Newest Articles
- Perception and Knowledge: An Overview
- Exploring Moral Relativism: A Comprehensive Overview
- The Nicomachean Ethics by Aristotle
- Exploring Friedrich Nietzsche: An Introduction to the Major Modern Philosopher
- Metaphysics
- Theory of Forms
- Epistemology
- Materialism
- Moral relativism
- Utilitarianism
- Virtue ethics
- Normative ethics
- Applied ethics
- Moral Psychology
- Philosophy of art
- Philosophy of language
- Philosophy of beauty
- Nature of Art
- Philosophy of Film
- Philosophy of Music
- Deductive reasoning
- Inductive reasoning
- Justification
- Perception and Knowledge
- Beliefs and Truth
- Modern philosophy
- Romanticism
- Analytic philosophy
- Enlightenment philosophy
- Existentialism
- Enlightenment
- Ancient philosophy
- Classical Greek philosophy
- Renaissance philosophy
- Medieval philosophy
- Pre-Socratic philosophy
- Hellenistic philosophy
- Presocratic philosophy
- Rationalism
- Scholasticism
- Jewish philosophy
- Early Islamic philosophy
- Reasoning and Argumentation
- Seeking Justice After a Tractor-Trailer Accident: Why You Need an Experienced Lawyer
- Critical Thinking
- Fallacies and logical errors
- Skepticism and doubt
- Creative Thinking
- Lateral thinking
- Thought experiments
- Argumentation and Logic
- Syllogisms and Deductive Reasoning
- Fallacies and Rebuttals
- Inductive Reasoning and Analogy
- Reasoning and Problem-Solving
- Critical Thinking and Decision Making
- Creative Thinking and Problem Solving
- Analytical Thinking and Reasoning
- Philosophical Writing and Analysis
- Argumentative Writing and Analysis
- Interpreting Philosophical Texts
- Writing Essays and Articles on Philosophy
- Philosophical Research Methods
- Qualitative Research Methods in Philosophy
- Quantitative Research Methods in Philosophy
- Research Design and Methodology
- Ethics and Morality
- Aesthetics and Beauty
- Metaphysical terms
- Ontological argument
- Ethical terms
- Aesthetic terms
- Metaphysical theories
- Kant's Categorical Imperative
- Aristotle's Four Causes
- Plato's Theory of Forms
- Hegel's Dialectic
- Ethical theories
- Aesthetic theories
- John Dewey's aesthetic theory
- Immanuel Kant's aesthetic theory
- Modern philosophical texts
- Foucault's The Order of Things
- Descartes' Meditations
- Nietzsche's Beyond Good and Evil
- Wittgenstein's Tractatus Logico-Philosophicus
- Ancient philosophical texts
- Kant's Critique of Pure Reason
- Hegel's Phenomenology of Spirit
- Aristotle's Nicomachean Ethics
- Plato's Republic
- Ancient philosophers
- Modern philosophers
- Modern philosophical schools
- German Idealism
- British Empiricism
- Ancient philosophical schools
- The Skeptic school
- The Cynic school
- The Stoic school
- The Epicurean school
- The Socratic school
- Philosophy of Language
- Semantics and Pragmatics of Language Usage
- Analytic-Synthetic Distinction
- Meaning of Words and Phrases
- Philosophy of Science
- Scientific Realism and Rationalism
- Induction and the Hypothetico-Deductive Model
- Theory-Ladenness and Underdetermination
- Philosophy of Mind
- Mind-Body Dualism and Emergentism
- Materialism and Physicalism
- Identity Theory and Personal Identity
- Philosophy of Religion
- Religious Pluralism and Exclusivism
- The Problem of Evil and Suffering
- Religious Experience and Faith
- Metaphysical Theories
- Idealism and Realism
- Determinism, Fatalism, and Libertarianism
- Phenomenalism and Nominalism
- Epistemological Theories
- Intuitionism, Skepticism, and Agnosticism
- Rationalism and Empiricism
- Foundationalism and Coherentism
- Aesthetic Theories
- Formalist Aesthetics, Emotional Aesthetics, Experiential Aesthetics
- Relational Aesthetics, Sociological Aesthetics, Historical Aesthetics
- Naturalistic Aesthetics, Immanent Aesthetics, Transcendental Aesthetics
- Ethical Theories
- Virtue Ethics, Utilitarianism, Deontology
- Subjectivism, Egoism, Hedonism
- Social Contract Theory, Natural Law Theory, Care Ethics
- Metaphysical Terms
- Cause, Necessity, Possibility, Impossibility
