or a feeling can often be lost when it reaches verbal formulation even for members of the same cultural and linguistic groups. This limitation will be more acute between interplanetary species that evolved on planets with potentially different physicochemical conditions, environmental contexts, and mixings.
5.2.3. Environmental perception—alien neural systems
Most advanced alien species will likely have developed forms of communication completely unrecognizable to us. It can be hypothesized, however, that life anywhere must gather information about its environment for purposes of development, adaptation, survival, and evolution (Geary, 2005 ; Roth, 2013 ; Krubitzer, 2014 ; Schulkin, 2014 ). For terrestrial species, information is collected through senses, including many shared across species, while others are species-specific ( e.g. , echolocation, electroreception, magnetoreception). The senses are transducers from the environment to the brain where information is being interpreted—or through homolog systems for species without a nervous system (Strausfeld and Hirth, 2015 ). Alien life may need equivalent systems to process and integrate internal and external information. This is how ET will perceive its environment ( e.g. , Nolfi and Parisi, 1996 ; Pfeifer and Gómez, 2006 ) and communicate information about it in the messages it encodes, and for us, this is the key to unlock how we will decode them.
In that respect, cognitive and mathematical sciences ( e.g. , neuroscience, computational modeling, machine learning, other) may be the disciplines that can deliver the most transformative advances to SETI at this point in its history. By understanding how perception, concepts, and archetypes are being formed and formulated (Perlovsky, 2006 ) and how information is being stored and processed through neural or homolog systems, SETI could start exploring the source point of communication, where the elemental components of perceiving, interacting with, and communicating our understanding of planetary environments, our place in the Universe, and the Universe itself are being generated.
The structure and content of any alien message will have a local overtone to these elemental components driven by where (space and environment) and when (time) a civilization appeared in the Universe. However, on the cosmic scale, physics, chemistry, and biology are constrained by universal parameters that link all life —as diverse as it may be. These parameters are our common heritage and the letters of our universal alphabet. Life sciences, and cognitive and mathematical sciences, provide a fundamental and mandatory path for SETI to understand this universal alphabet. They are already used in the investigation of possible universal languages (Vakoch, 2011a ). However, in order to decode or encode messages from and to ET, a broader approach than the existing one is needed, one that considers the many possible combinations of evolutionary paths—not just those most familiar to us.
What SETI needs is a comprehensive integration of the concept of coevolution of life and environment in its search strategy, and the essential role it may play in the structure of ET's environmental sensing systems. That coevolution may fundamentally affect how aliens perceive their world, intellectualize it, and communicate it. As our knowledge of exoplanetary environments broadens, we are becoming better prepared to conceptualize the potential biochemistries and types of life these environments could support. We must use this background to develop probabilistic simulations of alien neural—or homolog sensing—systems. This will allow us to see the environment and the Universe as ET may perceive them, and not as we hope it does with our own set of senses. Without that basic understanding, SETI will essentially continue to search blind.
Such a research avenue can now find a robust scientific foundation in the advances made by evolutionary biology in innovative areas such as evolvability, evolutionary developmental biology, epigenomics, and system biology—regrouped under the term of extended synthesis (Pringle, 1951 ; Smith and Cribbs, 1994 ; Ziman, 2000 ; Wagner, 2007 ; Wagner et al. , 2007 ; Pigliucci and Müller, 2010 ; Wagner and Draghi, 2010 ; Schrey et al. , 2012 ; Jerison and Jerison, 2013 ; Valiant, 2013 ; Badyaev and Walsh, 2014 ; Watson and Szathmáry, 2015 ). This body of work strongly suggests that life, intelligence, and perception are shaped by, and designed to respond to, a planetary environment; this has powerful implications for SETI's anthropocentric principle and implications for its search strategy that cannot be ignored.
Today, vast amounts of data and computational capacity are at hand (Graham et al. , 2005 ; Heinecke et al. , 2015 ), allowing us to start on the path of modeling probabilistic evolutionary tracks using known exoplanetary environments, Solar System exploration, the exploration of extreme environments, and the computational tools of life, mathematical, and cognitive sciences and neuroscience ( e.g. , Wolpaw and Winter Wolpaw, 2012 ; Rao et al. , 2014 ). Results may lead us to first identify, then design, novel types of communication methods ( e.g. , message content and support), instruments, and technologies to search for ET.
Further, while evolutionary biology holds critical clues for our understanding of advanced intelligent life in the Universe, the implications of its applications to modeling alien life are much broader than SETI alone. As new theories mature through validation and falsification, they will help us interrogate more deeply the evolutionary continuum of intelligence on Earth from simple to complex life, a process highly relevant to astrobiology.
Ultimately, though, while understanding evolution, perception, and communication is absolutely essential to SETI, it will not be impactful if this knowledge remains compartmentalized. Data generated by each of these disciplines must be fused together and transformed into reasoned search strategies and experimental protocols. They must result in the identification and articulation of science questions and hypotheses that open pathways forward to theories and quantifiable metrics and milestones (see also Fig. 1 ).
6. An Integrated Vision Moving Forward
So far, a number of perspectives have been offered to explain why radio receivers have remained silent for the past 50 years, including the Fermi paradox and the Zoo hypothesis (Ball, 1973 ). More recently, two new scenarios have been added: ET is dead, based on the argument for a Gaian bottleneck (Chopra and Lineweaver, 2016 ), and the opposite view, that we are the oldest civilization in the Galaxy (Sasselov, 2012 ). It may be that the answer is much simpler and humbling. The fact is that we embarked on this journey only a few decades ago and have applied to it very limited tools and detection strategies. Telescopes have become bigger, arrays larger, resolution better, but the technology and exploration strategies behind SETI have remained basically unchanged. In reality, we have yet to articulate core questions to place SETI in its proper, systemic, and evolutionary context.
In its current scientific and technological form, SETI can be likened to sampling the cosmic ocean with a plankton net, where the size of the antennas and their number are analogous to the mesh size. Refining the mesh size by adding antennas or making them bigger will not make the tool more effective compared to the breadth of exploration at hand. Further, this tool is only focused toward testing one very specific, largely anthropocentric, hypothesis about extraterrestrial intelligence (ETI), when data increasingly suggest that there are probably as many distinct life-forms and intelligences as habitable planetary environments in the Universe. While the historical view of ETI is a valid hypothesis that must be tested, it is one among many, and we should proceed with it acknowledging that the chances of a rendezvous somewhere in time, and space, with that particular alien are remote.
When the odds are small and the object of a search unknown, the rule of thumb is to cast as large a net as possible to increase the chances of success rather than the opposite. In this case, a larger net is not solely a larger set of antennas or more abundant resources focused on one technology or one experiment (Billings, 2015 ). For SETI, it is critical to fully embrace the multidisciplinary approach that was scripted 50 years ago in the Drake equation and create a well-stocked and diverse tool kit to explore a vision that serves as an umbrella under which many disciplines will synergistically contribute advances to expand the search for alien life beyond the anthropocentric principle.
Some bridges are already in place and being investigated, such as the clear connections between the search for ET and exoplanet exploration ( e.g. , Tarter, 2007 ). SETI uses the Kepler catalog and ground-based telescope databases to target high-priority candidates. Prioritization criteria are currently limited to parameters for life as we know it ( e.g. , exoplanets in the habitable zone of their parent stars; unexplained signals from known exoplanetary systems, see Harp et al. , 2015 ; Schuetz et al. , 2015 ; Abeysekara et al. , 2016 ). Those criteria will be refined over time as universal markers are being identified and as we learn more about possible exotic biochemistries ( e.g. , McKay, 2004 ; Ward and Benner, 2007 ; Davila and McKay, 2014 ), but the connection is established and will only grow stronger.
In addition to this, new research pathways bear significant promises for a more universal SETI reach, such as ET and systemic planetary disequilibria, the coevolution of life and environment, and neural systems and their role in our perception of the Universe, our interaction with it and how we communicate about it—to name but a few. Figure 3 summarizes a path to achieving this integrated and synergistic vision. It shows a notional network between disciplines with bridges and research avenues connecting together space, planetary, life, geosciences, astrobiology, cognitive, and mathematical sciences. Others will be added as knowledge progresses. It is a dynamical vision forward, built on a connectivity network that represents an expanded version of the Drake equation, one that integrates all the historical factors now broken down into measurable terms.

Connectivity network between disciplines showing the bridges and research avenues that link together space, planetary, and life sciences, geosciences, astrobiology, and cognitive and mathematical sciences. This representation is an expanded version of the Drake equation. It integrates all the historical factors now broken down in measurable terms and expanded to include the search for life we do not know using universal markers, and the disciplines, fields, and methods that will allow us to quantify them.
This vision expands the search to life as we do not know it using universal markers and the disciplines, fields, and methods that will allow us to quantify them. Points of intersection are the knowledge that will be contributed to SETI by integrating these various disciplines. This contribution will be provided in five fundamental areas: (1) scientific rationale (theories, hypotheses); (2) experimental design (methods, protocols, and metrics); (3) communication (universal markers, signals, instruments, systems, technologies); (4) target identification; and (5) missions that will allow SETI to implement its own vision. These sciences are already collecting vast data sets that can now be interrogated in ways we could not have imagined only a few years ago. Bioneural computing, communication theory, machine learning, neural coding, neural network and deep learning, data mining, and big data analysis are the building blocks of the connecting bridges that SETI must build as a prerequisite to a universal exploration of ET versus the current anthropocentric search.

7. Conclusion
Now is the time for SETI to develop a roadmap and long-term vision that includes the search for life as we do not know it. New tools are available that can enable this approach and help us decipher the evolutionary and probabilistic nature of advanced alien life. These tools are found in astronomy and astrophysics but also in the biological, geological, cognitive, mathematical, and computer sciences, among others. All of them must be deployed when thinking about who, what, and where ET could be, and how it might communicate.
To find ET, we will have to turn inward (evolution, perception, and communication) and outward (planetary environments) and challenge our anthropocentric assumptions. Through consilience, we can build a systemic and connective vision of intelligent life in the Universe. To be achieved, this vision requires the exploitation of multidisciplinary synergies and an intellectual framework with a clear agenda and milestones. A similar intellectual and logistical structure was successfully established 20 years ago by NASA with the creation of the NASA Astrobiology Institute (NAI) for the understanding of life on Earth and beyond. However, past and present astrobiology roadmaps have not put substantial emphasis on alien intelligence, communication, or technology.
In the coming months, the SETI Institute will be initiating efforts in this direction, and will invite the United States and international research communities to contribute to the drafting of a new scientific roadmap for SETI. We will explore resources for the development of a virtual institute and an intellectual framework for multidisciplinary projects specifically focused on the advancement of knowledge on ETI. Complementary to, and different from, the astrobiology roadmap, this vision will bridge the NAI roadmap, augment it, and go beyond it.
Its primary goal will be to “Understand how intelligent life interacts with its environment and communicates.”
This will be explored through three main questions:
Question 1: How abundant and diverse is intelligent life in the Universe? With this question, SETI will synergistically use data from astrobiology, biological sciences, space and planetary science and exploration, and geoscience to quantitatively and qualitatively characterize the potential abundance and diversity of intelligent life in the Universe. Its spatiotemporal distribution will be approached using cosmological models of the physicochemical evolution of the Universe and inferred viable biochemistries.
Question 2: How does intelligent life communicate? Cognitive sciences, neuroscience, communication theory, mathematical sciences, bioneural computing, data mining, and big data analysis, among other disciplines, will explore communication in intelligent terrestrial species. They will use physicochemical and biochemical models of known exoplanetary environments to generate and map probabilistic neural and homolog systems, and infer the resulting range of viable alien sensing systems and the perception of the Universe they confer. Spatiotemporal distribution models will be inferred from Question 1–related investigations.
Question 3: How can we detect intelligent life? Exploration strategies, instruments, experimental protocols, technologies, and messaging (content and support) will be designed using the results, data, and databases of research conducted under Questions 1 and 2.
Ultimately, SETI's vision should no longer be constrained by whether ET has technology, resembles us, or thinks like us. The approach presented here will make these attributes less relevant, which will vastly expand the potential sampling pool and search methods, ultimately increasing the odds of detection.
Advanced, intelligent life beyond Earth is most likely plentiful, but we have not yet opened ourselves to the full potential of its diversity. With the vision presented here, we offer a unified and universal approach for the search for extraterrestrial life—one that is measurable and searches for ET at the crossroads of scientific and technological innovation, and imagination.
Abbreviations Used
Acknowledgments.
I am particularly grateful to those who, through conversations, constructive criticism, suggestions, comments, and reviews at various stages of development have helped me articulate this perspective. Special thanks to Bill Diamond, David Darling, Margaret Race, Mark Showalter, and Jill Tarter for their inputs. Also thank you to Maggie Turnbull and Laurance Doyle for sharing thoughts over the past few months.
Author Disclosure Statement
The author declares no conflict of interest.
1 SETI in the context of this article means SETI research (as it is performed around the world) and should not be confused with the SETI Institute. In the cases where mention is made of the SETI Institute, this is clearly spelled out.
- Abeysekara A.U., Archambault S., Archer A., Bendow W., Bird R., Buchovecky M., Buckley J.H., Byrum K., Cardenzana J.V., Cerruti M., Chen X., Christiansen J.L., Ciupik L., Cui W., Dickinson H.J., Eisch J.D., Errando M., Falcone A., Furniss A., Gillanders G.H., Griffin S., Grube J., Gyuk G., Hütten M., Håkansson N., Hanna D., Holder J., Humensky T.B., Johnson C.A., Kaaret P., Kar P., Kelley-Hoskins N., Kertzman M., Kieda D., Krause M., Krennrich F., Kumar S., Lang M.J., Lin T.T.Y., Maier G., McArthur S., McCann A., Meagher K., Moriarty P., Mukherjee R., Nieto D., O'Brien S., O'Faoláin de Bhróithe A., Ong R.A., Otte A.N., Park N., Perkins J.S., Petrashyk A., Pohl M., Popkow A., Pueschel E., Quinn J., Ragan K., Ratliff G., Reynolds P.T., Richards G.T., Roache E., Santander M., Sembroski G.H., Shahinyan K., Staszak D., Telezhinsky I., Tucci J.V., Tyler J., Vincent S., Waleky S.P., Weiner O.M., Weinstein A., Williams D.A., and Zitzer B. (2016) A search for brief optical flashes associated with the SETI target KIC 8462852 . Astrophys J 818 , doi: 10.3847/2041-8205/818/2/L33 [ CrossRef ] [ Google Scholar ]
- Almár I. and Tarter J. (2011) The discovery of ETI as a high-consequence, low-probability event . Acta Astronaut 68 :358–361 [ Google Scholar ]
- Alvarez W. and Asaro F. (1990) An extraterrestrial impact: accumulating evidence suggests an asteroid or comet caused the Cretaceous extinction . Sci Am 263 :78–84 [ PubMed ] [ Google Scholar ]
- Ansbro E. (2001) New OSETI observatory to search for interstellar probes . Proc SPIE 4273, doi: 10.1117/12.435370 [ CrossRef ] [ Google Scholar ]
- Arrhenius G. and Mojzsis S. (1996) Extraterrestrial life: life on Mars—then and now . Curr Biol 6 :1213–1216 [ PubMed ] [ Google Scholar ]
- Arrhenius S. (1907) Panspermy: the transmission of life from star to star . Sci Am 96 :196 [ Google Scholar ]
- Arsuaga J.L. (2010) Terrestrial apes and phylogenetic trees . Proc Natl Acad Sci USA 107 :8910–8918 [ PMC free article ] [ PubMed ] [ Google Scholar ]
- Atri D., DeMarines J., and Haqq-Misra J. (2011) A protocol for messaging to extraterrestrial intelligence . Space Policy 27 :165–169 [ Google Scholar ]
- Azua-Bustos A. and Vega-Martinez C. (2013) The potential for detecting “life as we don't know it” by fractal complexity analysis . International Journal of Astrobiology 12 :314–320 [ Google Scholar ]
- Bada J.L., Bighamm C., and Miller S.L. (1994) Impact melting of frozen oceans on the early Earth—implications for the origin of life . Proc Natl Acad Sci USA 91 :1248–1250 [ PMC free article ] [ PubMed ] [ Google Scholar ]
- Badyaev A.V. and Walsh J.B. (2014) Epigenetic processes and genetic architecture . In Quantitative Genetics in the Wild , edited by Charmantier W.A., Garant D., and Kruuk L.E.B., Oxford University Press, Oxford, UK, pp 177–189 [ Google Scholar ]
- Ball J.A. (1973) The zoo hypothesis . Icarus 19 :347–349 [ Google Scholar ]
- Barnosky A.D., Matzke N., Tomiya S., Wogan G.O.U., Swartz B., Quental T.B., Marshall C., McGuire J.L., Lindsey E.L., Maguire K.C., Mersey B., and Ferrer E.A. (2011) Has the Earth's sixth mass extinction already arrived? Nature 471 :51–57 [ PubMed ] [ Google Scholar ]
- Batalha N.M. (2014) Exploring exoplanet populations with NASA's Kepler mission . Proc Natl Acad Sci USA 111 :12647–12654 [ PMC free article ] [ PubMed ] [ Google Scholar ]
- Beichman C., Benneke B., Knutson H., Smith R. D.ressing C., Latham D., Deming D., Lunine J., Lagage P.-O., Sozzetti A., Sing D., Kempton E., Ricker G., Bean J., Kreiberg L., Bouwman J., Crossfield I., Christiansen J., Ciardi D., Fortney J., Albert L., Doyon R., Rieke M., Rieke G., Clampin M., Greenhouse M., Goudfrooij P., Hines D., Keyes T., Lee J., McCullough P., Robberto M., Stansberry J., Valenti J., Deroo P.D., Mandell A., Ressler M.E., Shporer A., Swain M., Vasisht G., Carey S., Krick J., Birkmann S., Ferruit P., Giardino G., Greene T., and Howell S. (2014) Observations of transiting exoplanets with the James Webb Space Telescope (JWST) . Publ Astron Soc Pac 126 :1134–1173 [ Google Scholar ]
- Bijlsma R., Westerhof M.D.D., Roekx L.P., and Pen I. (2013) Dynamics of genetic rescue in inbred Drosophila melanogaster populations . Conserv Genet 111 :449–462 [ Google Scholar ]
- Billingham J., Edelson R., Gulkis S., Olsen E.T., Oliver B.M., Tarter J.C., and Seeger C.L. (1980) A search strategy for SETI [paper #IAA-80-57] . In 9 th International CETI Review Meeting of the International Academy of Astronautics, Tokyo, Japan [ Google Scholar ]
- Billings L. (2015, July 20) Stephen Hawking and Yuri Milner announce $100M initiative to seek ET . Sci Am [ Google Scholar ]
- Borucki W.J., Koch D., Basri G., Batalha N., Brown T., Caldwell D., Caldwell J., Christensen-Dalsgaard J., Cochran W.D., DeVore E., Dunham E.W., Dupree A.K., Gautier T.N., Geary J.C., Gilliland R., Gould R., Howell S.B., Jenkins J., Kondo Y., Latham D.W., Marcy G.W., Mejbom S., Kjeldsen H., Lissauer J.J., Monet D.G., Morrison D., Sasselov D., Tarter J., Boss A., Brownlee D., Owen T., Buzasi D., Charbonneau D., Doyle L., Fortney J., Ford E.B., Holman M.J., Seager S., Steffen J.H., Welsh W.F., Rowe J., Anderson H., Buchhave L., Ciardi D., Walkowicz L., Sherry W., Horch E., Isaacson H., Everett M.E., Fisher D., Torres G., Johnson J.A., Endl M., MacQueen P., Bryson S.T., Dotson J., Haas M., Kolodziejczak J., Van Cleve J., Chandrasekaran H., Twicken J.D., Quintana E.V., Clarke B.D., Allen C., Lie J., Wu H., Tenembaum P., Verner E., Bruhweiler F., Barnes J., and Prsa A. (2010) Kepler planet-detection mission: introduction and first results . Science 327 :977–980 [ PubMed ] [ Google Scholar ]
- Brack A. (2005) From the origin of life on Earth to life in the Universe . In Lectures in Astrobiology , Vol. 1 , edited by Gargaud M., Springer, Berlin, pp 3–21 [ Google Scholar ]
- Branscomb E. and Russell M.J. (2013) Turnstiles and bifurcators: the disequilibrium converting engines that put metabolism on the road . Biochim Biophys Acta 1827 :62–78 [ PubMed ] [ Google Scholar ]
- Burchell M.J., Mann J.R., and Bunch A.W. (2004) Survival of bacteria and spores under extreme pressures . Mon Not R Astron Soc 352 :1273–1278 [ Google Scholar ]
- Bürger R. and Lynch M. (1995) Evolution and extinction in a changing environment: a quantitative-genetic analysis . Evolution 49 :151–163 [ PubMed ] [ Google Scholar ]
- Burrows A.S. (2014) Highlights in the study of exoplanet atmospheres . Nature 513 :345–352 [ PubMed ] [ Google Scholar ]
- Chopra A. and Lineweaver C.H. (2016) The case for a Gaian bottleneck: the biology of habitability . Astrobiology 16 :7–22 [ PubMed ] [ Google Scholar ]
- Cleland C.E. and Copley S.D. (2005) The possibility of alternative microbial life on Earth . International Journal of Astrobiology 4 :165–173 [ Google Scholar ]
- Cocconi G. and Morrison P. (1959) Searching for interstellar communications . Nature 184 :844–846 [ Google Scholar ]
- Cockell C.S., Bush T., Bryce C., Direito S., Fox-Powell M., Harrison J.P., Lammer H., Landenmark H., Martin-Torres J., Nicholson N., Noack L., O'Malley-James J., Payler S.J., Rushby A., Samuels T., Schwendner P., Wadsworth J., and Zorzano M.P. (2016) Habitability: a review . Astrobiology 16 :89–117 [ PubMed ] [ Google Scholar ]
- Colwell R.K. (2009) Biodiversity: concepts, patterns and measurement . In The Princeton Guide to Ecology , edited by Levin S.A., Princeton University Press, Princeton, NJ, pp 257–263 [ Google Scholar ]
- Colwell R.K. and Coddington J.A. (1994) Estimating terrestrial biodiversity through extrapolation . Philos Trans R Soc Lond B Biol Sci 345 :101–118 [ PubMed ] [ Google Scholar ]
- Cowan J.J. and Sneden C. (2006) Heavy element synthesis in the oldest stars and early universe . Nature 440 :1151–1156 [ PubMed ] [ Google Scholar ]
- Crossfield I.J.M. (2015) Observations of exoplanet atmospheres . Publ Astron Soc Pac 127 :941–960 [ Google Scholar ]
- Crowe M.J. (2011) The Extraterrestrial Life Debate, 1750–1900 , Dover Publications, Mineola, NY [ Google Scholar ]
- Darling D. and Schulze-Makuch D. (2016) The Extraterrestrial Encyclopedia , First Edition Design Publishing, Sarasota, FL [ Google Scholar ]
- Davies P.C., Benner S.A., Cleland C.E., Lineweaver C.H., McKay C.P., and Wolfe-Simon F. (2009) Signatures of the shadow biosphere . Astrobiology 9 :241–291 [ PubMed ] [ Google Scholar ]
- Davila A.F. and McKay C.P. (2014) Chance and necessity in biochemistry: implications for the search of extraterrestrial biomarkers in Earth-like environments . Astrobiology 14 :534–540 [ PMC free article ] [ PubMed ] [ Google Scholar ]
- de Fontenelle B.B. (1686) Entretiens sur la Pluralité des Mondes , Nouvelle Edition augmentée (1701), Pierre Mortier, Librairie fur le Vygen-Dam, Amsterdam [ Google Scholar ]
- Deroo P., Swain M.R., and Green O. (2012) Spectroscopy of exoplanet atmospheres with the FINESSE Explorer mission . Proc SPIE 8442 , doi: 10.1117/12.925236 [ CrossRef ] [ Google Scholar ]
- Des Marais D.J. (1999) Astrobiology: exploring the origins, evolution, and distribution of life in the Universe . Annu Rev Ecol Syst 30 :397–420 [ PubMed ] [ Google Scholar ]
- Des Marais D.J., Nuth J.A., III, Allamandola L.J., Boss A.P., Farmer J.D., Hoehler T.M., Jakosky B.M., Meadows V.S., Pohorille A., Runnegar B., and Spormann A.M. (2008) The NASA Astrobiology Roadmap . Astrobiology 8 :715–730 [ PubMed ] [ Google Scholar ]
- Descartes R. (1644) Principia Philosophiae , apud Ludovicum Elzevirum, Ghent University, Belgium [ Google Scholar ]
- Dick S.J. (1984) Plurality of Worlds: The Origins of the Extraterrestrial Life Debate from Democritus to Kant , Cambridge University Press, Cambridge, UK [ Google Scholar ]
- Dietrich L.E.P, Michael M., and Newman D.K. (2006) The co-evolution of life and Earth . Curr Biol 16 :pR395–pR400 [ PubMed ] [ Google Scholar ]
- Domagal-Goldman S.D., Meadows V.S., Claire M.W., and Kasting J.F. (2011) Using biogenic sulfur gases as remotely detectable biosignatures on anoxic planets . Astrobiology 11 :419–441 [ PMC free article ] [ PubMed ] [ Google Scholar ]
- Doyle L.R., McCowan B., Johnston S., and Hanser S.F. (2011) Information theory, animal communication, and the search for extraterrestrial intelligence . Acta Astronaut , SETI Special Edition, 68 :406–417 [ Google Scholar ]
- Drake F. (2011) The search for extra-terrestrial intelligence . Philos Trans A Math Phys Eng Sci 369 :633–643 [ PubMed ] [ Google Scholar ]
- Dyson F.J. (1999) Origins of Life , 2 nd ed., Cambridge University Press, Cambridge, UK [ Google Scholar ]
- Eliott J. (2011a) A human language corpus for interstellar message construction . Acta Astronaut , SETI Special Edition, 68 :418–424 [ Google Scholar ]
- Eliott J. (2011b) A semantic “engine” for universal translation . Acta Astronaut , SETI Special Edition, 68 :435–440 [ Google Scholar ]
- Eliott J. (2011c) A post-detection decipherment strategy . Acta Astronaut , SETI Special Edition, 68 :441–444 [ Google Scholar ]
- Fabbro F., Aglioti S.M., Bergamasco M., Clarici A., and Panksepp J. (2015) Evolutionary aspects of self- and world consciousness in vertebrates . Front Hum Neurosci 9 , doi: 10.3389/fnhum.2015.00157 [ PMC free article ] [ PubMed ] [ CrossRef ] [ Google Scholar ]
- Fitz D., Reiner H., and Rode B.M. (2007) Chemical evolution toward the origin of life . Pure Appl Chem 79 :2101–2117 [ Google Scholar ]
- Flammarion C. (1872) La Pluralité des Mondes Habités , Didier, Paris [ Google Scholar ]
- Forgan D.H. (2014) Can collimated extraterrestrial signals be intercepted? J Br Interplanet Soc 67 :232–236 [ Google Scholar ]
- Gaidos E., Anderson D.R., Lepine S., Colon K.D., Maravelias G., Narita N., Chang E., Beyer J., Fukui A., Armstrong J.D., Zezas A., Fulton B.J., Mann A.W., West R.G., and Faedi F. (2014) Trawling for transits in a sea of noise: a search for exoplanets by analysis of WASP optical light curves and follow-up (SEAWOLF) . Mon Not R Astron Soc 437 :3133–3143 [ Google Scholar ]
- Garber S.J. (1999) Searching for good science: the cancellation of NASA's SETI Program . J Br Interplanet Soc 52 :3–12 [ Google Scholar ]
- Gaston K.J. (2000) Global patterns in biodiversity . Nature 405 :220–227 [ PubMed ] [ Google Scholar ]
- Geary D.C. (2005) The Origin of the Mind: Evolution of Brain, Cognition, and General Intelligence , 1 st ed., American Psychological Association, Washington, DC [ Google Scholar ]
- Gibb B.C. (2013) Life, the Universe and nomenclature . Nat Chem 5 , doi: 10.1038/nchem.1530 [ PubMed ] [ CrossRef ] [ Google Scholar ]
- Gladman B., Dones L., Levison H.F., and Burns J.A. (2005) Impact seeding and reseeding in the inner Solar System . Astrobiology 5 :483–496 [ PubMed ] [ Google Scholar ]
- Golding S.D. and Glikson M. (2011) Earliest Life on Earth: Habitats, Environments and Methods of Detection , Springer, Dordrecht, the Netherlands [ Google Scholar ]
- Gould S.J. (1998) Evolution and Extinction , Audio Scholar, Mendocino, CA [ Google Scholar ]
- Graham S.L., Snir M., and Patterson C.A., editors. (2005) The Future of Supercomputing , The National Academies Press, Washington, DC [ Google Scholar ]
- Grazier K.R. (2016) Jupiter: cosmic Jekyll and Hyde . Astrobiology 16 :23–38 [ PubMed ] [ Google Scholar ]
- Grenfell J.L., Rauer H., Selsis F., Kaltenegger L., Beichman C., Danchi W., Eiroa C., Fridlund M., Henning T., Herbst T., Lammer H., Léger A., Lisaeu R., Lunine J., Paresce F., Penny A., Quirrenbach A., Röttgering H., Schneider J., Stam D., Tinetti G., and White G.J. (2010) Co-evolution of atmospheres, life, and climate . Astrobiology 10 :77–88 [ PubMed ] [ Google Scholar ]
- Grinspoon D.H. (2012) Assessing the Anthropocene in the context of comparative planetology [abstract #P14A-02] . In American Geophysical Union, Fall Meeting 2012 , American Geophysical Union, Washington, DC [ Google Scholar ]
- Gulkis S., Olsen E.T., and Tarter J.C. (1981) A bimodal search strategy for SETI . In Strategies for the Search for Life in the Universe , edited by Papagiannis M.D., D. Reidel Publishing Co., Dordrecht, the Netherlands, pp 93–105 [ Google Scholar ]
- Harp G.R., Richards J., Shostak S., Tarter J.C., Vakoch D.A., and Munson C. (2015) Radio SETI observations of the anomalous star KIC 8462852 . arXiv:1511.01606
- Hatzes A.P. (2014) The detection of Earth-mass planets around active stars: the mass of Kepler-78B . Astron Astrophys 568 , doi: 10.1051/0004-6361/201424025 [ CrossRef ] [ Google Scholar ]
- Heath M.J., Doyle L.R., Joshi M.J., and Haberle R. (1998) Habitability of planets around red dwarf stars . Orig Life Evol Biosph 29 :405–424 [ PubMed ] [ Google Scholar ]
- Heinecke A., Eckhardt W., Horsch M., and Bungartz H.-J. (2015) Supercomputing for Molecular Dynamics Simulations: Handling Multi-Trillion Particles in Nanofluidics , Springer Briefs in Computer Science, Springer International Publishing, Cham, Switzerland [ Google Scholar ]
- Heller R. and Pudritz R.E. (2016) The search for extraterrestrial intelligence in Earth's solar transit zone . Astrobiology 16 :259–270 [ PubMed ] [ Google Scholar ]
- Heller R., Leconte J., and Barnes R. (2011) Tidal obliquity evolution of potentially habitable planets . Astron Astrophys 528 , doi: 10.1051/0004-6361/201015809 [ CrossRef ] [ Google Scholar ]
- Horner J., Waltham D., and Koch F.E. (2013) The role of Jupiter in driving Earth's orbital evolution . In Proceedings of the 13 th Australian Space Science Conference, Sept. 30–Oct. 2, 2013 [ Google Scholar ]
- Houri-Ze'evi L., Korem Y., Sheftel H., Faigenbloom L., Toker I.A., Dagan Y., Awad L., Degani L., Alon U., and Rechavi O. (2016) A tunable mechanism determines the duration of the transgenerational small RNA inheritance in C. elegans . Cell 165 :88–99 [ PubMed ] [ Google Scholar ]
- Ilhan H. and Linscott I. (2011) Using fractal derivatives as “degree of symmetry” to characterize natural shapes . Acta Astronaut , SETI Special Edition, 68 :425–434 [ Google Scholar ]
- Imari Walker S. (2014) Transition from abiotic to biotic: is there an algorithm for it? In An Evolutionary Approach , edited by Kolb V., CRC Press, Boca Raton, FL, pp 371–398 [ Google Scholar ]
- Irwin N.I. and Schulze-Makuch D. (2001) Assessing the plausibility of life on other worlds . Astrobiology 1 :143–160 [ PubMed ] [ Google Scholar ]
- Irwin N.I., Méndez A., Fairén A.G., and Schulze-Makuch D. (2014) Assessing the possibility of biological complexity on other worlds, with an estimate of the occurrence of complex life in the Milky Way Galaxy . Challenges 5 :159–174 [ Google Scholar ]
- Jerison H.J. and Jerison I. (2013) Intelligence and Evolutionary Biology , NATO ASI Series G, Ecological Sciences, Vol. 17 Springler-Verlag, Berlin [ Google Scholar ]
- Kant I. (2009) Universal Natural History and Theory of the Heavens , Translated by Johnston I.C., Richer Resources Publications, Arlington, VA: (Original work published in 1755) [ Google Scholar ]
- Kasting J.F. (1993) Earth's earliest atmosphere . Science 259 :920–926 [ PubMed ] [ Google Scholar ]
- Keller G. and Kerr A. (2014) Volcanism, Impacts, and Mass Extinctions: Causes and Effects , GSA Special Paper 505, Geological Society of America, Boulder, CO [ Google Scholar ]
- Kipping D.M. and Teachey A. (2016) A cloaking device for transiting planets . Mon Not R Astron Soc 458 , doi: 10.1093/mnras/stw672 [ CrossRef ] [ Google Scholar ]
- Knoll A.H. (2009) The co-evolution of life and environments . Rendiconti Lincei Scienze Fisiche e Naturali 20 :301–306 [ Google Scholar ]