- Identity, Persistence, Time, Space
- Substance, Attribute, Essence, Accident
- Logic and Argumentation Terms
- Analogy, Syllogism, Deduction, Induction
- Inference, Validity, Soundness, Refutation
- Premise, Conclusion, Entailment, Contradiction
- Epistemological Terms
- Perception and Knowledge Claims
- Infallibility, Verifiability, Coherence Theory of Truth
- Justification, Beliefs and Truths
- Ethical Terms
- Modern Texts
- A Vindication of the Rights of Woman by Mary Wollstonecraft
- Thus Spoke Zarathustra by Friedrich Nietzsche
- The Critique of Pure Reason by Immanuel Kant
- Medieval Texts
- The Guide for the Perplexed by Moses Maimonides
- The Summa Theologiae by Thomas Aquinas
- The Incoherence of the Incoherence by Averroes
- Ancient Texts
- The Art of Rhetoric by Cicero
- The Republic by Plato
- Exploring Skepticism and Doubt: A Philosophical and Critical Thinking Perspective
- Philosophical thinking
From Socrates to the present day, skepticism and doubt have been at the forefront of philosophical thought. Skepticism has been used to challenge existing beliefs and assumptions, while doubt has been used to question and probe ideas, concepts, and beliefs. In this article, we explore the philosophical and critical thinking perspectives on skepticism and doubt. We will look at how they have been used throughout history to shape our thinking and inform our decisions.
We will also examine the implications of skepticism and doubt for our society today and how they can be used to foster greater understanding and collaboration. Skepticism and doubt have long been seen as tools of inquiry and analysis. They have been used to challenge established conventions, challenge accepted wisdom, and explore new ideas. As such, skepticism and doubt can be seen as essential elements of philosophical thinking. This article will explore the various ways in which skepticism and doubt have been employed by philosophers throughout history, as well as their implications for critical thinking. We will also examine the implications of skepticism and doubt in today's world.
We will look at how they can be used to promote greater understanding, collaboration, and progress in our society. We will also explore the potential pitfalls of relying too heavily on skepticism and doubt, such as the tendency to become too skeptical or too trusting of ideas. Finally, we will discuss how skepticism and doubt can be used in a constructive manner to promote meaningful dialogue and progress. Skepticism and doubt are two closely related concepts that have been discussed in philosophy and critical thinking for centuries. They involve questioning beliefs or assumptions, and seeking evidence in order to form one’s own conclusions.

Historically, skepticism and doubt have been used to challenge existing beliefs and accepted truths
Today, skepticism and doubt can still be used to think more critically about our beliefs and assumptions, by questioning our beliefs and assumptions, we can avoid making decisions based on false information or our own biases, when we question our beliefs and assumptions, it can help us to think more deeply about our positions and arguments, if taken too far, they can lead to a sense of cynicism or apathy, which can prevent us from forming meaningful connections with others or taking meaningful action.
By questioning our beliefs and assumptions, we can avoid making decisions based on false information or biases. We can also identify potential pitfalls in our reasoning and uncover alternative perspectives that we may not have considered before. Finally, when engaging in debates or discussions, skepticism and doubt can help us to think more deeply about our positions and arguments. However, it is important to use skepticism and doubt judiciously in order to avoid falling into a state of cynicism or apathy. For those looking to learn more about skepticism and doubt, there are a number of resources available.
What is Skepticism and Doubt?