- Kolb V. (2014) Astrobiology: An Evolutionary Approach , CRC Press, Boca Raton, FL [ Google Scholar ]
- Kooijman S.A.L.M. (2004) On the co-evolution of life and its environment . In Scientists Debate Gaia: The Next Century , edited by Schneider S.H., Miller J.R., Crist E., and Boston P.J., MIT Press Scholarship Online, Cambridge, MA, doi: 10.7551/mitpress/9780262194983.003.0032 [ CrossRef ] [ Google Scholar ]
- Kopparapu R., Ravi K., Ramirez R., Kasting J.F., Eymet V., Robinson T.D., Mahadevan S., Terrien R., Ryan C., Domagal-Goldman S., Meadows V., and Deshpande R. (2013) Habitable zones around main sequence stars: new estimates . Astrophys J 765 , doi: 10.1088/0004-637X/765/2/131 [ CrossRef ] [ Google Scholar ]
- Krissansen-Totton J., Bergsman D.S., and Catling D.C. (2016) On detecting biospheres from chemical thermodynamic disequilibrium in planetary atmospheres . Astrobiology 16 :39–67 [ PubMed ] [ Google Scholar ]
- Krubitzer L. (2014) Lessons from evolution . In The Future of the Brain: Essays by the World's Leading Neuroscientists , edited by Marcus G. and Freeman J., Princeton University Press, Princeton, NJ, pp 186–193 [ Google Scholar ]
- Lai S., Safaei J., and Pelech S. (2016) Evolutionary ancestry of eukaryotic protein kinases and choline kinases . J Biol Chem 291 :5199–5205 [ PMC free article ] [ PubMed ] [ Google Scholar ]
- Laskar J., Joutel F., and Robutel P. (1993) Stabilization of the Earth's obliquity by the Moon . Nature 361 :615–617 [ Google Scholar ]
- Lazcano A. and Miller S.L. (1996) The origin and early evolution of life: prebiotic chemistry, the pre-RNA world, and time . Cell 85 :793–798 [ PubMed ] [ Google Scholar ]
- Lehman J. and Miikkulainen R. (2015) Extinction events can accelerate evolution . PLoS One 10 , doi: 10.1371/journal.pone.0132886 [ PMC free article ] [ PubMed ] [ CrossRef ] [ Google Scholar ]
- Lissauer J.J., Barnes J.W., and Chambers J.E. (2011) Obliquity variations of a moonless Earth . Icarus 217 :77–87 [ Google Scholar ]
- Locey K.J. and Lennon J.T. (2016) Scaling laws predict global microbial diversity . Proc Natl Acad Sci USA 113 :5970–5975 [ PMC free article ] [ PubMed ] [ Google Scholar ]
- Lowell P. (1906) Mars and Its Canals , Macmillan Company, New York [ Google Scholar ]
- Lynch M. and Lande R. (1993) Evolution and extinction in response to environmental change . In Biotic Interactions and Global Change , edited by Kingsolver J.G. and Huey R.B., Sinauer, Sunderland, MA [ Google Scholar ]
- Lyons T.W., Reinhard C.T., and Planavsky N.J. (2014) The rise of oxygen in Earth's early ocean and atmosphere . Nature 506 :307–315 [ PubMed ] [ Google Scholar ]
- Maccone C. (2012) Mathematical SETI: Statistics, Signal Processing, Space Missions , Springer-Verlag, Berlin [ Google Scholar ]
- Macintosh B., Graham J.R., Barman T., De Rosa R.J., Konopacky Q., Marley M.S., Marois C., Nielsen E.L, Pueyo L., Rajan A., Rameau J., Saumon D., Wang J.J., Ammons M., Arriaga P., Artigau E., Beckwith S., Brewster J., Bruzzone S., Bulger J., Burningham B., Burrow A.S., Chen C., Chiang E., Chilcote J.K., Dawson R.I., Dong R., Doyon R., Draper Z.H., Duchêne G., Esposito T.M., Fabrycky D., Fitzgerald M.P., Follette K.B., Fortney J.J., Gerard B., Goodsell S., Greenbaum A.Z., Hibon P., Hinkley S., Cotton T.H., Hung L.-W., Ingraham P., Johnson-Groh M., Kalas P., Lafreniere D., Larkin J.E., Lee J., Line M., Long D., Maire J., Marchis F., Matthews B.C., Max C.E., Metchev S., Millar-Blanchaer M.A., Mittal T., Morley C.V., Morzinskim K.M., Murray-Clay R., Oppenheimer R., Palmer D.W., Patel R., Patience J., Perrin M.D., Poyneer L.A., Rafikov R.R., Rantakyrö F.T., Rice E., Rojo P., Rudy A.R., Ruffio J.-B., Ruiz M.T., Sadakuni N., Saddlemyer L., Salama M., Savransky D., Schneider A.C., Sivaramakrishnan A., Song I., Soummer R., Thomas S., Vasisht G., Wallace J.K., Ward-Duong K., Wiktorowicz S.J., Wollff S.G., and Zuckerman B. (2015) Discovery and spectroscopy of the young jovian planet 51 Eri b with the Gemini Planet Imager . Science 350 :64–67 [ PubMed ] [ Google Scholar ]
- Mandelbrot B.B. (1982) The Fractal Geometry of Nature , W.H. Freeman and Company, San Francisco [ Google Scholar ]
- Mandell A.V.(2008) Expanding and improving the search for habitable worlds . In New Horizons in Astronomy: Frank N. Bash Symposium 2007, ASP Conference Series Vol. 393 , Astronomical Society of the Pacific, San Francisco, pp 19–34 [ Google Scholar ]
- Margulis L. (1996) Archaeal-eubacterial mergers in the origin of Eukarya: phylogenetic classification of life . Proc Natl Acad Sci USA 93 :1071–1076 [ PMC free article ] [ PubMed ] [ Google Scholar ]
- Martin W., Baross J., Kelley D., and Russel M. (2008) Hydrothermal vents and the origin of life . Nat Rev 6 :805–814 [ PubMed ] [ Google Scholar ]
- McCollom T.M. (2013) Miller-Urey and beyond: what have we learned about prebiotic organic synthesis reactions in the past 60 years? Annu Rev Earth Planet Sci 41 :207–229 [ Google Scholar ]
- McGinley M. (2014) Species richness . In The Encyclopedia of Earth (EoE) . Retrieved from http://www.eoearth.org/view/article/156216
- McKay C.P. (2004) What is life—and how do we search for it in other worlds? PLoS Biol 2 , doi: 10.1371/journal.pbio.0020302 [ PMC free article ] [ PubMed ] [ CrossRef ] [ Google Scholar ]
- Melosh J. (1996) Impact Cratering: A Geologic Process , Oxford Monographs on Geology and Geophysics no. 11, Oxford University Press, New York [ Google Scholar ]
- Monod J. (1972) Chance and Necessity: An Essay on the Natural Philosophy of Modern Biology , Vintage Books, New York [ Google Scholar ]
- Mora C., Tittensor D.P., Adl S., Simpson A.G.B., and Worm B. (2011) How many species are there on Earth and in the ocean? PLoS Biol 9 , doi: 10.1371/journal.pbio.1001127 [ PMC free article ] [ PubMed ] [ CrossRef ] [ Google Scholar ]
- Musso P. (2012) The problem of active SETI: an overview . Acta Astronaut 78 :43–54 [ Google Scholar ]
- Napier W.M. (2004) A mechanism for interstellar panspermia . Mon Not R Astron Soc 348 :46–51 [ Google Scholar ]
- Neal M. (2014) Preparing for extraterrestrial contact . Risk Management 16 :63–87 [ Google Scholar ]
- Nealson K.H. and Conrad P.G. (1999) Life: past, present and future . Philos Trans R Soc Lond B Biol Sci 354 :1923–1939 [ PMC free article ] [ PubMed ] [ Google Scholar ]
- Nolfi S. and Parisi D. (1996) Learning to adapt to changing environments in evolving neural networks . Adapt Behav 5 :75–98 [ Google Scholar ]
- Ollongren A. and Vakoch D.A. (2011) Large-size message construction for ETI: logical existence expressed in Lingua Cosmica . In SETI Science and Technology Review Meeting, 62 nd International Astronautical Congress, Cape Town, South Africa [ Google Scholar ]
- O'Malley-James J.T., Cockell C.S., Greaves J.S., and Raven J.A. (2014) Swansong biospheres II: The final signs of life on terrestrial planets near the end of their habitable lifetimes . International Journal of Astrobiology 13 :229–243 [ Google Scholar ]
- Owen T. (2008) The contributions of comets to planets, atmospheres, and life: insights from Cassini-Huygens, Galileo, Giotto, and inner planet missions . Origin and Early Evolution of Comet Nuclei: Workshop Honouring Johannes Geiss on the Occasion of His 80 th Birthday, Space sciences series of ISSI, Vol. 28 , Springer, New York, pp 301–316 [ Google Scholar ]
- Pascal R., Boiteau L., Forterre P., Gargaud M., Lazcano A., López-García P., Moreira D., Maurel M.-C., Peretó J., Prieur D., and Reisse J. (2006) Prebiotic chemistry—biochemistry—emergence of life (4.4–2 Ga) . Earth Moon Planets 98 :153–203 [ Google Scholar ]
- Perlovsky L.I. (2006) Toward physics of the mind: concepts, emotions, consciousness, and symbols . Phys Life Rev 3 :23–55 [ Google Scholar ]
- Petigura E.A., Howard A.W., and Marcy G.W. (2013) Prevalence of Earth-size planets orbiting Sun-like stars . Proc Natl Acad Sci USA 110 :19273–19278 [ PMC free article ] [ PubMed ] [ Google Scholar ]
- Pfeifer R. and Gómez G. (2006) Morphological computation—connecting brain, body, and environment . In Creating Brain-Like Intelligence , Lecture Notes in Computer Science 5436, edited by Sendhoff B., Springer, Berlin, pp 66–83 [ Google Scholar ]
- Pigliucci M. and Müller G.B., editors. (2010) Evolution, the Extended Synthesis , MIT Press, Cambridge, MA [ Google Scholar ]
- Pilcher C.B. (2004) Biosignatures of early Earths . Astrobiology 3 :471–486 [ PubMed ] [ Google Scholar ]
- Popa R. (2004) Between Necessity and Probability: Searching for the Definition and Origin of Life , Springer, Berlin [ Google Scholar ]
- Pringle J.W.S. (1951) On the parallel between learning and evolution . Behaviour 3 :174–214 [ Google Scholar ]
- Pross A. (2012) What Is Life? How Chemistry Becomes Biology , Oxford University Press, Oxford, UK [ Google Scholar ]
- Pross A. and Pascal R. (2013) The origin of life: what we know, what we can know, and what we will never know . Open Biology 3 , doi: 10.1098/rsob.120190 [ PMC free article ] [ PubMed ] [ CrossRef ] [ Google Scholar ]
- Quintana E.V., Barclay T., Raumond S.N., Rowe J.F., Bolmont E., Caldwell D.A., Howell S.B., Kane S.R., Huber D., Crepp J.R., Lissauer J.J., Ciardi D.R., Coughlin J.L., Everett M.E., Henze C.E., Horch E., Isaacson H., Ford E.B., Adams F.C., Still M., Hunter R.C., Quarles B., and Selsis F. (2014) An Earth-sized planet in the habitable zone of a cool star . Science 344 :277–280 [ PubMed ] [ Google Scholar ]
- Rao R.P.N., Stocco A., Bryan M., Sarma D., and Youngquist T.M. (2014) A direct brain-to-brain interface in humans . PLoS One 9 , doi: 10.1371/journal.pone.0111332 [ PMC free article ] [ PubMed ] [ CrossRef ] [ Google Scholar ]
- Raup D.M. (1999) The role of extinction in evolution . Proc Natl Acad Sci USA 91 :6758–6763 [ PMC free article ] [ PubMed ] [ Google Scholar ]
- Rose C. and Wright G. (2004) Inscribed matter as an energy-efficient means of communication with an extraterrestrial civilization . Nature 431 :47–49 [ PubMed ] [ Google Scholar ]
- Rosenfeld S. (2011) Mathematical descriptions of biochemical networks: stability, stochasticity, evolution . Prog Biophys Mol Biol 106 :400–409 [ PMC free article ] [ PubMed ] [ Google Scholar ]
- Roth G. (2013) The Long Evolution of Brains and Minds , Springer, Dordrecht, the Netherlands [ Google Scholar ]
- Ruiz-Mirazo K., Brionest C., and de la Escosura A. (2013) Prebiotic systems chemistry: new perspectives on the origins of life . Chem Rev 114 :285–366 [ PubMed ] [ Google Scholar ]
- Russell M.J., Nitschke W., and Branscomb E. (2013) The inevitable journey to being . Philos Trans R Soc Lond B Biol Sci 368 , doi: 10.1098/rstb.2012.0254 [ PMC free article ] [ PubMed ] [ CrossRef ] [ Google Scholar ]
- Sagan C. (1975) Communication with Extraterrestrial Intelligence , MIT Press, Cambridge, MA [ Google Scholar ]
- Sasselov D. (2012) The Life of Super-Earths: How the Hunt for Alien Worlds and Artificial Cells Will Revolutionize Life on our Planet , Basic Books, New York [ Google Scholar ]
- Schrey A.W., Richards C.L., Meller V., Sollars V., and Ruden D.M. (2012) The role of epigenetics in evolution: the extended synthesis . Genet Res Int 2012 , doi: 10.1155/2012/286164 [ PMC free article ] [ PubMed ] [ CrossRef ] [ Google Scholar ]
- Schuch H.P. (2011) Project Ozma: the birth of observational SETI . In Searching for Extraterrestrial Intelligence , The Frontiers Collection, Springer, Berlin, pp 13–19 [ Google Scholar ]
- Schuetz M., Vakoch D.A., Shostak S., and Richards J. (2015) Optical SETI observations of the anomalous star KIC 8462852 . arXiv:1512.02388
- Schulkin J. (2014) Cognitive Adaptation: A Pragmatist Perspective , Cambridge University Press, Cambridge, UK [ Google Scholar ]
- Schulze-Makuch D. and Irwin L.N. (2001) Lessons from the history of life on Earth . In Life in the Universe , edited by Schulze-Makuch D. and Irwin L.N., Advances in Astrobiology and Biogeophysics, Springer, Berlin, pp 25–41 [ Google Scholar ]
- Schulze-Makuch D., Irwin L.N., and Fairén A.G. (2013) Drastic environmental change and its effects on a planetary biosphere . Icarus 225 :775–780 [ Google Scholar ]
- Schwartz R.N. and Townes C.H. (1961) Interstellar and interplanetary communication by optical masers . Nature 190 :205–208 [ Google Scholar ]
- Schwartzman D.W. (2004) Does life drive disequilibrium in the biosphere? In Scientists Debate Gaia: The Next Century , edited by Schneider S.H., Miller J.R., Crist E., and Boston P.J., MIT Press, Cambridge, MA, doi: 10.7551/mitpress/9780262194983.003.0013 [ CrossRef ] [ Google Scholar ]
- Schwieterman E.W., Meadows V.S., Domagal-Goldman S.D., Deming D., Arney G.N., Luger R., Harman C.E., Misra A., and Barnes R. (2016) Identifying planetary biosignature impostors: spectral features of CO and O 4 resulting from abiotic O 2 /O 3 production . Astrophys J 819 , doi: 10.3847/2041-8205/819/1/L13 [ PMC free article ] [ PubMed ] [ CrossRef ] [ Google Scholar ]
- Seager S. (2013) Exoplanet habitability . Science 340 :577–581 [ PubMed ] [ Google Scholar ]
- Seager S. (2014) The future of spectroscopic life detection on exoplanets . Proc Natl Acad Sci USA 11 :12634–12640 [ PMC free article ] [ PubMed ] [ Google Scholar ]
- Seager S. and Deming D. (2010) Exoplanet atmospheres . Annu Rev Astron Astrophys 48 :631–672 [ Google Scholar ]
- Seckbach J., editor. (2006) Life as We Know It , Cellular Origin and Life in Extreme Habitats and Astrobiology, Vol. 10 , Springer, Dordrecht, the Netherlands [ Google Scholar ]
- Seckbach J., Chela-Flores J., Owen T., andRaulin F., editors. (2004) Life in the Universe: From the Miller Experiment to the Search for Life on Other Worlds , Cellular Origin and Life in Extreme Habitats and Astrobiology, Vol. 7 , Springer, Dordrecht, the Netherlands [ Google Scholar ]
- Segura A., Kasting J.F., Meadows V., Cohen M., Scalo J., Crisp D., Butler R.A.H., and Tinetti G. (2005) Biosignatures from Earth-like planets around M dwarfs . Astrobiology 5 :706–725 [ PubMed ] [ Google Scholar ]
- Seifer M.J. (1996) Martian fever (1895–1896) . In Wizard: The Life and Times of Nikola Tesla: Biography of a Genius , Carol Publishing Group, Secaucus, NJ [ Google Scholar ]
- Shapiro R. (2006) Small molecule interactions were central to the origin of life . Q Rev Biol 81 :105–125 [ PubMed ] [ Google Scholar ]
- Shostak S. (1998) Sharing the Universe: Perspectives on Extraterrestrial Life , Berkeley Hills Book, Berkeley, CA [ Google Scholar ]
- Shostak S. (2011a) Short-pulse SETI . Acta Astronaut , SETI Special Edition, 68 :362–365 [ Google Scholar ]
- Shostak S. (2011b) Limits on interstellar messages . Acta Astronaut , SETI Special Edition, 68 :366–371 [ Google Scholar ]
- Smith R.E. and Cribbs H.B., III, editors. (1994) Evolutionary Computation , MIT Press, Cambridge, MA [ Google Scholar ]
- Spohn T. (1991) Mantle differentiation and thermal evolution of Mars, Mercury, and Venus . Icarus 90 :222–236 [ Google Scholar ]
- Stern R.J. (2015) Is plate tectonics needed to evolve technological species on exoplanets? Geoscience Frontiers 7 :573–580 [ Google Scholar ]
- Strausfeld N.J. and Hirth F. (2015) Introduction to “Homology and convergence in nervous system evolution.” Philos Trans R Soc Lond B Biol Sci 317, doi: 10.1098/rstb.2015.0034 [ PMC free article ] [ PubMed ] [ CrossRef ] [ Google Scholar ]
- Summons R.E., Amend J.P., Bish D., Buik R., Cody G.D., Des Marais D.J., Dromart G., Eigenbrode J.L., Knoll A.H., and Sumner D.Y. (2011) Preservation of martian organic and environmental records: final report of the Mars Biosignature Working Group . Astrobiology 11 :157–181 [ PubMed ] [ Google Scholar ]
- Swendenborg E. (1758) Life on Other Planets , 1 st ed., Swedenborg Foundation, West Chester, PA [ Google Scholar ]
- Tarter J.C. (1979) The Cosmic Haystack , Harlan Smith Working Group on SETI of the National Academy of Sciences Astronomy and Astrophysics for the 1980s Survey Committee [ Google Scholar ]
- Tarter J.C. (1983) SETI Program . Letter to Science 22 :359 [ PubMed ] [ Google Scholar ]
- Tarter J.C. (2001a) The search for extraterrestrial intelligence (SETI) . Annu Rev Astron Astrophys 39 :511–548 [ Google Scholar ]
- Tarter J.C. (2001b) What is SETI? Ann NY Acad Sci 950 :269–275 [ PubMed ] [ Google Scholar ]
- Tarter J.C. (2004) Astrobiology and SETI . New Astronomy Reviews 48 :1543–1549 [ Google Scholar ]
- Tarter J.C. (2007) The evolution of life in the Universe: are we alone? Proceedings of the International Astronomical Union 2 :14–29 [ Google Scholar ]
- Tarter J.C. and Chyba C.F. (1999) Is there life elsewhere in the Universe? Sci Am 281 :80–85 [ Google Scholar ]
- Tarter J.C., Backus P., Mancinelli R., Aurnou J., Backman D., Basri G., Boss A., Clarke A., Deming D., Doyle L., Feigelson E., Freund F., Grinspoon D., Haberle R., Hauck S., Heath M., Henry T., Hollingsworth J., Joshi M., Jura M., Kilston S., Laughlin G., Liu E., Meikle M., Reid I., Rothschild L., Scalo J., Segura A., Tang C., Tiedje J., Turnbull M., Walkowicz L., Weber A., and Young R. (2007) A reappraisal of the habitability of planets around M dwarf stars . Astrobiology 7 :30–65 [ PubMed ] [ Google Scholar ]
- Turnbull M.C. and Tarter J.C. (2003) Target selection for SETI. I. A catalog of nearby habitable stellar systems . Astrophys J Suppl Ser 145 :181–198 [ Google Scholar ]
- Vakoch D.A., editor. (2011a) Communication with Extraterrestrial Intelligence , State University of New York Press, Albany, NY [ Google Scholar ]
- Vakoch D.A. (2011b) A taxonomic approach to communicating maxims in interstellar messages . Acta Astronaut , SETI Special Edition, 68 :500–511 [ Google Scholar ]
- Vakoch D.A. (2011c) A narratological approach to interpreting and designing interstellar messages . Acta Astronaut , SETI Special Edition, 68 :520–534 [ Google Scholar ]
- Vakoch D.A., editor. (2014) Between Worlds: The Art and Science of Interstellar Message Composition , MIT Press, Cambridge, MA [ Google Scholar ]
- Vakoch D.A. and Dowd M.F. (2015) The Drake Equation: Estimating the Prevalence of Extraterrestrial Life through the Ages , Cambridge Astrobiology Series Book 8, Cambridge University Press, Cambridge, UK [ Google Scholar ]
- Vakoch D.A. and Harrison A.H., editors. (2011) Civilization beyond Earth: Extraterrestrial Life and Society , Berghahn Books, New York [ Google Scholar ]
- Vakoch D.A. and Matessa M. (2011) An algorithmic approach to communicating reciprocal altruism in interstellar messages: drawing analogies between social and astrophysical phenomena . Acta Astronaut , SETI Special Edition, 68 :459–475 [ Google Scholar ]
- Valiant L. (2013) Probably Approximately Correct: Nature's Algorithms for Learning and Prospering in a Complex World , Basic Books, New York [ Google Scholar ]
- Voltaire F.-M.A. (2002) Micromégas and Other Short Fictions , translated by Cuffe T. and Mason H.T., Penguin Classics, New York. (Original work published in 1752) [ Google Scholar ]
- Wagner A. (2007) Robustness and Evolvability in Living Systems , Princeton University Press, Princeton, NJ [ Google Scholar ]
- Wagner G.P. and Draghi J. (2010) Evolution of evolvability . In Evolution: The Extended Synthesis , edited by Pigliucci M. and Müller G.B., MIT Press, Cambridge, MA, pp 218–228 [ Google Scholar ]
- Wagner G.P., Pavlicev M., and Cheverud J.M. (2007) The road to modularity . Nat Rev Genet 8 :921–931 [ PubMed ] [ Google Scholar ]
- Walker S.I. (2014) Top-down causation and the rise of information in the emergence of life . Information 5 :424–439 [ Google Scholar ]
- Ward P.D. and Benner S.A. (2007) Alien biochemistries . In Planets and Life: The Emerging Science of Astrobiology , edited by Sullivan W.T., III and Baross J.A., Cambridge University Press, Cambridge, UK, pp 537–544 [ Google Scholar ]
- Ward P.D. and Brownlee D. (2003) Rare Earth: Why Complex Life is Uncommon in the Universe , Copernicus Books, New York [ Google Scholar ]
- Waters C.N., Zalasiewicz J., Summerhayes C., Barnosky A.D., Poirier C., Galuszka A., Cearreta A., Edgeworth M., Ellis E.C., Ellis M., Jeandel C., Leindfelder R., McNeill J.R., deB. Richter D., Steffen W., Syvitski J., Vids D., Wagreich M., Williams M., Grinevald J., Odada E., Oreskes N., and Wolfe A.P. (2016) The Anthropocene is functionally and stratigraphically distinct from the Holocene . Science 351 , doi: 10.1126/science.aad2622 [ PubMed ] [ CrossRef ] [ Google Scholar ]
- Watson A.J. (1999) Coevolution of the Earth's environment and life: Goldilocks, Gaia and the anthropic principle . Geol Soc Spec Publ 150 :75–88 [ Google Scholar ]
- Watson R.A. and Szathmáry E. (2015) How can evolution learn? Trends Ecol Evol 31 :147–157 [ PubMed ] [ Google Scholar ]
- Wheeler E. (2014) The “Wow” signal, Drake equation, and exoplanet considerations . J Br Interplanet Soc 67 :412–417 [ Google Scholar ]
- Witzany G. (2015) Life is physics and chemistry and communication . Ann NY Acad Sci 1341 :1–9 [ PubMed ] [ Google Scholar ]
- Wolf Y.I., Rogozin I.B., Grishin N.V., and Koonin E.V. (2002) Genome trees and the tree of life . Trends Genet 18 :472–479 [ PubMed ] [ Google Scholar ]
- Wolpaw J.R. and Winter Wolpaw E., editors. (2012) Brain-Computer Interfaces: Principles and Practice , Oxford University Press, Oxford, UK [ Google Scholar ]
- Worth R.J., Sigurdsson S., and House C.H. (2013) Seeding life on the moons of the outer planets via lithopanspermia . Astrobiology 13 :1155–1165 [ PMC free article ] [ PubMed ] [ Google Scholar ]
- Wright S.A., Werthimer D., Treffers R.R., Maire J., Marcy G.W., Stone R.P.S., Drake F., Meyer E., Dorval P., and Siemon A. (2014) A near-infrared SETI experiment: instrument overview . Proc SPIE 9147 , doi: 10.1117/12.2055616 [ CrossRef ] [ Google Scholar ]
- Yeomans D.K. (2013) Near-Earth Objects: Finding Them Before They Find Us , Princeton University Press, Princeton, NJ [ Google Scholar ]
- Zackrisson E., Calissendorff P., González J., Benson A., Johansen A., and Janson M. (2016) Terrestrial planets across space and time . arXiv:1602.00690 [ Google Scholar ]
- Ziman J., editor. (2000) Technological Innovation as an Evolutionary Process , Cambridge University Press, Cambridge, UK [ Google Scholar ]
- Zuckerman B. and Tarter J.C. (1979) Is there anyone out there? Nature 281 :528–529 [ Google Scholar ]
- Skip to main content
- Keyboard shortcuts for audio player
NASA is looking for a framework to better help you understand its deep space finds
Joe Hernandez

The moon shines over radio antennas at the operations support facility of one of the worlds largest astronomy projects, the Atacama Large Millimeter/submillimeter Array (ALMA), in the Atacama desert in northern Chile in this 2012 photo. Jorge Saenz/AP hide caption
The moon shines over radio antennas at the operations support facility of one of the worlds largest astronomy projects, the Atacama Large Millimeter/submillimeter Array (ALMA), in the Atacama desert in northern Chile in this 2012 photo.
Is there life on other planets? It's a simple question without a simple answer.
"The expectation, in particular with the public, is a yes-or-no answer. Did you find it or didn't you find it?" Jim Green, NASA's chief scientist, told NPR.
But Green says the reality is much more complex. One scientific discovery about extraterrestrial life may give us a small new insight into the universe, but it may be exaggerated by researchers or misunderstood by the public.
The discovery of the meteorite ALH 84001 — which ignited excitement about the possibility of life on Mars — is one example from history.

Strange News
Ufo report: no sign of aliens, but 143 mystery objects defy explanation.
Green walks us through another example. Say a scientist is looking at a distant exoplanet roughly the size of Earth, roughly the same distance from a star, with oxygen and clouds in its atmosphere. That means vegetation on the surface of the planet may be generating the oxygen in the atmosphere, and it's possible we've just found life outside our solar system!
"Well, I got news for you, we know that planet, and that planet is Venus," Green said. "We see oxygen in the atmosphere of Venus, and I can guarantee there is no vegetation on the surface of Venus. So that one observation, although it sounded great, doesn't give us the confidence to lean forward and say we found life or could have found life."
Just because a planet could support life doesn't mean it ever has or will.
"That extrapolation may have absolutely no basis in the science that they've done," he said, referring to researchers, "and potentially could mislead the community into saying we have found life or we're closer to finding life without a really good scale as to how far away that really is."
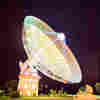
Scientists tracked a mysterious signal in space. Its source was closer to Australia
That's why Green and NASA are proposing a scale to better contextualize new findings in research about extraterrestrial life and help explain those discoveries to the public.
In an article in Nature , Green and other NASA scientists propose a seven-step rubric for understanding new discoveries. It starts with the remote detection of something that could hint at life and progresses to ruling out non-biological factors, making actual observations and finally conducting follow-up observations to be sure that life exists.

If NASA greenlights this interstellar mission, it could last 100 years
The scientists say their scale is just a proposal, and they're looking for the scientific community to weigh in and improve it.
According to the article, an objective standard is needed now because the current generation of scientists may be the one to discover life beyond Earth.
"Without the scale, it's hard to tell we're making progress, we're going in the right direction," Green said.
Aliens may be hitching rides on meteors to colonize the cosmos, study suggests. Here's how we could spot them.
A fringe theory called "panspermia" suggests that lifeforms can spread to new planets by hitching rides on meteors. New research lays out a roadmap for finding where these hypothetical, planet-hopping aliens may reside.
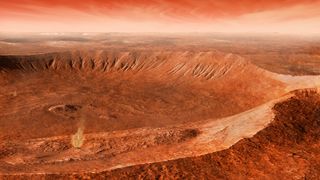
If life is capable of spreading from planet to planet — a concept known as " panspermia " — then we might be able to detect it, even if we don't know what we're looking for, new research suggests.
Astronomers are on the hunt for life beyond Earth . While there are several promising locations within the solar system , the sheer abundance of exoplanets means that we are most likely to find life on a planet orbiting another star. There are more than 5,000 confirmed exoplanets to date, according to NASA — and that number is always rising.
But these searches for extraterrestrial life currently face one major roadblock: we don't know exactly what we're looking for. We only know of one kind of planet that is definitely capable of hosting life, and we only know of one kind of life — that is, Earth, and the carbon-based life that is found here. But life may, say astronomers, take an astonishing number of forms throughout the galaxy. While we might get extremely lucky and find an exact replica of Earth with the exact same kind of life, we are more likely to face fuzzy, unclear, nuanced situations that will take many years to unravel.
A pair of astronomers recently proposed an alternative to this approach, focusing less on what life would look like and more on what life would do. Specifically, they propose a detection strategy based on the concept of panspermia, the idea that life can start on one planet and spread to others by hitching rides on meteorites.
Related: What's the best evidence we've found for alien life?
While panspermia lies outside the mainstream of scientific research, it's not outright pseudo-science either. Martian meteorites have been found on Earth , and scientists are routinely surprised by the hardiness of living creatures and the extreme environments that they can survive in.
One of the key characteristics of any kind of life is its ability to change a planet's natural balance. On Earth, for example, we have far more oxygen in our atmosphere than we would were life not present, and distant observers would notice much more green on our landmasses than there would be otherwise.
Sign up for the Live Science daily newsletter now
Get the world’s most fascinating discoveries delivered straight to your inbox.
We don't know exactly what properties of an exoplanet that alien life would change — but, if that life is capable of panspermia, then it would attempt to make those same changes on every planet it came across as it spread from world to world. Sometimes it would fail, if the conditions weren't right to allow that life to thrive — but sometimes it would succeed, making the new planet similar to its original world. Then that new planet would serve as a starting point for a new round of panspermia.
In their new study, the researchers devised a statistical test where, if we measure enough properties of enough planets, then we can potentially identify a cluster of nearby planets that share similar characteristics. Since these planets, each one orbiting a distant star, would have no other reason to be similar to each other, this cluster would stand out from the collection of all exoplanets .
Such a discovery wouldn't be a smoking gun for identifying life, but it would be a major clue that something strange was happening to those planets — and that the cause just might be life propagating among the stars.
— Seismic reading linked to 'alien technology' by Harvard professor likely came from a passing truck, study claims
— US government wanted to reverse-engineer alien ships — but never found any, Pentagon UFO report reveals
— World's 1st 'conversation' between humans and whales could help us talk to aliens someday, scientists claim
The researchers acknowledged that their work has limitations. Firstly, it assumes that panspermia is possible, which is an untested hypothesis. Secondly, their technique only works if we can collect enough data about a large number of exoplanets. But the advantage of their technique is that it's "agnostic," meaning that it can identify a potential signature of life without any bigger assumptions about how that life works.
The team's research was published in March to the preprint database arXiv, and has not yet been peer reviewed.
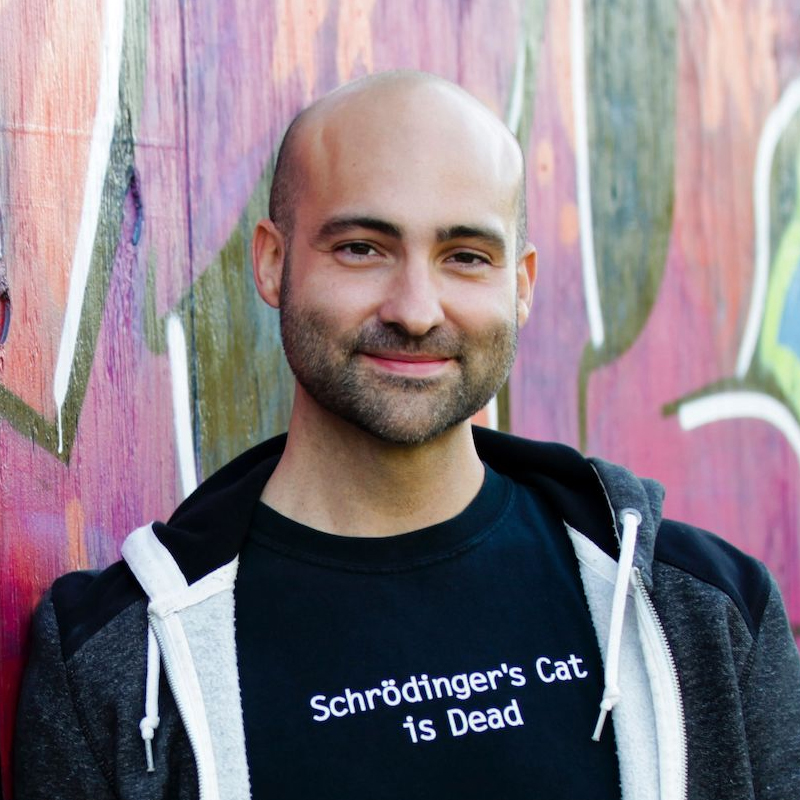
Paul M. Sutter is a research professor in astrophysics at SUNY Stony Brook University and the Flatiron Institute in New York City. He regularly appears on TV and podcasts, including "Ask a Spaceman." He is the author of two books, "Your Place in the Universe" and "How to Die in Space," and is a regular contributor to Space.com, Live Science, and more. Paul received his PhD in Physics from the University of Illinois at Urbana-Champaign in 2011, and spent three years at the Paris Institute of Astrophysics, followed by a research fellowship in Trieste, Italy.
Purple bacteria could be key to finding extraterrestrial life on exoplanets
Seismic reading linked to 'alien technology' by Harvard professor likely came from a passing truck, study claims
How common chemicals — including those in bed sheets — can boost eczema risk
admin said: A fringe theory called "panspermia" suggests that lifeforms can spread to new planets by hitching rides on meteors. New research lays out a roadmap for finding where these hypothetical, planet-hopping aliens may reside. Aliens may be hitching rides on meteors to colonize the cosmos, study suggests. Here's how we could spot them. : Read more
- Bonzadog Too much pure speculation is being treated as factual information. this seems to be happening a lot. More scientific methodology is needed. Reply
- Robert Lohman I don't think a panspermia theory needs to posit intentional action by intelligent aliens. On earth,life on the prokaryotic level can remain dormant for a ridiculously long time. The odds against such life being launched into space in ejecta and landing on a suitable planet may be very long,but given enough time and a vast universe,it may happen. Reply
TheBox said: Communication between ourselves and them is difficult because of wave-function collapse over a huge distance and that is why the more evolved humanised feral's use E.S.P because the mind is much faster than the light .
veggiedude said: Thoughts are not faster than light. Electrical impulses through the brain has to travel through matter, so thought will be impeded by some resistance - it will be much slower.
- View All 5 Comments
Most Popular
- 2 James Webb telescope confirms there is something seriously wrong with our understanding of the universe
- 3 Giant, 82-foot lizard fish discovered on UK beach could be largest marine reptile ever found
- 4 Scientists discover once-in-a-billion-year event — 2 lifeforms merging to create a new cell part
- 5 George Washington's stash of centuries-old cherries found hidden under Mount Vernon floor
- 2 Why does striking flint against steel start a fire?
- 3 Tweak to Schrödinger's cat equation could unite Einstein's relativity and quantum mechanics, study hints
- 4 32 times lasers revealed hidden forts and settlements from centuries ago
- 5 New UTI vaccine wards off infection for years, early studies suggest
Biogeographical Modeling of Alien Worlds
Affiliations.
- 1 Science Department, Carlton le Willows Academy, Nottingham, United Kingdom.
- 2 Division of Epidemiology, The New York State Psychiatric Institute at Columbia University, New York Psychiatric Institute, New York, USA.
- PMID: 33904766
- DOI: 10.1089/ast.2020.2304
In this article, we partially quantify the biological potential of an exoplanet. We employ a variety of biogeographical analyses, placing biological evolution in the context of the geological evolution of the planet as a whole. Terrestrial (as in Earthly) biodiversity is tightly constrained in terms of species richness by its environment. An organism's habitable environment may be considered its niche space or hypervolume in terms of the physical characteristics in which that organism can survive and reproduce. This fundamental niche forms the broader space in which the organism realizes its true niche in terms of its interactions with other species. Many of the physical characteristics can be determined from astrophysical constraints and are thus amenable for dissection. However, the geographical space that organisms occupy is driven by the geological evolution of a sizable telluric planet. In turn, this is driven by the progressive differentiation of its interior to produce increasingly felsic crust. Using a variety of available models, we can then constrain the available space that species can inhabit using species-area relationships. By considering a combination of astrophysical constraints and geographical space, we partially quantify the numbers of species that can inhabit the landscape that geology provides. Finally, we also identify a correlation between geomorphological scale and speciation, which, if validated, will allow further dissection of species diversity on alien worlds.
Keywords: Allopatric speciation; Continental growth; Geomorphology; Niche space; Species richness; Super-Earth; Synchronous rotation.
- Biodiversity*
- Introduced Species*
AIR & SPACE MAGAZINE
The astrobiology of alien worlds.
A comprehensive review of life as we know it—and may not know it.
/https://tf-cmsv2-smithsonianmag-media.s3.amazonaws.com/accounts/headshot/Dirk-Schulze-Makuch-headshot.jpg)
Dirk Schulze-Makuch
/https://tf-cmsv2-smithsonianmag-media.s3.amazonaws.com/filer/4d/d8/4dd8a654-259f-4dd5-bc3a-737c242b4604/slime_mold.jpg)
In a new paper just published in the journal Universe , Louis Irwin and I attempt to sum up scientists’ current understanding of life “as we know it,” and speculate how life may look and function on alien planets and moons. That includes potential biospheres very different from our own, such as a rocky planet with an ice-covered global ocean. Or it might be a barren planet devoid of surface liquids, or a frigid world with abundant liquid hydrocarbons. It could even be a rogue planet with no “host” star, a tidally locked planet, or a so-called Super-Earth . Maybe the biosphere exists only in the planet’s atmosphere.
In all those places, life could thrive—maybe not with the diversity and amount of biomass we have on Earth, but still with the ability to persist. One fascinating analog for exotic organisms on an alien world are slime molds. Here on Earth, under certain conditions, they aggregate into acellular, multinucleate bags of protoplasm, with an indefinite shape and the ability to move using a process called cytoplasmic streaming . They reproduce sexually (they have several different mating types), but in the multinucleate unicellular state, they can break apart into unequal fragments that can remerge back into a single organism.
Our paper goes into detail on how biochemistry could be different on an alien planet. In a subsurface ocean like the one on Jupiter’s moon Europa , for example, an entire large biosphere could draw energy from ionic or osmotic gradients rather than sunlight. On some other world, information could be passed from generation to generation by the alignment of magnets or dust particles rather than DNA. On others, organic beings may have yielded to mechanical or robotic life.
The rise of mechanical life may be particularly relevant, in fact. Our own terrestrial civilization will soon have (and others may already have) the capability to fabricate intelligent mechanical forms of life so sophisticated that their manufacture can be carried out robotically under the control of advanced forms of artificial intelligence. This would represent, for all practical purposes, autonomous reproduction. At that point, the distinction between organic life and mechanical life ceases to be important.
The rise of mechanical life might enhance contact with alien life forms, because travel through space would be easier to accomplish robotically than with organic bodies. Such contact is still statistically improbable given the immense distances from Earth to the nearest plausible home for life, the apparent insignificance of Earth on a cosmic scale, and the extremely narrow window for two civilizations to exchange and understand signals using any one communication technology, since technology is always evolving.
This, by the way, is our explanation for the Fermi Paradox or Great Silence . Still, we conclude that it is extremely likely that alien life is out there. And we’re betting that when we finally discover it, we will be surprised how different it really is.
Get the latest stories in your inbox every weekday.
/https://tf-cmsv2-smithsonianmag-media.s3.amazonaws.com/accounts/headshot/Dirk-Schulze-Makuch-headshot.jpg)
Dirk Schulze-Makuch | | READ MORE
Dirk Schulze-Makuch is a Professor at the Technical University Berlin, Germany, and an Adjunct Professor at Arizona State University and Washington State University. He has published eight books and nearly 200 scientific papers related to astrobiology and planetary habitability. His latest books are The Cosmic Zoo: Complex Life on Many Worlds and the 3rd edition of Life in the Universe: Expectations and Constraints. Website
- Share full article

How a Harvard Professor Became the World’s Leading Alien Hunter
Avi Loeb’s single-minded search for extraterrestrial life has made him the most famous practicing astronomer in the country — and possibly the most controversial.
Credit... Michael Marcelle for The New York Times
Supported by
By Seth Fletcher
- Aug. 24, 2023
On Oct. 19, 2017, a telescope in Maui detected something that had entered our solar system from elsewhere in the galaxy. Astronomers named it Oumuamua, Hawaiian for “scout” or “messenger,” because it was the first interstellar object they had ever recorded — the only known traveler to have crossed the vast distance between another star system and our own. Where it came from was only part of its mystery. Oumuamua fit none of the well-understood astronomical categories. If it was a rock — an asteroid — it was an extremely strange one. Researchers estimated that it was at least the length of a football field; its shape was hard to determine, but it seemed to be long and thin, like a cigar. “No known objects in the Solar System have such extreme dimensions,” wrote the group of astronomers who discovered the object.
Listen to This Article
The more that scientists studied Oumuamua, the weirder it seemed. Analysis of its trajectory showed that, in the weeks before its detection, Oumuamua sped up as it approached the sun, and its acceleration couldn’t be explained by the sun’s gravity alone. That extra kick would be normal for a comet. Comets are rocky snowballs, and when they get close to the sun, ice within them turns to vapor, releasing gas and giving them a boost. But Oumuamua lacked a comet’s signature tail, and none of the telescopes that observed it detected water vapor, carbon monoxide or other telltale signs of sublimating ice. Scientists started inventing wild ideas to explain Oumuamua’s observed characteristics, things like hydrogen icebergs and gigantic dust bunnies less dense than air. They were reaching.
Avi Loeb, a theoretical astrophysicist at Harvard University, followed the news about Oumuamua for months. Then one morning in the fall of 2018, he had an idea. For Oumuamua to accelerate as it did, something had to have given it a push. What if that thing was sunlight? For years, scientists have theorized that sunlight, properly captured in the vacuum of space, could exert enough force to boost an object to incredible speeds. Nature doesn’t make anything that harnesses light quite so well, but Loeb thought he might have the answer. “One possibility,” he and a postdoctoral researcher wrote in a paper, “is that Oumuamua is a light sail.” Light sails have long been proposed as a method of space travel, though as of now they are mostly hypothetical. (Japan’s space agency successfully tested one in 2010.) The idea is that a superthin metallic sheet could catch sunlight the way a ship’s sail catches wind, propelling a craft through space. Loeb’s hypothesis could explain some of Oumuamua’s strange behavior, but if he was right, it meant the object was not a natural phenomenon. It was an extraterrestrial artifact.
Loeb was known in the scientific community for his openness to unconventional ideas, but he was an establishment figure who had published hundreds of papers over three decades on traditional astronomical subjects. He had a reputation for finding creative ways to subject hard-to-study phenomena to the rigors of the scientific method. “Avi is very good at picking problems to work on that have testable results,” Robert Wilson, a Nobel Prize winner in physics, told The Times in 2014. By the time Loeb published his Oumuamua hypothesis, he had collected a stack of impressive titles at Harvard: chairman of the astronomy department, director of the Institute for Theory and Computation, director of the Black Hole Initiative. Loeb could not have been any more mainstream or credentialed, yet here he was, saying that maybe an alien spaceship had arrived. It only took a few days for camera crews to show up at his house.
Since then, Loeb has made extraterrestrial life his primary research focus. In thousands of news media appearances and near-daily online essays, he has called for scientists to seriously consider the possibility that aliens, or hardware they built, have visited our planet. He says scientists have a responsibility to investigate astronomical oddities like Oumuamua as well as reported sightings of U.F.O.s, which have recently been rebranded as U.A.P., for unidentified anomalous phenomena. “Two-thirds of the American public believes there is extraterrestrial life, more than the 56 percent that believes in the God of the Bible,” Loeb told me. Dismissing their questions as unworthy of consideration, he argues, is not a good way to earn back the trust of an American public that has become skeptical of science and scientists.