Philosophical skepticism can be divided into several different types, including Cartesian skepticism, Pyrrhonism, Academic skepticism, and Humean skepticism. Cartesian skepticism is the view that nothing can be known with absolute certainty, while Pyrrhonism holds that nothing can be known with absolute certainty and that one should suspend judgement until further evidence is obtained. Academic skepticism maintains that no knowledge is certain and that wisdom is achieved through doubt, while Humean skepticism suggests that knowledge is based on experience and can never be certain. Scientific skepticism is a form of inquiry that uses scientific methods to investigate claims made in the absence of definitive proof. This type of skepticism is based on the premise that extraordinary claims require extraordinary evidence in order to be accepted as valid.
The Historical Roots of Skepticism and Doubt
Descartes argued that people should use their own reason and experience to form beliefs, rather than relying solely on the teachings of others. He was an advocate of the “method of doubt”, which instructed people to doubt all of their beliefs until they could be proven true. Descartes’ ideas were highly influential and were taken up by other philosophers such as David Hume. Hume argued that humans are limited in their knowledge, and that we should not assume our beliefs to be true unless there is sufficient evidence for them.
The Application of Skepticism and Doubt
Additionally, by applying doubt to our problem-solving abilities, we can avoid the potential pitfalls of relying too heavily on instinct or intuition. For example, if we are presented with a complicated problem, we may be tempted to rush to a solution without considering the wider implications of our decision. However, by taking a moment to consider the implications of our decision and question any underlying assumptions, we can make sure that our solution is as informed and well-considered as possible. In addition to helping us think more critically, skepticism and doubt can help us develop a healthier sense of self-awareness. By questioning our own beliefs and assumptions, we can gain a better understanding of our own thought processes and the biases which might be influencing them.
Resources for Learning More About Skepticism and Doubt
Carroll 2.The Internet Encyclopedia of Philosophy: A Peer-Reviewed Academic Resource 3.The Skeptics Society: Promoting Science and Reason Since 1992 4.Doubtful News: Keeping You Informed on Strange and Unusual Claims Podcasts: 1.Skeptoid: Critical Analysis of Pop Phenomena 2.The Partially Examined Life: Philosophy for the Rest of Us 3.Reasonable Doubts: Exploring the Claims of Christianity 4.Think Twice: Exploring the Relationship between Science and ReligionIn conclusion, skepticism and doubt are important philosophical and critical thinking tools that can help us better understand our world, beliefs, assumptions, and perspectives. By engaging in questioning and challenging our beliefs and assumptions, we are able to think more critically and gain new insights. Furthermore, skepticism and doubt can be used to inform our decision-making processes, problem-solving abilities, and more. Finally, there are a variety of resources available for readers to learn more about skepticism and doubt and how to apply them to their everyday lives.
Skepticism , doubt , critical thinking , philosophical thinking , and decision-making are all key concepts explored in this article.