In 2021, with funding from private donors, Loeb co-founded the Galileo Project, a research program at the Harvard-Smithsonian Center for Astrophysics dedicated to the search for alien technology near and on Earth. Its aim is to bring the scientific method to the realm of eyewitness testimonies, grainy Polaroids and shady former military guys who end every debate by saying “that’s classified.”
Loeb is far from alone in hypothesizing that the universe could be filled with life. Astronomers now estimate that the Milky Way is home to 100 billion planets, a couple billion of which are similar to our own. It’s not particularly controversial to posit that some of these planets may be home to civilizations more intelligent than we are. Because microbial life emerged soon after Earth cooled and most star systems are billions of years older than our sun, it’s reasonable to imagine that life in other star systems could have begun evolving eons before the first protobiological goo formed here. Where Loeb departs from almost all of his colleagues is in thinking that aliens on other planets could have already made their way to us.
Loeb maintains that looking for alien spacecraft is less speculative than a lot of mainstream science. His go-to foil is fundamental physics. Since the discovery of the Higgs boson particle more than a decade ago, the multibillion-dollar particle colliders that physicists built to find postulated forces and fields have mostly come up empty, and still, Loeb says, scientists believe with quasi-religious faith that if they just build even bigger colliders, their theories will be redeemed. He reserves most of his scorn for string theorists, who, after assembling a theory of nature based on tiny hypothetical entities, have spent decades postulating extra dimensions and parallel universes trying to make the math work. These same people, Loeb claims, refuse to consider anomalies like unexplainable interstellar objects. Out of fear or intellectual rigidity, these scientists have retreated into their own minds while ignoring strange phenomena in the real world.
Loeb’s outspokenness about extraterrestrial life has made him the most famous practicing astronomer in the country. His 2021 book about Oumuamua, “Extraterrestrial,” debuted on The Times’s nonfiction best-seller list; a new book, “Interstellar,” which presents contact with extraterrestrials as the next big step on humankind’s evolutionary ladder, comes out this month. He has become the sort of academic star who gets invited to Richard Branson’s private island and other exclusive gatherings of rich and powerful patrons interested in heterodox ideas. He is being followed by a Netflix documentary crew.
Yet many in his own field consider Loeb a pariah. His more polite critics say that he is distracting from the horizon-expanding discoveries astronomers are making with new instruments like the James Webb Space Telescope. The more outspoken ones accuse Loeb of abandoning the scientific method and misleading the public in pursuit of fame. Loeb says he gets attacked from both sides: by his colleagues in the mainstream and by the U.A.P. “crazies” who get upset when he dismisses their most ridiculous theories by pointing to the laws of physics. He sometimes talks about himself as a martyr. “I’m putting my body on the barbed wire,” he told me.
One morning in January, I visited Loeb at his three-story clapboard house in the wealthy, historic suburb of Lexington, Mass. He was working from home but wearing a tailored suit, as he does most days. At 61, he is energetic and trim from a low-carb diet and a morning jogging routine that he often mentions in his emails and essays — the predawn ritual where the day’s ideas arrive. He offered me a bowl of blueberries and an enormous cup of coffee, which he says he doesn’t drink because it would amplify his already manic pace of speaking. After talking for a couple of hours in a tidy front sitting room, he drove us to see an installation built to fulfill one of the Galileo Project’s main goals: getting a high-resolution image of a U.A.P.
Students and volunteers assembled the Galileo Project’s first “observatory” on the roof of the Harvard-Smithsonian Center for Astrophysics, but they recently moved it to university-owned land in the Boston suburbs. He asked me not to identify the location because he was worried about “hackers” disrupting or stealing the equipment. After a while, we pulled into a wooded area, parked by a stand of conifers and walked across a snowy lawn to what looked like a high-tech Boy Scout weather station. Metal antennas stood on tripods. Eight infrared cameras were embedded within a synthetic dome the size of a charcoal grill, staring at the sky. There were visible-light cameras, ultrasensitive microphones, spectrum analyzers and other sensors, including a Geiger counter, all of it connected to the cloud, where machine-learning algorithms would scan the data for anything unusual. It was, in a sense, an elaborate wildlife camera for aliens.
Astronomers have been conducting the Search for Extraterrestrial Intelligence (SETI) since 1960, using telescopes to watch for signals from space. NASA scientists are drawing up plans to look for primitive life on certain moons of Jupiter and Saturn. Astrobiologists talk about searching for artificial light or industrial pollution on planets orbiting other stars. None of these endeavors carry stigma because they assume that, if life is out there, it’s out there . The boundary between mainstream astrobiology and the fringe is the idea that extraterrestrials have crossed the abyss of space to come see us — and that the governments of the world have somehow kept the evidence a secret. But governments do keep secrets, and secrecy has long fueled conspiratorial thinking about aliens and U.A.P.s. Leaked videos of unidentified objects taken by cameras on fighter jets are hard to make sense of, in part because those cameras are classified. The idea behind Loeb’s observatory is to start building a library of unclassified data that scientists can use to study U.A.P.s.
Loeb told me that he has always had a speculative disposition. As a kid growing up on a farm in Israel, he had an uncomfortably hyperactive mind. “It was like flies buzzing around in a metal box, bumping up against the wall,” he said. He wanted to be a philosopher or a writer, but compulsory military service led him to science. In the Israeli Defense Forces’ elite Talpiot program for academically promising recruits, Loeb studied physics and mathematics while learning to drive tanks and jump out of planes. In graduate school he did research on weaponizing electromagnetic fields and plasmas before moving to the United States for a postdoctoral position in astrophysics at the Institute for Advanced Study in Princeton. Starting at Harvard in the early 1990s, he published prolifically on subjects such as infant black holes, the large-scale structure of the cosmos and the universe’s first stars.
In 2015, Yuri Milner, a billionaire Silicon Valley investor and philanthropist, showed up at Harvard hoping Loeb could figure out how to send a probe to another star during his lifetime. Loeb was game to try. A year later he was standing on top of One World Trade Center alongside Milner and Stephen Hawking announcing Breakthrough Starshot, a plan to attach tiny probes to micrometer-size sheets of reflective material — light sails — and blast them with ground-based lasers, propelling them to Alpha Centauri in a couple of decades. Breakthrough Starshot, which is still in an early research phase, was what got Loeb thinking seriously about the mechanics of interstellar travel.
Right around the same time that Oumuamua appeared in the sky, in a cosmic coincidence, the U.S. government started talking openly about U.A.P.s. It began on Dec. 16, 2017, with a story in The New York Times that revealed the existence of a shadowy military U.A.P. research program called the Advanced Aerospace Threat Identification Program. In an accompanying article, two Navy pilots described a mystifying 2004 encounter with a flying object off the coast of San Diego: an oval-shaped craft that appeared to hover 50 feet above the frothing ocean surface before bolting out of sight. More reported sightings of unidentified phenomena soon went public. In a 2019 Times article, Lt. Ryan Graves, a Navy pilot, described repeated encounters with unexplained aircraft off the East Coast of the United States. “These things would be out there all day,” he said. Marco Rubio added language to the Intelligence Authorization Act of 2021 asking the Director of National Intelligence to submit a report to Congress on the subject.
The Princeton astrophysicist Edwin Turner, a close friend of Loeb’s, says that during the first few years of this efflorescence of U.A.P. interest, they both watched with skeptical curiosity. “Our conversation about U.A.P. was very much along the lines of, Who knows, it’s not obvious,” he said. “It didn’t seem plausible that there were extraterrestrials visiting the earth.” What made Turner think U.A.P.s were worth investigating, he said, was the report that the Office of the Director of National Intelligence delivered to Congress in June 2021. The nine-page document described the “threat posed by unidentified aerial phenomena,” which included a “handful” of U.A.P. that “appeared to remain stationary in winds aloft, move against the wind, maneuver abruptly, or move at considerable speed, without discernible means of propulsion.” Loeb came across an interview in which Bill Nelson, the NASA administrator and former U.S. senator from Florida, said that he saw classified material while serving in Congress that made the hair on the back of his neck stand up. “Now, I don’t know how frequently the hair stands up on the back of Bill Nelson’s neck,” Loeb told me. “But to me it was intriguing.”
If Loeb’s mother had been around at that point, he said, she would have tried to dissuade him from his late-career turn toward alien hunting. “She would say, ‘Why would you give up on everything you accomplished?’” Loeb has described his mother, Sara, as an “interrupted intellectual” whose family pulled her from college in Bulgaria to move to Israel upon its founding. When he and his two sisters were old enough, she continued her studies, and in Loeb’s adolescent years she took him along to college philosophy classes. They were very close; until her death in 2019, they spoke on the phone nearly every day. “I sort of realized on a personal level that, up until that point, I tried to make everyone happy,” he said. “After my parents passed away, I said: ‘The hell with it, I’ll focus on substance. I don’t care how many people like me or not like me, I would just do what seems to me is the right thing to do.’” Criticism from other astronomers only hardened his commitment. “The more pushback that I got,” he said, “the more appropriate it looked to me.”
Mainstream scientists might have been distancing themselves, but Loeb was discovering a different world of allies, fans and patrons. The newly revealed government interest in U.A.P.s got wealthy people wondering how to invest in the search for alien life. That led them, naturally, to Loeb. “I started getting money without soliciting it,” he told me. In May 2021, the Harvard astronomy-department administrator told Loeb that an anonymous donor had given him $200,000 in research funding. Within a few days, they determined that it came from a wealthy software engineer named Eugene Jhong. Loeb arranged a Zoom call with Jhong and got another $1 million. Around the same time, Frank Laukien, the chief executive of the scientific-instrument manufacturer Bruker, who had read Loeb’s book “Extraterrestrial,” showed up on his front porch in Lexington. Together they decided to establish the Galileo Project.
The observatory near Boston had been running for several months, and they were still training the machine-learning algorithms to identify birds, planes and other common airborne objects. The goal is to install up to 100 such observatories around the world; so far Loeb has obtained funding to install five more stations in the United States. While the dream is to get the first megapixel-quality photo of something anomalous, he says he expects almost everything these instruments detect to be mundane. “The Galileo Project is completely agnostic, has no expectations,” he told me. I asked him how an experiment like this could ever deliver a convincing negative result. A failure to photograph a U.A.P. would never convince a believer that there are no alien ships in the sky, only that the aliens were smart enough to avoid Loeb’s camera trap. “If we search the sky for five years, 24/7, and see nothing unusual except for birds and drones and airplanes, and we do it at tens of different locations, maybe 100 locations,” he said, “then we move on.”
The week after Loeb showed me the observatory, I joined a planning meeting for another Galileo Project initiative — an effort to retrieve an unusual meteorite that had fallen to Earth. Several years ago, Amir Siraj, a Harvard undergraduate working with Loeb, identified a curious entry in a government meteor database: On Jan. 8, 2014, an object exploded near Papua New Guinea. Its orbit suggested an origin outside our solar system, though it was impossible to say for sure because the government satellites that detected it were classified. In 2022, after a lot of prodding from Loeb, the U.S. Space Command released a letter saying with “99.999 percent confidence” that the Papua New Guinea fireball was interstellar. The government also published the meteor’s light curve, a graph of its brightness over time. From this, Loeb concluded that it had exploded so close to the Earth’s surface that it must have been made of something much harder than normal meteors, maybe even an artificial alloy like stainless steel. Which made him wonder: What if it was an extraterrestrial probe? And could he find its remains?
If anything was left of this meteor, or extraterrestrial probe, it was scattered across the seafloor north of Papua New Guinea. When meteors burn up in the atmosphere, the molten remains condense into sand-grain-size orbs called spherules that cascade to earth like glitter. The logistics of searching for those spherules under several thousand feet of water were daunting, but there was reason to think it could be done. In 2018, scientists used remotely operated vehicles and a “magnetic rake” to find spherules from a meteor that had fallen off the coast of Washington. Encouraged by that project, Loeb and Siraj started thinking about going after the Papua New Guinea meteorite. Charles Hoskinson, a mathematician who made a fortune in cryptocurrency, heard Loeb talking about the meteor on a podcast and pledged $1.5 million for the search. To figure out the logistics, they hired EYOS Expeditions, the company that helped the director James Cameron dive to the Pacific Ocean’s 36,000-foot-deep Mariana Trench. They planned to go to sea later in the spring.
Loeb ran the planning meeting on Zoom from his home office. He started by telling the group about a conversation he just had with Hoskinson, whose $1.5 million donation was, until that week, an undeposited promise. Loeb met with him two days earlier to get a final commitment. “It was quite nerve-racking,” Loeb said. Hoskinson asked hard questions about their chances of success. The plan was to drag a magnet-studded sled along the seafloor to collect the spherules. But what if the remains weren’t magnetic? Loeb explained that they would have nets attached to the sled to catch particles the magnets missed. Hoskinson was not reassured. Could they do better than nets? Could they dredge, or use some sort of sluicing device? To appease Hoskinson, Loeb agreed that the magnet-sled engineers would design a supplementary sluicing device. Loeb got the go-ahead; he told the group that the money would soon be transferred to the expedition’s bank account. Still, Loeb seemed a little shaken by the difficulty of closing the deal. “Anyone who knows me knows that I’m task-oriented rather than promise-oriented,” he said. It was as if he was only then internalizing the idea that private donors expect a return on their investment. “The nightmare scenario is that we go there and find only muck.”
The first step in avoiding the nightmare scenario was to search the right area, and that would be difficult enough. The location data they had for the meteor came from military satellites that watch for incoming nuclear missiles. Every measurement on every instrument has some margin of error, which is why a huge amount of scientific work involves calibrating instruments and working with uncertainty. But the margin of error for the missile-spotting satellites was classified. If they relied solely on government data, they would be left searching 50 square miles with a magnetic sled smaller than a golf cart. Loeb told the group that he and Siraj thought they had found a way to shrink the search area using seismometer readings and a lot of math. Even so, Rob McCallum, the cheerful New Zealander who runs EYOS, later conceded to me that the hunt would be challenging. “The complexity lies in the fact that no one knows what it is we’re looking for,” he said. “We assume that it’s a few shovels full of small black particulate, spread over a mile, two miles, 10 miles.” The expedition would depend to an unusual degree on luck.
Loeb’s theory about Oumuamua — the baffling interstellar object that set him on his late-career course as an alien hunter — was always controversial among his colleagues, and not just because it invoked alien technology. Karen Meech, an astronomer at the University of Hawaii and the lead author on the Oumuamua discovery study, had a long list of complaints about Loeb’s paper. First was its rhetorical mode. “I would have been OK with that first paper had at the very end they said: ‘This is puzzling, we don’t have a very good explanation. Let’s speculate on what would it take to provide enough evidence for us to go the route of extraterrestrials.’” Instead, she said, Loeb’s case consisted of “I’m just declaring that it is with no evidence whatsoever.” She pointed out that Oumuamua’s light curve showed that it was wobbling like a top. “I’m not a sailor, but you’ve got to keep the sail pointing in the correct direction,” she said.
Beyond that, Loeb’s critics argued that his proposal was physically unrealistic. Even if Oumuamua was made of the least dense artificial solid material known to humans, it would still be roughly 10 times denser than his math required and was preposterously slow for an interstellar spacecraft. “You don’t leap to ‘it’s alien technology’ before you’ve exhausted everything thoroughly,” Meech said, “and I get the feeling that Avi’s so excited about his ideas that he picks out bits of the observations that fit and discounts the others that do not.” She continued, “That’s what we’re trying not to teach young students to do, because that’s not science.”
In late March, as Loeb was preparing for his trip to the South Pacific, two American scientists published a paper in Nature proposing a new, natural explanation for Oumuamua. The study described how a small comet that had spent eons drifting among the stars could become something like Oumuamua and how, in the little time they had available to study it, astronomers wouldn’t have been able to figure that out. It was written by Jennifer Bergner, an astrochemist at the University of California, Berkeley, and Darryl Seligman, a postdoctoral researcher in astrophysics at Cornell University. Together they made the following case: In the unimaginable cold of interstellar space, the ice in a comet takes on a fluffy, amorphous form. When this porous ice is cooked by radiation, which happens continuously in the cold furnace between stars, bubbles of hydrogen form inside. As Oumuamua got close to the sun, the warmth rearranged the molecules in the ice, releasing some of the trapped hydrogen. The escaping gas gave Oumuamua a shove, causing the unexplained acceleration.
Headlines around the world declared the mystery of Oumuamua all but solved. In a news release from the University of California, Berkeley, Seligman said, “We had all these stupid ideas, like hydrogen icebergs and other crazy things, and it’s just the most generic explanation. ”
Under the norms of polite academic discourse, if Loeb disagreed with Bergner and Seligman, he would have welcomed their contribution and promised to explain his objections in due course in a peer-reviewed journal article. Instead, Loeb went on the attack. The day after the paper came out, he emailed me saying he found an error in their work. Loeb accused Bergner and Seligman of ignoring the cooling effect of evaporating hydrogen in their model, a grave mistake that he said dominoed through the rest of their calculations and made their proposal untenable. He asked Nature to correct the original paper; they declined to do so. He wrote to The Times, The Daily Beast and The Times of London and other outlets asking them to correct their stories about the study. When The Times of London agreed to look into Loeb’s objection, he forwarded me the exchange, adding, “The Brits have integrity!”
Bergner and Seligman declined to respond on the record to Loeb’s critique of their paper, which he has continued to make loudly and often; it even appears on the third page of his new book. These days, few American astronomers want to enter a public debate with Loeb, particularly if they are untenured. So I asked Aurélie Guilbert-Lepoutre, an expert on comets with the French National Center for Scientific Research, to walk me through the problem. She said that Bergner and Seligman’s choice to omit the variable of evaporating hydrogen was reasonable given the lack of laboratory data on hydrogen ice. You could debate it, she told me, but she probably would have done the same. No mathematical model of an astronomical body will ever be perfect: “You have to make assumptions.” Next Guilbert-Lepoutre directed me to an equation in Loeb’s response paper, where, she said, Loeb had made a mistake. When Loeb and his co-author added in hydrogen — a gradually vanishing substance — to their calculations, they should have written the variable to evolve with time. But they kept it static, and that was wrong.
When I ran Guilbert-Lepoutre’s criticism by Loeb, he brushed it off as irrelevant, but she maintained that this error mathematically forces Loeb’s model to produce the low temperatures that contradict Bergner and Seligman. Guilbert-Lepoutre told me that she spent a lot of time going through the Bergner and Seligman study and related data and scientific literature. “And then I went to Avi’s paper, and I was like, ‘Jeez, I just lost two hours, because that’s so stupid,’” she said. “That’s a stupid mistake.”
What should the field of astronomy do with someone like Avi Loeb? David Spergel, the astrophysicist leading a NASA study group on U.A.P.s, told me that Loeb was too quick to jump to exciting conclusions and that he wished he would be more careful in his statements to the news media but that he saw value in his work. “There’s a lot of elements in the Galileo Project that look like a good approach to what I view as high-risk, high-return science,” he said. Loeb is known to be kind and nurturing to his students and postdocs, but the number of scientists who refuse to talk to the media about him is telling. “So many of my colleagues would just prefer to ignore him until he makes an ass of himself and goes away,” the Arizona State University astrophysicist Steve Desch told me. “But he has a high threshold for shame.”
The more time Loeb has spent in the scientific borderlands, the more speculative his ideas have become. One day when we were talking in his office, he started considering the possibility that an ancient Martian civilization might have terraformed Earth a billion years ago. We would never see the evidence on Earth, he said, because meteorite bombardments and plate tectonics would have destroyed it all, but there could still be archaeological remains on Mars, and we could always look for them. In March, he and Sean Kirkpatrick, director of the Pentagon’s new office coordinating a “whole of government” approach to demystifying reports of unidentified objects, wrote a paper claiming that alien “motherships” could be lurking in our solar system and sending “dandelion seed” probes to explore Earth.
One of Loeb’s sayings is an inversion of Carl Sagan’s famous dictum, “Extraordinary claims require extraordinary evidence.” Loeb believes that the discovery of alien life would be so important that even the tiniest possibility of success justifies a tremendous investment in research. “Avi says extraordinary evidence” — like, say, widespread reports of unexplained flying objects — “requires extraordinary funding,” Turner, the Princeton astrophysicist, told me. Turner is an active member of the Galileo Project, though he has a reputation as the house skeptic. “We’re not mounting scientific investigations into ghost stories, right?” Turner said. “There has to be some level of skepticism where you say, ‘That’s just too outlandish to be worth our time investigating.’”
Loeb often speaks dismissively of unnamed “administrators” or “colleagues” who refuse to see the interstellar light. His sense of being slighted, dismissed or overlooked bubbles up frequently and spontaneously. If you get him talking for more than an hour or so, invariably his mood turns dark, his eyes narrow and he starts listing resentments and perceived injuries. He sometimes describes the Galileo Project as a direct response to insult. “The Galileo Project was in a way a substitute to NASA not acting on my white paper,” he told me. In 2021, Loeb sent Thomas Zurbuchen, a NASA administrator, a paper proposing a working group to study U.A.P.s. He says he never heard back. The next year, Loeb was celebrating his 60th birthday with some former students and postdocs on Martha’s Vineyard when someone emailed him saying that NASA had convened the U.A.P. study group led by Spergel. He hadn’t been invited. “I wrote an email to Thomas Zurbuchen basically saying, ‘This is really surprising, and you basically ruined my week,’” he said. “ ‘I had a celebration here, and now I learned that you decided to establish this study.’”
Some of Loeb’s grievances go back decades, to well before the alien-hunting began. In our first meeting, he brought up an arcane mathematical link between the masses of supermassive black holes and the velocities of stars within their host galaxies — the sort of correspondence that astronomers detect in data and then generalize into a formula. He said that he mentioned this possible cosmic relationship at a conference in 2000, “and it was immediately dismissed by the experts in the audience.” Soon thereafter, two young scientists, Laura Ferrarese and Karl Gebhardt, came to Harvard for job interviews. Each was studying galaxies at the time with data from the Hubble Space Telescope. In those interviews, Loeb encouraged them to look in their data for evidence of this link between black holes and stars. They did. It checked out. They each published landmark studies on what became known as the M-sigma relation for black holes — a big discovery. With Loeb’s agreement, they put him in the acknowledgments. Now, 23 years later, Loeb was ruefully telling me: “Look, I suggested it in that conference, I could have written it myself, I was completely discouraged from pursuing it by the people at the conference. And then I suggested it to these young people just to say, ‘Look, you can check it and then let me know, and we will write together the paper.’ And then it ended up in a different way.” On a document linked to his Center for Astrophysics website, the M-sigma relation for black holes is No. 11 on a list of Loeb’s “Top 20 Confirmed Predictions.”
I contacted Ferrarese and Gebhardt. Both were surprised I was asking about something that happened more than 20 years ago. At the time, Ferrarese said, comparing the mass of a galaxy’s black hole with the velocities of the stars around it was just an obvious thing to do. “I wouldn’t even call it an idea,” she said. Similarly, Gebhardt said he would have done the measurement that confirmed M-sigma anyway, but Loeb’s enthusiasm made him push a little harder, so he acknowledged him in the paper. When I told him that Loeb felt excluded from the discovery, Gebhardt seemed taken aback. He quickly cycled through surprise and disappointment (“I thought we had a really positive exchange with him”) to clarity. “To be blunt, and I would say this to him as well, if he thinks he owns the M-sigma relation, that’s basically nonsense.”
On a Sunday in early June, Loeb boarded Charles Hoskinson’s private jet at a small airport outside Boston for the flight to Papua New Guinea. When I talked to him two days earlier, he sounded paranoid. A whistle-blower named David Grusch had just gone to Congress claiming the government ran a decades-old secret program for studying crashed alien spacecraft. This news made Loeb worry that the U.S. Navy might beat them to the site of the object, which they had named IM1. If the government decided to search the site, Loeb told me, they would be looking for large, intact fragments of a space probe, probably with remotely operated vehicles. On the flight between Australia and Papua New Guinea, they took a detour for a quick aerial inspection of the patch of ocean they were set to trawl. They saw no competitors.
After another short flight, they landed near the port town of Lorengau, where Rob McCallum, the expedition leader, was waiting with their aluminum catamaran, the Silver Star. Some 60 miles north of their launch point, they lowered the magnet sled from a winch on the deck of the ship and started dragging the meteorite’s likely landing path. Over the next six days, they hauled in a manganese-platinum wire, a paint bucket, some nails, shards of shock-resistant steel and lots of volcanic ash. Loeb began to get anxious. They were preparing to use the sluicing sled later that week in case the particles they were looking for weren’t magnetic after all. Then, finally, an expedition team member filtered some volcanic ash through mesh, placed the remains under a microscope and saw a tiny metallic pearl: the first spherule.
As they kept searching, their spherule collection expanded. When they reached a milestone of 50 spherules, they opened a case of Moët & Chandon Champagne they had waiting in a refrigerator. Using the ship’s Starlink connection, Loeb did interviews with podcasters and journalists, declaring victory. His Medium posts grew exuberant and occasionally snide. A post headlined “What a Wonderful World” took a swipe at “a so-called ‘Harvard astrophysicist” who in 2022 told The Harvard Crimson that he didn’t expect Loeb’s efforts to be “terribly scientifically productive.”
Back at home a couple of weeks later, in an interview with NBC, Loeb made bold claims about their discovery. Though it was still unclear whether the spherules came from any meteor, let alone the first interstellar meteor or an alien probe — the planet is covered with spherules produced by processes ranging from volcanism to iron smelting — he told the NBC interviewer that this was “the first time that humans hold material belonging to a big object that came from outside the solar system.” In an expedition summary posted on Medium, he wrote of the possibility of returning to Papua New Guinea to search the site with new equipment. If IM1 was a piece of extraterrestrial technology, Loeb reasoned, maybe the spherules come from the melting of its outer layer; if so, they might trace a path to intact remains.
On Zoom in late July, the day before a conference in Cambridge celebrating the two-year anniversary of the Galileo Project, Loeb was more subdued. An article on the Papua New Guinea expedition was published in The Times earlier that week, and it quoted meteor experts who were highly skeptical of Loeb’s claims. He was still brooding about it. “It’s just unfair,” he told me. “I’m doing the best I can to go to the Pacific Ocean, putting a lot of my time and energy into collecting the materials, just following the scientific method and then doing the analysis with the best instruments. And all they’re doing is sitting in their chairs and saying negative things about me. That’s unfair. And they’re saying I’m distorting the way science is done. I’m just following exactly the scientific method, collecting and analyzing.”
He shared his screen and showed me some of that analysis in progress. First was a map of the suspected IM1 crash site overlaid with a grid of color-coded cells showing the distribution of spherules. “Per unit mass, the number of spherules appears to be correlated with the meteor path,” he said. “That’s interesting, because it says that we didn’t just collect crap.” Next he pulled up some new electron-microscope images of spherules that had been magnified to nearly atomic level. Whether they were from interstellar space or a volcano, they were gorgeous. To figure out where they came from, Galileo Project-affiliated laboratories across the world were measuring the amounts of elements and isotopes within them. Loeb said that analysis should determine first whether the spherules originated in space, and if so, whether they were from our own solar system or elsewhere. He said the labs had been working through a few spherules per day, but that the pace should soon increase, and he hoped to have results within a few weeks.
Before long, though, he came back to the criticism in the Times piece, which he couldn’t shake off. He told me it was strange that people who didn’t have access to the spherules would have such strong opinions about them. “I mean, I’m talking about scientists, who call themselves scientists but yet are not willing to wait until the evidence comes out,” he said. I asked if his critics could have been annoyed that he went straight to the press with such preliminary findings. “OK, just to explain,” he said. “I didn’t go straight to the press. I wrote my essays. I just gave a diary about the expedition, because that’s an unusual opportunity to educate the public about how science is done. I’m just doing it the scientific way.” The scientific way, I suggested, might have been to keep things quiet until the results are peer-reviewed and published. “That’s another way to do it,” he said. “But it was not a crime. I didn’t do a crime.”
Loeb says he doesn’t care what his critics say, but he spends far too much time complaining about them for that to be entirely true. It’s probably more accurate to say that he’s betting that if he’s right, any transgressions against scientific norms and protocols will be forgiven. That’s a sentiment that I heard in various forms even from some of Loeb’s harshest critics. They were tired of Loeb’s antics, his bullying, his delusions, but it was hard not to wonder ... what if? A good scientist can never completely dismiss a nonzero possibility. When I spoke to Karl Gebhardt, one of the astrophysicists who discovered the M-sigma relation, he told me wearily that he wished the news media would stop indulging Loeb’s over-the-top ideas and let the field get back to doing science. Then Gebhardt paused. “Now, that being said, if he finds something, it’s life-changing,” he said. “It will change everything.”
Seth Fletcher is the chief features editor at Scientific American. He last wrote for the magazine about the astronomers who took the first picture of a black hole. Michael Marcelle is a New York-based photographer whose works blurs the line between fantasy and reality. His forthcoming second book is called “The Living End” and focuses on American spectacle and oblivion.
An earlier version of this article misstated how many Earth-like planets astronomers have found around sunlike stars. Though astronomers’ ongoing census of planets suggests that potentially habitable worlds are common, so far they have found no Earth-like planets.
How we handle corrections
What’s Up in Space and Astronomy
Keep track of things going on in our solar system and all around the universe..
Never miss an eclipse, a meteor shower, a rocket launch or any other 2024 event that’s out of this world with our space and astronomy calendar .
Scientists may have discovered a major flaw in their understanding of dark energy, a mysterious cosmic force . That could be good news for the fate of the universe.
A new set of computer simulations, which take into account the effects of stars moving past our solar system, has effectively made it harder to predict Earth’s future and reconstruct its past.
Dante Lauretta, the planetary scientist who led the OSIRIS-REx mission to retrieve a handful of space dust , discusses his next final frontier.
A nova named T Coronae Borealis lit up the night about 80 years ago. Astronomers say it’s expected to put on another show in the coming months.
Is Pluto a planet? And what is a planet, anyway? Test your knowledge here .
Advertisement
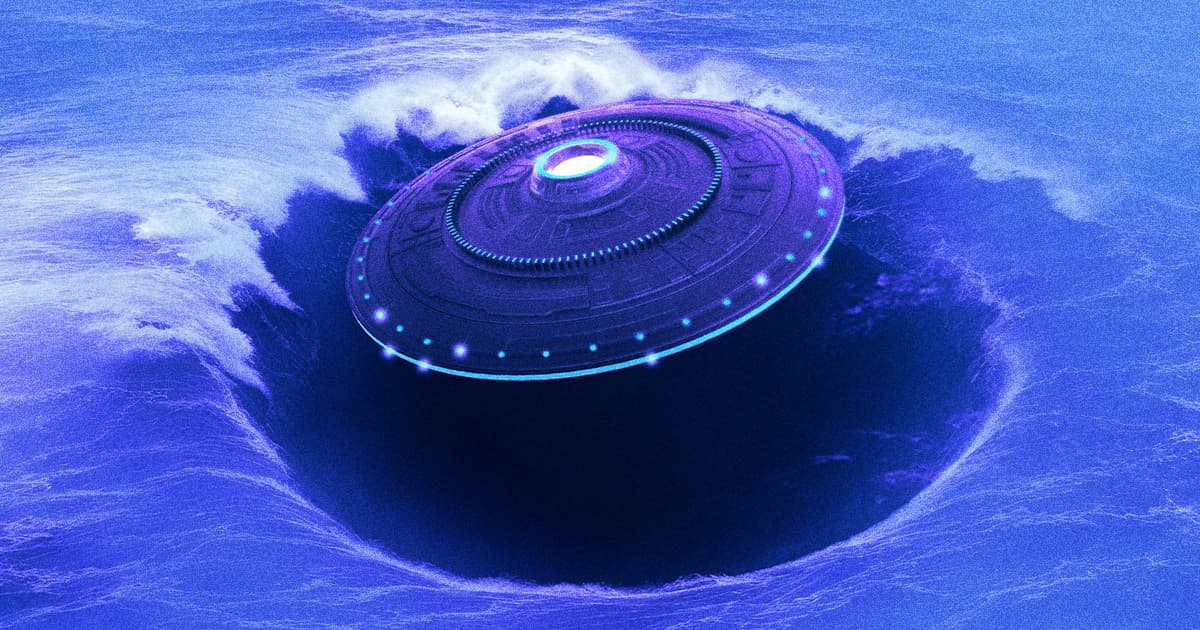
Former Naval Officer Raises Alarm About “World-Changing” Underwater UFO Captured on Video
So-called "unidentified submerged objects" could pose as large a threat as ufos, the former admiral argues..
A report about a strange craft that appeared to defy both aerial and aquatic physics is apparently making a splash among the ex-military set.
Tim Gallaudet, an oceanographer and former Naval rear admiral who served as the author of a March white paper about so-called "unidentified submerged objects" or USOs, told Fox News this week that he considers it both "scientifically valid" and critical to national security to study these phenomena.
Released by the newly-convened Sol Foundation , a think tank dedicated to studying what the military calls "unidentified anomalous phenomena" (UAPs), the 29-page report centers on a 2019 video taken aboard the Omaha off the coast of San Diego.
The video, which was leaked to filmmaker Jeremy Corbell and verified by the Pentagon as a legit instance of Naval-recorded UAP in 2021, raises more questions than it answers — and in both his interview and the Sol Foundation report, Gallaudet suggests that the strange craft should be treated as a threat.
"Pilots, credible observers, and calibrated military instrumentation have recorded objects accelerating at rates and crossing the air-sea interface in ways not possible for anything made by humans," Gallaudet wrote in the think tank's first report.
July 2019: The USS Omaha filmed a UFO #UAP pic.twitter.com/vb0oC10vKR — Dagnum P.I. (@Dagnum_PI) May 6, 2023
While nobody can explain what exactly is going on in the USS Omaha video, the capabilities of the craft it documented could jeopardize American maritime security, Gallaudet told Fox , "which is already weakened by our relative ignorance about the global ocean."
"The fact that unidentified objects with unexplainable characteristics are entering US water space and the [Department of Defense] is not raising a giant red flag is a sign that the government is not sharing all it knows about all-domain anomalous phenomena," the former Naval officer continued, perhaps referencing the Pentagon's UAP-hunting All-domain Anomalous Resolution Office (AARO).
While UFOs generally get most of the attention — be it from the government , academia , or c onspiracy theorists — Gallaudet and others consider USOs to be equally threatening as their flying counterparts, if not more so.
Scot Christenson, the director of the US Naval Institute, wrote in a 2022 editorial for Naval History Magazine that although there has to date been "no documented damage to a plane caused by a UFO," mysterious sea creatures and other USOs "have presented the Navy with the greatest hazard."
While there's a wide gap between little green men and the storied krakens of the deep, the military's main priority when it comes to unidentified objects or creatures in the air or in the sea is safety — and if there's video footage out there of "world-changing" crafts, as Gallaudet put it in his report, that's definitely something that's going to concern the Pentagon .
"To meet the security and scientific challenges," he continued in the Sol Foundation report, "transmedium UAP and USOs should be elevated to national ocean research priorities."
More on the unknown: Whoopi Goldberg Says Aliens Are "Watching Us"
Share This Article
April 15, 2024
10 min read
If Alien Life Is Found, How Should Scientists Break the News?
At a recent workshop, researchers and journalists debated how to announce a potential discovery of extraterrestrial life
By Sarah Scoles
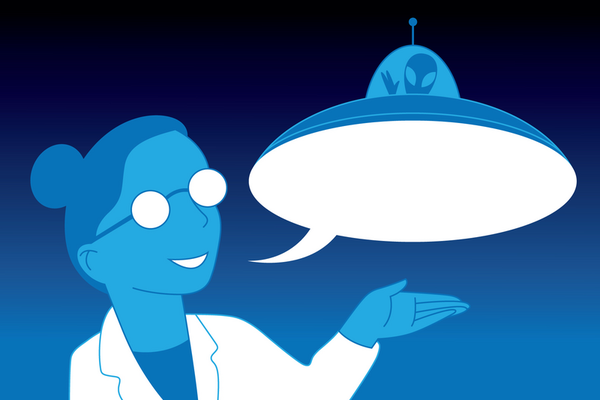
Thomas Fuchs
If one day scientists discover evidence of extraterrestrial life, how will they tell the world ? How certain will they be of their discovery, and how will the public know what sense to make of it? Will the news cause fear, existential agony, dancing in the streets or merely a worldwide shrug? And how much will that reaction depend on the news’s delivery?
During four days in February and March astrobiologists, journalists, science communicators, communications scholars, ethicists and artists got together digitally at a NASA Astrobiology Program workshop to discuss those questions. Over Zoom the participants discussed how researchers might find that elusive evidence of alien life in the universe and how to talk publicly about those hypothetical discoveries. “We all have our own disciplines,” says Jack Madden, an astrobiologist-turned-artist, who attended the workshop. “And this is a multidisciplinary endeavor. So we’re isolated in the knowledge we have and what other people are doing.” Part of the goal of the project was to cinch that knowledge gap.
The motley crew at the event, called “Communicating Discoveries in the Search for Life in the Universe,” spent their four sessions together hashing out the lessons astrobiology could take from the past and the ways they might be applied to the future—a future in which, perhaps, scientists will find evidence of extraterrestrial biology.
On supporting science journalism
If you're enjoying this article, consider supporting our award-winning journalism by subscribing . By purchasing a subscription you are helping to ensure the future of impactful stories about the discoveries and ideas shaping our world today.
But here’s the problem with the future: no one can foretell it. Will a discovery of alien life ever happen? Is there any alien life ? What forms might that life and its discovery take ? And what will the headline writers of 2028 (or 2058 or 2888) do with all that information?
No one knows answers to those questions, but because scientists love to predict, they and the other workshop participants made educated guesses and gamed them out with an eye toward relaying information about aliens to the rest of this world. As with the question of extraterrestrial life itself, though, concrete answers and plans were hard to come by.
In trying to predict the future, the past is always key—history is wont to repeat itself, at least on Earth. And so the workshop attendees discussed old tales of extraterrestrial news, such as the story of ALH84001 . Also known as the “ Allan Hills meteorite ,” this space rock traveled from Mars to land in the wilds of Antarctica, creating a dark, sharp spot among the ice fields. The meteorite had formed more than four billion years ago and then shot to space around 16 million years ago. It spent most of that time wandering the cosmic wilderness before ending up on Earth’s southernmost continent 13,000 years ago.
In 1996 a group of scientists—some of whom were affiliated with NASA— claimed in the journal Science that the special space rock, which the team had scrutinized closely by electron microscope, seemed to have microscopic fossils whose tubular, wormy character resembled that of earthly bacteria. “The image spoke immediately to a person,” Madden says—to laypeople, who know what bacteria look like, and to the scientists, who had been exposed to the same images of microorganisms for their whole life. “Having an image in that situation was so powerful,” he continues. The meteorite also had organic molecules and carbonate globules that contained magnetite, which further supported the idea that the rock might shake up the field of biology.
The findings, which were thought to potentially be the first evidence of life from another planet, shot through the headlines like the meteorite itself. Then-president Bill Clinton even gave a public speech about it after NASA had held its own press conference. “If this discovery is confirmed, it will surely be one of the most stunning insights into our universe that science has ever uncovered,” Clinton said. “Its implications are as far-reaching and awe-inspiring as can be imagined.”
In the view of most scientists, though, that confirmation hasn’t come through. Decades later the results remain the subject of debate, but the scientific consensus is that the rock’s chemistry and embedded shapes could be explained with mere geology and chemistry, not biology. During the initial announcements, officials showed caution and hedged their bets. “Like all discoveries, this one will and should continue to be reviewed, examined and scrutinized,” Clinton said.
But some scientists, including some who attended the workshop, see the hype around this preliminary and ultimately contested result as a failure nonetheless. They think that the finding’s announcers jumped the gun, prematurely waving “jazz hands” (a phrase workshop participants used to indicate official hype). This type of overpromotion can undermine trust and pass sticky but likely incorrect information into the public domain.
The kind of debate that ALH84001 started, though, is an important part of the scientific process. And scientific results are more likely to spark discussion that could lead, haltingly, toward the right answer if they are high-stakes—and maybe even a little hyped. Take, for instance, the 2020 announcement that scientists had found evidence of phosphine on Venus—and that, as far as they could tell, only life could produce that chemical on the searing, pressurized planet.
The result made splashy headlines—at the workshop, both journalists and scientists acknowledged that splashy headlines are here to stay—and it also caught the attention of scientists who scrutinized the claim and disagreed, in a debate that, like with ALH84001, is still going on. “This is how science evolves,” says Sarah Rugheimer, an astrobiologist and chair for the public understanding of astronomy at York University in Ontario and a workshop participant. “If I make a small, middling claim, no one’s going to care about it or read about it.”
Future discoveries are likely to include more like Venus’s alleged phosphine: today’s scientists, including many of those who attended the workshop, are keen to use super-powerful observatories such as the James Webb Space Telescope to search for “biosignatures” in the atmospheres of exoplanets—that is, chemicals they believe are produced by living beings.
Unlike with the Allan Hills meteorite, such data will provide nothing tangible to study, just photons and spectral fingerprints on computer screens. Proving that only living beings—and not, as with ALH84001, hypothetical quirks of geochemistry—have produced a purported biosignature is going to be difficult and maybe impossible. Scientists could send a probe to Venus to hunt for the hypothetical makers of phosphine, but any exoplanetary biosignature scientists find may be destined to be called “a sign that’s consistent with life” rather than “a sign of life” for a long time, if not forever. “I think that discovery of life is going to be an incremental process,” says Victoria Meadows, an astrobiologist at the University of Washington, who attended the workshop. “Unless something wanders past the camera and waves to us or encodes pi and transmits that in a radio signal, it’s not going to be definitive. And there’s going to be a lot of discussion going on.”
Scientists at the workshop and throughout history have worried that readers of news stories can’t hold that uncertainty or grasp the idea that follow-up research will be required. Some are not convinced that the public is scientifically literate enough to know or be shown that science is a process . But that’s perhaps an unfair assessment: often people see science as a set of settled results because that’s how it’s presented in public—in news stories and sometimes by scientists themselves. “At some level, we sort of hobble ourselves a bit in assuming that people can’t follow along, and I think that’s unfair,” Meadows says. In the workshop discussions, journalists called for agencies such as NASA to be more forthcoming, candid and timely—which would allow reporters to access the information and scientist sources they need to write about potential discoveries in a nuanced way. Many (though not all) scientists agreed, expressing frustration with the many levels of permission required to do public interviews and with statements carefully crafted by committee much too slowly for the news cycle.
Conveying where a result stands within ongoing debates while follow-up observations are happening is the key to good public information. But scientists are often reluctant to critique their peers’ work, and agencies such as NASA aren’t always keen to approve their researchers to do so. This makes it difficult, journalists at the workshop said, for the media or the public to evaluate a result’s status.
Something that could help with that is a set of “standards of evidence” for life claims—sort of like a key or legend to portray how many grains of salt to take with an alien life announcement. The standards would also show how those grains might dissolve as more evidence sluices in. “[The research process] is this arc of discovery, rather than a single point of discovery,” Meadows says. Scientists could come forward at any time during the research, including when results are far from certain, “as long as [they’re] clear about where [they] are in that process.”
Coming to the realization that the discovery of life, if it happens, will be an arc and not a point—and maybe a gray arc rather than a black-and-white one—was a process of its own for Rugheimer. “I started a little more bright-eyed and bushy-tailed way back when, and I thought we’d be able to” make a surefire discovery, she says. Now she’s taking a more practical approach. “The point when I pull out the champagne might just be what I consider a really strong signal but not something that might be 100 percent definite.”
To Adam Robinson, an astrobiologist at St. Petersburg College and NASA's Jet Propulsion Laboratory, Biodefense Coordinator at the Florida Bureau of Labs, and a workshop participant, the process of looking for signs of extraterrestrial life—and the possibility of spending years discovering and interpreting these signs—isn’t frustrating. It is, he says, inspiring.
“It’s the whole idea of people planting trees for shade that they’ll never sit under,” he says. “I went to a conference two years ago, and I was sitting there listening to these people planning these planetary science missions [in which] they’d probably be dead when the data came back. And it’s awesome to see people just commit their lives and their whole careers to something that they may never see.”
At the conclusion of the workshop, participants tried to sum up their conclusions in shared Google docs, to mixed success. If there was one takeaway, it was that attendees of all sorts thought news of potential alien discoveries shouldn’t shy away from the caveats: good public reporting should talk about uncertainty, include criticism, discuss the arduous process of confirmation and be honest about how far in the future a yes or no to the “aliens?” question is likely to be. That kind of communication demonstrates transparency and gives important context, even if a headline doesn’t.
Much of the workshop’s discussion, somewhat contradictorily, was nonetheless about how scientists and agencies such as NASA could coordinate messages that reach the public about those same discoveries. Crafting a message so tightly, though, doesn’t typically engender trust or read as transparent—something journalists pointed out in discussions. And besides, it rarely works: Plan a big, choreographed press conference for your best biosignature candidate for Tuesday at 8:14 A.M. EDT, and someone can leak the results Sunday at zero dark thirty. If you write an understated headline for your press release, be prepared for a tabloid (or digital publication hungry for traffic) to run with “NASA Finds Aliens!!!”
And then there are the inevitable mixed messages that occur when people aren’t familiar with the research process. Take the Mars Sample Return mission, which is set to launch later this decade and will bring material from the Red Planet back to Earth, in part to search for evidence of life. In one of the workshop’s scenarios, participants considered what might happen if the mission returned remnants of something alive, which would leave people concerned that Martian germs might bring a new plague to Earth.
The chance that Mars has Earth-harming microbes is extremely small—but not zero. And that’s why scientists plan to treat all material brought back as if it could be very dangerous. “They’re going to be housed in a facility that essentially is what we use to manipulate deadly pathogens such as Ebola,” Robinson says—basically a biosafety level 4 lab meant to protect people outside and in from danger. “So people hear that, and they’re going to be like, ‘I’ve got a scientist telling me there’s probably nothing to worry about. Then why are they putting it in there if there’s nothing to worry about?’”
Another communication complication, according to the Zoom meeting attendees, is that a significant proportion of the public thinks that we already have found aliens. And who can blame people? Headlines have suggested as much over and over for years for titillating false alarms or inconclusive results. If a statement includes the words “found aliens,” that’s, sensibly, the part that sticks, even if it also says “might have,” and “possibly”—especially if it meshes with a reader’s, watcher’s or listener’s existing worldview.
To nobly convey uncertainty, the nature of the scientific process and ongoing debate, then, means to first debunk incorrect information. The fact that that incorrect information is out there, though, also suggests that maybe the discovery of extraterrestrials wouldn’t actually be that Earth-shattering. After all, people who think aliens are among us have just gone on with their life.
With all those complications (and more), Rugheimer was left, at the end of the workshop, thinking that perhaps the discussion had focused too much on the wrong things—specifically, message control.
No matter how scripted a press conference is, people are going to think what they’re going to think—and people being people, they’re going to think a cornucopia of things. We have to “be real,” Rugheimer says, about how the next potential alien discovery might go down. “Someone is going to find something they think is cool. They’re going to try to publish it first. They’re going to be overinflated in what that discovery is,” she says.
“This is what happens,” she adds. “We’ve seen this time and time again. That’s how science works, too.”
In fact, the historical scenarios the workshop examined basically all occurred like this—in direct contradiction to the plans the participants were making for the future. To expect that the future will somehow unfold differently while humans as a species remain the same is magical thinking, Rugheimer says. “I think we as a community need to come to peace with the fact that there’s only so much we can do to try to be careful,” she continues. “Because as soon as there’s an exciting discovery, no one is going to be careful. And that’s okay.”
Sure, she continues, it’s fine to think about and have Zoom meetings about how to communicate more responsibly than before. “But we also need to have some framework of how to handle it when it’s done wrong,” she says. “Because it will be.”
Main Navigation
- Contact NeurIPS
- Code of Ethics
- Code of Conduct
- Create Profile
- Journal To Conference Track
- Diversity & Inclusion
- Proceedings
- Future Meetings
- Exhibitor Information
- Privacy Policy
NeurIPS 2024
Conference Dates: (In person) 9 December - 15 December, 2024
Homepage: https://neurips.cc/Conferences/2024/
Call For Papers
Author notification: Sep 25, 2024
Camera-ready, poster, and video submission: Oct 30, 2024 AOE
Submit at: https://openreview.net/group?id=NeurIPS.cc/2024/Conference
The site will start accepting submissions on Apr 22, 2024
Subscribe to these and other dates on the 2024 dates page .
The Thirty-Eighth Annual Conference on Neural Information Processing Systems (NeurIPS 2024) is an interdisciplinary conference that brings together researchers in machine learning, neuroscience, statistics, optimization, computer vision, natural language processing, life sciences, natural sciences, social sciences, and other adjacent fields. We invite submissions presenting new and original research on topics including but not limited to the following:
- Applications (e.g., vision, language, speech and audio, Creative AI)
- Deep learning (e.g., architectures, generative models, optimization for deep networks, foundation models, LLMs)
- Evaluation (e.g., methodology, meta studies, replicability and validity, human-in-the-loop)
- General machine learning (supervised, unsupervised, online, active, etc.)
- Infrastructure (e.g., libraries, improved implementation and scalability, distributed solutions)
- Machine learning for sciences (e.g. climate, health, life sciences, physics, social sciences)
- Neuroscience and cognitive science (e.g., neural coding, brain-computer interfaces)
- Optimization (e.g., convex and non-convex, stochastic, robust)
- Probabilistic methods (e.g., variational inference, causal inference, Gaussian processes)
- Reinforcement learning (e.g., decision and control, planning, hierarchical RL, robotics)
- Social and economic aspects of machine learning (e.g., fairness, interpretability, human-AI interaction, privacy, safety, strategic behavior)
- Theory (e.g., control theory, learning theory, algorithmic game theory)
Machine learning is a rapidly evolving field, and so we welcome interdisciplinary submissions that do not fit neatly into existing categories.
Authors are asked to confirm that their submissions accord with the NeurIPS code of conduct .
Formatting instructions: All submissions must be in PDF format, and in a single PDF file include, in this order:
- The submitted paper
- Technical appendices that support the paper with additional proofs, derivations, or results
- The NeurIPS paper checklist
Other supplementary materials such as data and code can be uploaded as a ZIP file
The main text of a submitted paper is limited to nine content pages , including all figures and tables. Additional pages containing references don’t count as content pages. If your submission is accepted, you will be allowed an additional content page for the camera-ready version.
The main text and references may be followed by technical appendices, for which there is no page limit.
The maximum file size for a full submission, which includes technical appendices, is 50MB.
Authors are encouraged to submit a separate ZIP file that contains further supplementary material like data or source code, when applicable.
You must format your submission using the NeurIPS 2024 LaTeX style file which includes a “preprint” option for non-anonymous preprints posted online. Submissions that violate the NeurIPS style (e.g., by decreasing margins or font sizes) or page limits may be rejected without further review. Papers may be rejected without consideration of their merits if they fail to meet the submission requiremhttps://www.overleaf.com/read/kcffhyrygkqc#85f742ents, as described in this document.
Paper checklist: In order to improve the rigor and transparency of research submitted to and published at NeurIPS, authors are required to complete a paper checklist . The paper checklist is intended to help authors reflect on a wide variety of issues relating to responsible machine learning research, including reproducibility, transparency, research ethics, and societal impact. The checklist forms part of the paper submission, but does not count towards the page limit.
Supplementary material: While all technical appendices should be included as part of the main paper submission PDF, authors may submit up to 100MB of supplementary material, such as data, or source code in a ZIP format. Supplementary material should be material created by the authors that directly supports the submission content. Like submissions, supplementary material must be anonymized. Looking at supplementary material is at the discretion of the reviewers.
We encourage authors to upload their code and data as part of their supplementary material in order to help reviewers assess the quality of the work. Check the policy as well as code submission guidelines and templates for further details.
Use of Large Language Models (LLMs): We welcome authors to use any tool that is suitable for preparing high-quality papers and research. However, we ask authors to keep in mind two important criteria. First, we expect papers to fully describe their methodology, and any tool that is important to that methodology, including the use of LLMs, should be described also. For example, authors should mention tools (including LLMs) that were used for data processing or filtering, visualization, facilitating or running experiments, and proving theorems. It may also be advisable to describe the use of LLMs in implementing the method (if this corresponds to an important, original, or non-standard component of the approach). Second, authors are responsible for the entire content of the paper, including all text and figures, so while authors are welcome to use any tool they wish for writing the paper, they must ensure that all text is correct and original.
Double-blind reviewing: All submissions must be anonymized and may not contain any identifying information that may violate the double-blind reviewing policy. This policy applies to any supplementary or linked material as well, including code. If you are including links to any external material, it is your responsibility to guarantee anonymous browsing. Please do not include acknowledgements at submission time. If you need to cite one of your own papers, you should do so with adequate anonymization to preserve double-blind reviewing. For instance, write “In the previous work of Smith et al. [1]…” rather than “In our previous work [1]...”). If you need to cite one of your own papers that is in submission to NeurIPS and not available as a non-anonymous preprint, then include a copy of the cited anonymized submission in the supplementary material and write “Anonymous et al. [1] concurrently show...”). Any papers found to be violating this policy will be rejected.
OpenReview: We are using OpenReview to manage submissions. The reviews and author responses will not be public initially (but may be made public later, see below). As in previous years, submissions under review will be visible only to their assigned program committee. We will not be soliciting comments from the general public during the reviewing process. Anyone who plans to submit a paper as an author or a co-author will need to create (or update) their OpenReview profile by the full paper submission deadline. Your OpenReview profile can be edited by logging in and clicking on your name in https://openreview.net/ . This takes you to a URL "https://openreview.net/profile?id=~[Firstname]_[Lastname][n]" where the last part is your profile name, e.g., ~Wei_Zhang1. The OpenReview profiles must be up to date, with all publications by the authors, and their current affiliations. The easiest way to import publications is through DBLP but it is not required, see FAQ . Submissions without updated OpenReview profiles will be desk rejected. The information entered in the profile is critical for ensuring that conflicts of interest and reviewer matching are handled properly. Because of the rapid growth of NeurIPS, we request that all authors help with reviewing papers, if asked to do so. We need everyone’s help in maintaining the high scientific quality of NeurIPS.
Please be aware that OpenReview has a moderation policy for newly created profiles: New profiles created without an institutional email will go through a moderation process that can take up to two weeks. New profiles created with an institutional email will be activated automatically.
Venue home page: https://openreview.net/group?id=NeurIPS.cc/2024/Conference
If you have any questions, please refer to the FAQ: https://openreview.net/faq
Ethics review: Reviewers and ACs may flag submissions for ethics review . Flagged submissions will be sent to an ethics review committee for comments. Comments from ethics reviewers will be considered by the primary reviewers and AC as part of their deliberation. They will also be visible to authors, who will have an opportunity to respond. Ethics reviewers do not have the authority to reject papers, but in extreme cases papers may be rejected by the program chairs on ethical grounds, regardless of scientific quality or contribution.
Preprints: The existence of non-anonymous preprints (on arXiv or other online repositories, personal websites, social media) will not result in rejection. If you choose to use the NeurIPS style for the preprint version, you must use the “preprint” option rather than the “final” option. Reviewers will be instructed not to actively look for such preprints, but encountering them will not constitute a conflict of interest. Authors may submit anonymized work to NeurIPS that is already available as a preprint (e.g., on arXiv) without citing it. Note that public versions of the submission should not say "Under review at NeurIPS" or similar.
Dual submissions: Submissions that are substantially similar to papers that the authors have previously published or submitted in parallel to other peer-reviewed venues with proceedings or journals may not be submitted to NeurIPS. Papers previously presented at workshops are permitted, so long as they did not appear in a conference proceedings (e.g., CVPRW proceedings), a journal or a book. NeurIPS coordinates with other conferences to identify dual submissions. The NeurIPS policy on dual submissions applies for the entire duration of the reviewing process. Slicing contributions too thinly is discouraged. The reviewing process will treat any other submission by an overlapping set of authors as prior work. If publishing one would render the other too incremental, both may be rejected.
Anti-collusion: NeurIPS does not tolerate any collusion whereby authors secretly cooperate with reviewers, ACs or SACs to obtain favorable reviews.
Author responses: Authors will have one week to view and respond to initial reviews. Author responses may not contain any identifying information that may violate the double-blind reviewing policy. Authors may not submit revisions of their paper or supplemental material, but may post their responses as a discussion in OpenReview. This is to reduce the burden on authors to have to revise their paper in a rush during the short rebuttal period.
After the initial response period, authors will be able to respond to any further reviewer/AC questions and comments by posting on the submission’s forum page. The program chairs reserve the right to solicit additional reviews after the initial author response period. These reviews will become visible to the authors as they are added to OpenReview, and authors will have a chance to respond to them.
After the notification deadline, accepted and opted-in rejected papers will be made public and open for non-anonymous public commenting. Their anonymous reviews, meta-reviews, author responses and reviewer responses will also be made public. Authors of rejected papers will have two weeks after the notification deadline to opt in to make their deanonymized rejected papers public in OpenReview. These papers are not counted as NeurIPS publications and will be shown as rejected in OpenReview.
Publication of accepted submissions: Reviews, meta-reviews, and any discussion with the authors will be made public for accepted papers (but reviewer, area chair, and senior area chair identities will remain anonymous). Camera-ready papers will be due in advance of the conference. All camera-ready papers must include a funding disclosure . We strongly encourage accompanying code and data to be submitted with accepted papers when appropriate, as per the code submission policy . Authors will be allowed to make minor changes for a short period of time after the conference.
Contemporaneous Work: For the purpose of the reviewing process, papers that appeared online within two months of a submission will generally be considered "contemporaneous" in the sense that the submission will not be rejected on the basis of the comparison to contemporaneous work. Authors are still expected to cite and discuss contemporaneous work and perform empirical comparisons to the degree feasible. Any paper that influenced the submission is considered prior work and must be cited and discussed as such. Submissions that are very similar to contemporaneous work will undergo additional scrutiny to prevent cases of plagiarism and missing credit to prior work.
Plagiarism is prohibited by the NeurIPS Code of Conduct .
Other Tracks: Similarly to earlier years, we will host multiple tracks, such as datasets, competitions, tutorials as well as workshops, in addition to the main track for which this call for papers is intended. See the conference homepage for updates and calls for participation in these tracks.
Experiments: As in past years, the program chairs will be measuring the quality and effectiveness of the review process via randomized controlled experiments. All experiments are independently reviewed and approved by an Institutional Review Board (IRB).
Financial Aid: Each paper may designate up to one (1) NeurIPS.cc account email address of a corresponding student author who confirms that they would need the support to attend the conference, and agrees to volunteer if they get selected. To be considered for Financial the student will also need to fill out the Financial Aid application when it becomes available.

latest in US News

ADL accuses Ilhan Omar of 'blood libel' for labeling Jewish...

Man claims he was 'over medicated' when he buried wife alive —...

NJ's ban on immigrant detention centers challenged in fiery...

Green Party presidential candidate arrested during anti-Israel...

'Long-withheld rage' from COVID shutdowns, isolation to blame for...

College protesters seek amnesty to keep arrests and suspensions...

New York trans advocate, park ranger falls to her death while ice...

Doctor who drove Tesla off cliff with family inside had...
Yale professor accuses columbia prez shafik of plagiarism, ‘intellectual theft’ in resurfaced 1994 research paper.
- View Author Archive
- Get author RSS feed
- Email the Author
- Follow on Twitter
Contact The Author
Thanks for contacting us. We've received your submission.
Thanks for contacting us. We've received your submission.
Embattled Columbia University president Nemat “Minouche” Shafik screwed a former underling out of credit on a research paper published 30 years ago, a Yale University professor claims.
Ahmed Mushfiq Mobarak posted the bombshell allegations in a blistering thread on X early Friday, juxtaposing images of a 1992 report Shafik co-authored for World Bank with researcher Sushenjit Bandyopadhyay, along with a journal published in Oxford Economic Papers two years later in which Bandyopadhyay’s name was removed.

Mobarak, an economics and management professor at Yale, told The Post the findings and research cited in both papers are pretty much equal.
“It got rewritten, but fundamentally it’s the same paper,” he alleged.

“We can’t get in the room and [learn] what sentences did he write and what sentences she wrote, but what we do know is his contribution was sufficient to warrant co-authorship [in 1992],” he added. “What is not common is for someone to be a co-author and then suddenly their name is taken off.”
Instead, Bandyopadhyay is only “thanked” in an acknowledgement section in the back of the 1994 published journal — which screams of “power asymmetry” considering Shafik was then Bandyopadhyay’s boss, alleged Mobarak.
Bandyopadhyay declined comment when asked whether he felt slighted.
However, Mobarak, also a former World Bank consultant and University of Maryland graduate, said he spoke to Bandyopadhyay about the issue and that Bandyopadhyay believes he should have been credited as a co-author in the second paper. The professor conceded Bandyopadhyay never said anything “negative” about the Columbia president.

“This [1994] paper is lifted almost entirely from a 1992 report coauthored with consultant not credited in the publication,” wrote Mobarak on X. “This is wholesale intellectual theft, not subtle plagiarism.”
At the time both papers were written, Shafik was a vice president for World Bank and Bandyopadhyay, a consultant who also attended the University of Maryland.

Mobarak’s allegations echo plagiarism accusations leveled against former Harvard University president Claudine Gay, who eventually resigned in disgrace in January .
Columbia University spokesperson Ben Chang shot down the Yale professor’s claims, saying “this is an absurd attempt at running a well-known playbook, and it has no credibility.”
Share this article:

Advertisement
- Publications
Transforming Energy Demand
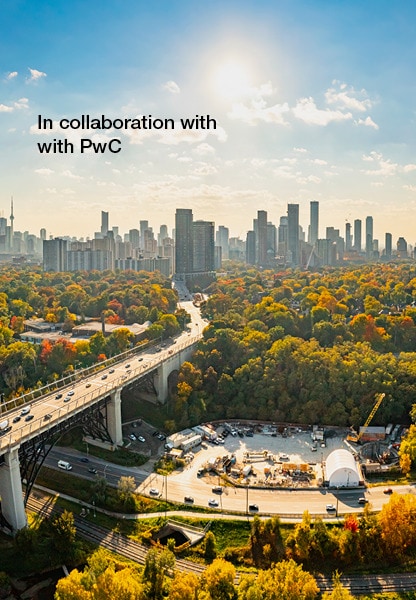
In this paper, the Transforming Energy Demand initiative outlines actions for businesses and countries to enhance energy management, efficiency and carbon intensity reduction. It highlights commercially beneficial levers, implementable with existing technologies, to impact the transition significantly.
Adopting measures for energy-efficient output and service delivery is essential for businesses and countries to sustain economic growth and achieve net-zero goals.
As the global population and energy demand rise, particularly in developing markets, implementing public policies and fostering value chain collaborations are key to managing energy consumption and reducing carbon intensity. This will help mitigate energy costs and supply issues and unlock commercial benefits, thereby accelerating the transition. At COP28, over 120 countries committed to doubling the pace of energy efficiency improvement, necessitating concrete, realistic plans.
World Economic Forum reports may be republished in accordance with the Creative Commons Attribution-NonCommercial-NoDerivatives 4.0 International Public License , and in accordance with our Terms of Use .
Further reading All related content
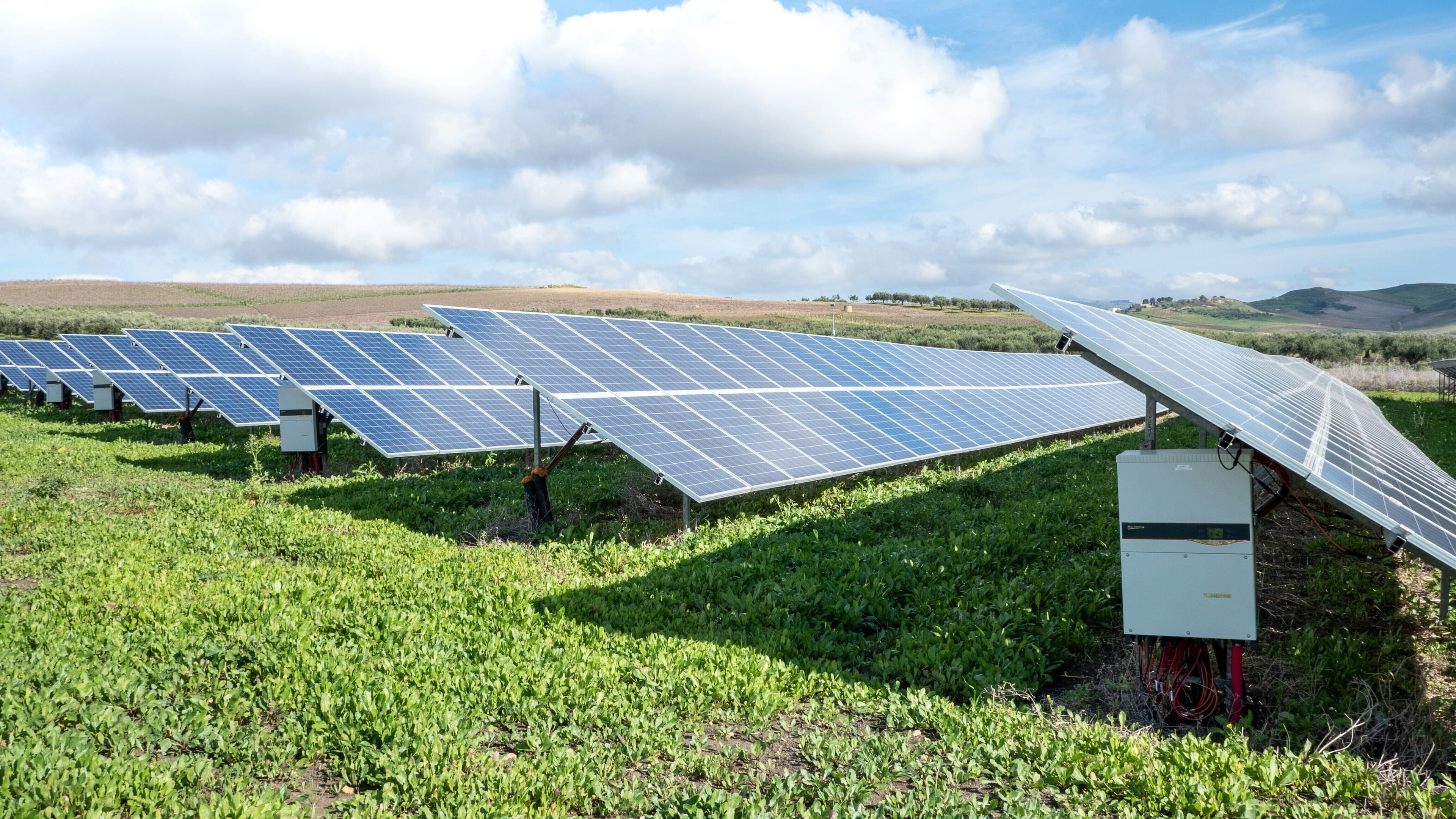
Cómo Davos 2024 fijó la agenda para acelerar una transición energética justa y rentable
La transición a la energía verde debe ser equitativa y justa, y el capital debe dirigirse a los más necesitados. Si se hace bien, podemos ahorrar billones y triplicar la energía limpia.
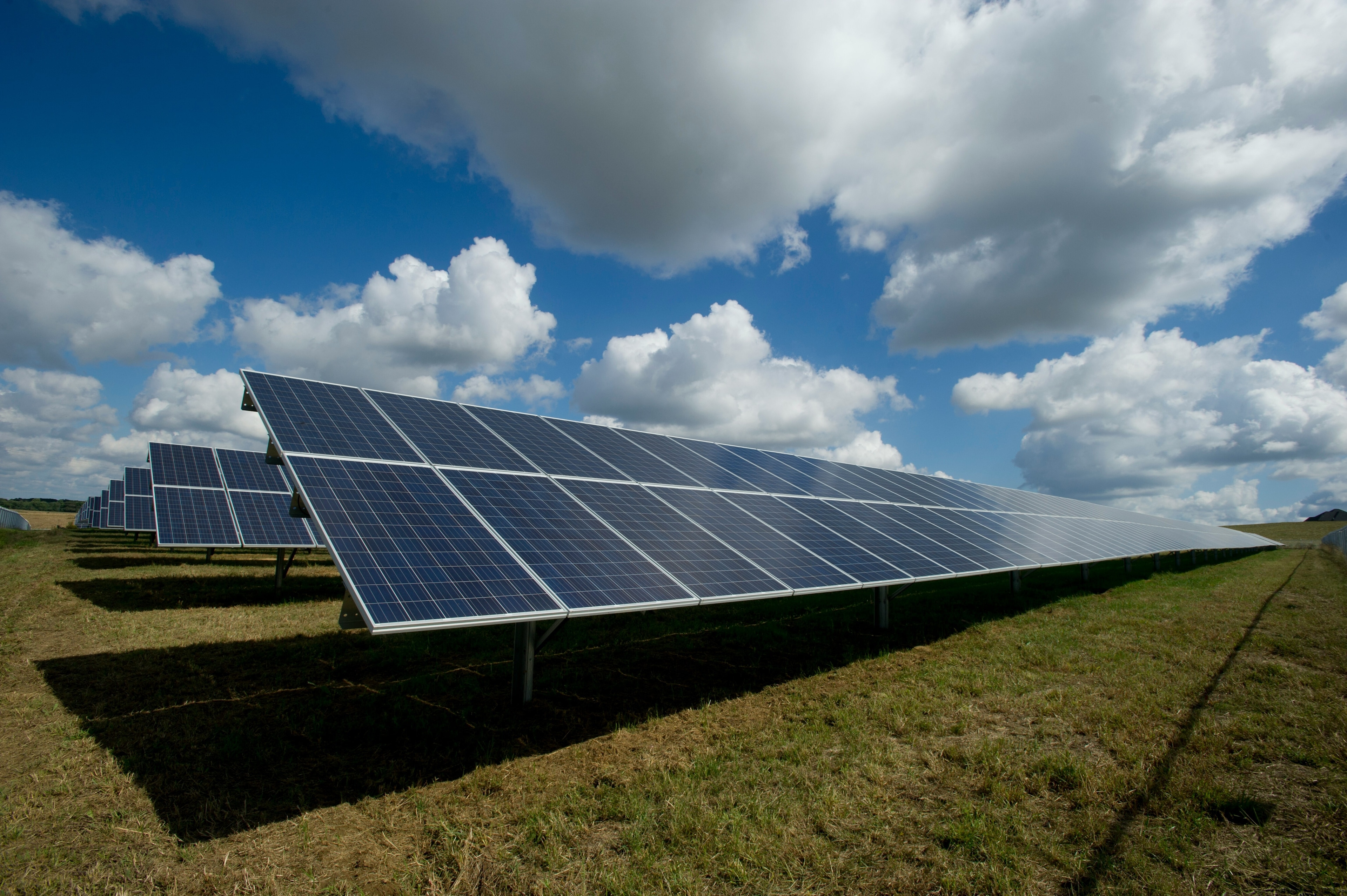
The world added 50% more renewable capacity last year than in 2022
How much is global renewable energy capacity increasing and what must happen to achieve the COP28 pledge to triple clean energy capacity by 2030?
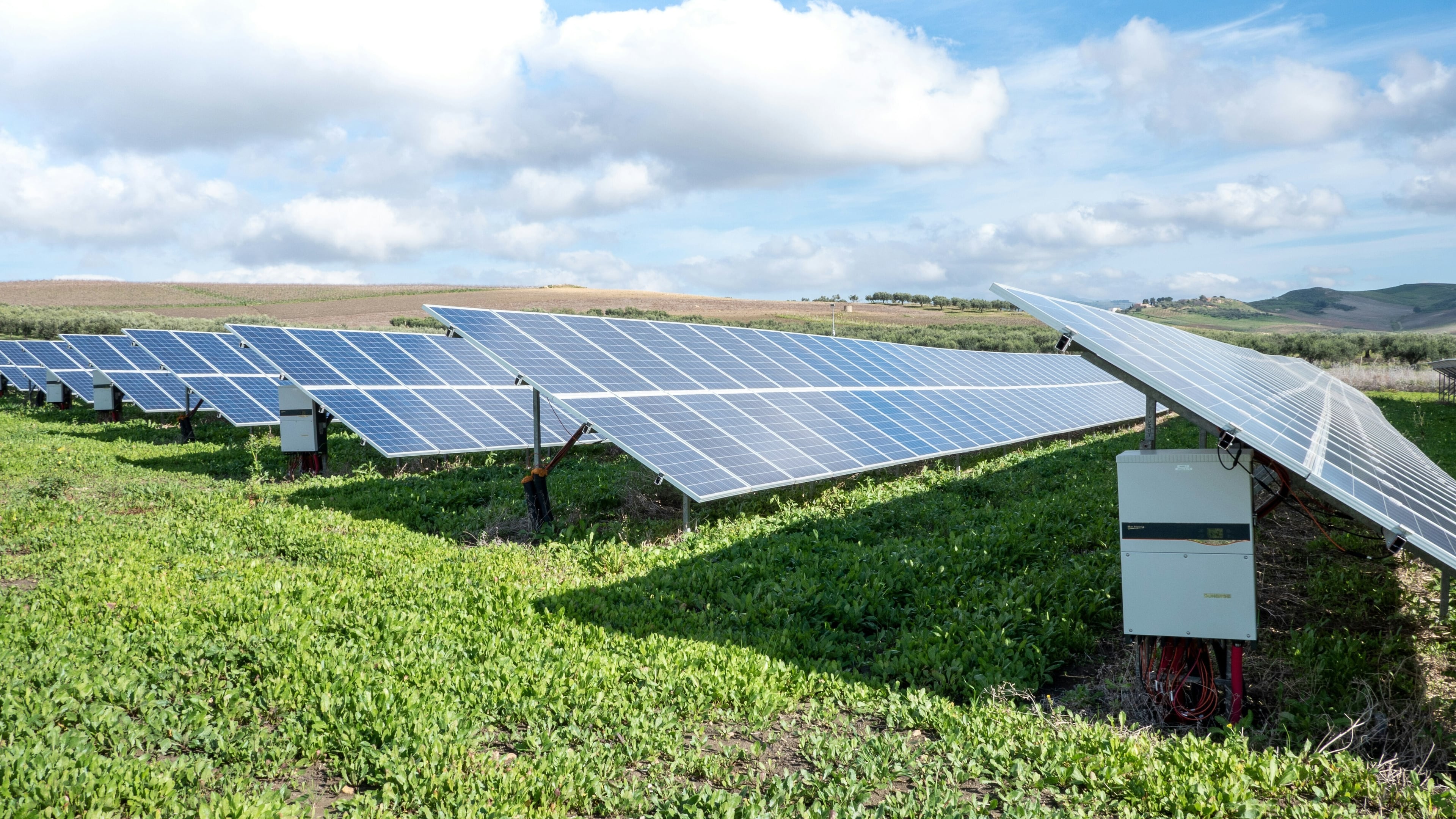
How Davos 2024 set the agenda for accelerating the energy transition in a fair and cost-effective way
The green energy transition must be equitable and fair, with capital directed to the most needed. If done right, we can save trillions and triple clean energy.

IMAGES
VIDEO
COMMENTS
NASA. The researchers carefully simulated the atmospheric chemistry of alien worlds devoid of life thousands of times over a period of more than four years, varying the atmospheric compositions and star types. "When we ran these calculations, we found that in some cases, there was a significant amount of ozone that built up in the atmosphere ...
By The Hebrew University of Jerusalem February 1, 2024. New research redefines the Habitable Zone of exoplanets by considering subglacial liquid water, extending potentially habitable conditions beyond traditional boundaries. Supported by recent JWST observations, this study broadens the scope for finding life on exoplanets, especially those ...
SETI, the search for extraterrestrial intelligence, is deploying machine-learning algorithms that filter out Earthly interference and spot signals humans might miss. Machine learning is good at ...
For all these reasons, we need to invoke multiple methods to search for signs of life in the atmosphere of other worlds. In a recent paper, we proposed information theory—a methodology to decode ...
The search for weird life isn't actually a search for life, Bartlett argues. It's a search for "lyfe," a term proposed in a 2020 article he co-authored in, ironically, the journal Life ...
Related links in Nature Research. These seven alien worlds could help explain how planets form 2017-Feb-22. Earth-sized planet around nearby star is astronomy dream come true 2016-Aug-24.
But now the study of Boyajian's star is being promoted as a research model that could help in one of the most intriguing of all scientific quests: finding intelligent life on other worlds.
1 SETI in the context of this article means SETI research (as it is performed around the world) and should not be confused with the SETI Institute. In the cases where mention is made of the SETI Institute, this is clearly spelled out. ... Gulkis S., Olsen E.T., Oliver B.M., Tarter J.C., and Seeger C.L. (1980) A search strategy for SETI [paper # ...
Research across a range of fields, from examining caves on Earth to visiting distant worlds, is on the cusp of bringing us closer to the discovery (or continued refutation) of alien life than ever ...
Its source was closer to Australia. That's why Green and NASA are proposing a scale to better contextualize new findings in research about extraterrestrial life and help explain those discoveries ...
A fringe theory called "panspermia" suggests that lifeforms can spread to new planets by hitching rides on meteors. New research lays out a roadmap for finding where these hypothetical, planet ...
Abstract. In this article, we partially quantify the biological potential of an exoplanet. We employ a variety of biogeographical analyses, placing biological evolution in the context of the geological evolution of the planet as a whole. Terrestrial (as in Earthly) biodiversity is tightly constrained in terms of species richness by its environment.
A Feature Paper should be a substantial original Article that involves several techniques or approaches, provides an outlook for future research directions and describes possible research applications. Feature papers are submitted upon individual invitation or recommendation by the scientific editors and must receive positive feedback from the ...
One fascinating analog for exotic organisms on an alien world are slime molds. Here on Earth, under certain conditions, they aggregate into acellular, multinucleate bags of protoplasm, with an ...
A post headlined "What a Wonderful World" took a swipe at "a so-called 'Harvard astrophysicist" who in 2022 told The Harvard Crimson that he didn't expect Loeb's efforts to be ...
The Alien Worlds Technical Blueprint (also known as the Whitepaper) document is the foundation of Alien Worlds knowledge. It includes descriptions and calculations of the game, as well as the original game lore and a link to the official attributes sheet. Download PDF: Blockchain Technical Blueprint.
Pop culture icons such as Flash Gordon, Buck Rogers, and alien creatures have populated our movie and television screens since the 1930's. In the 1990s, astronomers detected the first worlds orbiting distant stars, and as of January 2010, at least 424 of these exoplanets are known to exist. As our observation techniques improve, we look to ...
On June 9, with only a few hours' notice, NASA held a press conference to announce a study it was commissioning on unidentified aerial phenomena (UAPs). The acronym is a rebranding of what are ...
In the 1940s and 50s reports of "flying saucers" became an American cultural phenomena. Sightings of strange objects in the sky became the raw materials for Hollywood to present visions of potential threats. Posters for films, like Earth vs. the Flying Saucers from 1956 illustrate these fears. Connected to ongoing ideas about life on the Moon, the canals on Mars, and ideas about Martian ...
There are many notable UFO encounters around the world involving single witnesses, including the 1964 Lonnie Z amora incident, the 1965 V alensole UFO Encounter, the 196 7 Falcon Lake incident ...
Guide - Technical Blueprint. The Alien Worlds Technical Blueprint (also known as the Whitepaper) document is the foundation of Alien Worlds knowledge. It includes descriptions and calculations of the game, as well as the original game lore and a link to the official attributes sheet. Find the Technical Blueprint PDF file here: https ...
Tim Gallaudet, an oceanographer and former Naval rear admiral who served as the author of a March white paper about so-called "unidentified submerged objects" or USOs, told Fox News this week that ...
As pioneers in the vast and evolving universe of Alien Worlds, Dacoco has continually sought ways to innovate, empower, and engage our vibrant community. 03.28.24. Gameplay. Tokenized Lore Blog Article Series 5: Onoros. Part five in a series of articles that will break down all aspects of the new races, vehicles, and architecture!
The Alien Worlds community recently approved the new foundational lore for the Alien Worlds metaverse, setting the stage for unique and engaging narratives. "Starblind," a webcomic set in a mining prison colony on Magor and supported in part by the Galactic Hubs grant program, is a prime example of this new lore coming to life.
Scientists could send a probe to Venus to hunt for the hypothetical makers of phosphine, but any exoplanetary biosignature scientists find may be destined to be called "a sign that's ...
Call For Papers. Abstract submission deadline: May 15, 2024 01:00 PM PDT or. Full paper submission deadline, including technical appendices and supplemental material (all authors must have an OpenReview profile when submitting): May 22, 2024 01:00 PM PDT or. Author notification: Sep 25, 2024.
Yale management and economics professor Ahmed Mushfiq Mobarak accused Columbia University president Minouche Shafik of "intellectual theft" over a 30-year-old research paper he says fails to ...
This white paper contains the findings of phase one of the Transforming Energy Demand initiative (2023/24). It demonstrates a compelling case for energy demand actions. Research and International Business Council (IBC) members' examples show the potential for around a 31% reduction in the amount of energy required for businesses to deliver products and services at attractive returns, requiring ...