Top Articles
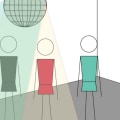
- Understanding Fallacies and Logical Errors
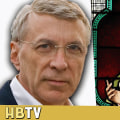
- Exploring the Ontological Argument
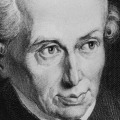
- The Critique of Pure Reason by Immanuel Kant: A Comprehensive Overview
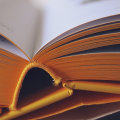
- Exploring Pragmatism: A Modern Philosophical School
- Exploring the Theory of Forms: A Comprehensive Overview
- Exploring the Philosophy of Art
- Classical Greek Philosophy: A Comprehensive Overview
- Understanding Utilitarianism: A Guide
- Exploring Pragmatism: A Modern Philosophy
- Analytic Philosophy: A Comprehensive Overview
- Medieval Philosophy: An Overview
- Epistemology: Understanding the Nature of Knowledge
- Exploring the Ideas of Enlightenment Philosophy
- Existentialism: An Introduction
- Exploring Idealism: The History and Concepts of a Modern Philosophy
- Exploring Pre-Socratic Philosophy: An Overview
- Exploring Ontology: A Comprehensive Overview
- Understanding Inference: A Comprehensive Overview
- Philosophy of Language: Exploring the Ways We Communicate
- Exploring the Philosophy of Beauty
- Understanding Inductive Reasoning
- Understanding Deontology: Ethics and Principles
- Lateral Thinking: An Overview
- Thought Experiments: Exploring Creative and Philosophical Thinking
- Exploring Hellenistic Philosophy: An Introduction
- Exploring Deductive Reasoning
- A Comprehensive Look at Causality
- Exploring Egoism: What It Is and What It Means
- Exploring the Ethical Theory of Utilitarianism
- Exploring Aristotle's Four Causes
- Exploring Plato's Theory of Forms
- Deontology: An Introduction to an Ethical Theory
- Exploring Virtue Ethics: The Philosophical Theory
- Philosophy of Art: Exploring Aesthetics and Beauty
- Hegel's Dialectic: A Comprehensive Overview
- Descartes' Meditations: An Introduction for None
- Aristotle: A Comprehensive Overview
- Exploring Hegel's Phenomenology of Spirit
- Exploring Expression: A Philosophical and Aesthetic Overview
- Exploring Noumenon: A Philosophical and Metaphysical Overview
- Exploring the Life and Legacy of Cicero: An Introduction
- Exploring Taste: A Philosophical and Aesthetic Guide
- Exploring Nietzsche's Beyond Good and Evil
Exploring Wittgenstein's Tractatus Logico-Philosophicus
- Descartes: A Comprehensive Overview
- Sublime: An Introduction to Aesthetic and Philosophical Terms
- Exploring Plato's Republic
- Understanding Existentialism: A Brief Introduction
- Exploring the Life and Work of Georg Wilhelm Friedrich Hegel
- German Idealism: A Comprehensive Overview
- Explore The Epicurean School of Ancient Philosophy
- Analytic Philosophy: A Primer
- Exploring Jewish Philosophy
- Exploring the Philosophy of Immanuel Kant
- Exploring British Empiricism
- Justification: A Comprehensive Overview
- Exploring the History and Impact of Empiricism
Enlightenment: A History of Philosophy
- Understanding Fallacies and Rebuttals
Exploring Applied Ethics
- Exploring Critical Thinking and Decision Making
- Exploring Theology: A Comprehensive Overview
- Exploring the Rationalism of Renaissance Philosophy
- Understanding Inductive Reasoning and Analogy
- Exploring Moral Psychology: A Closer Look
- Exploring Identity Theory and Personal Identity
- Exploring Quantitative Research Methods in Philosophy
- Exploring the Analytic-Synthetic Distinction in Philosophy of Language
- Exploring the Role of Research Design and Methodology
- Understanding the Meaning of Words and Phrases
- The Problem of Evil and Suffering: A Philosophical Exploration
- Exploring the Interplay between Religious Experience and Faith
- Exploring the Concepts of Cause, Necessity, Possibility, and Impossibility
- Comparing Analogy, Syllogism, Deduction and Induction
- Exploring Aesthetic Theories: Formalism, Emotionalism and Experientialism
- Understanding Inference, Validity, Soundness, and Refutation
- Exploring Relational Aesthetics, Sociological Aesthetics, and Historical Aesthetics
- Exploring Naturalistic, Immanent and Transcendental Aesthetics
- Exploring Rationalism and Empiricism
- Understanding Determinism, Fatalism, and Libertarianism
- Exploring Identity, Persistence, Time, and Space
- Understanding Virtue Ethics, Utilitarianism and Deontology
- Exploring Phenomenalism and Nominalism
- Ethical Theories: Virtue Ethics, Utilitarianism, and Deontology
- Exploring Justification, Beliefs and Truths
- Foundationalism and Coherentism: An Overview
- Exploring Social Contract Theory, Natural Law Theory, and Care Ethics
- An Overview of Friedrich Nietzsche's Thus Spoke Zarathustra
- Exploring Subjectivism, Egoism and Hedonism
- The Art of Rhetoric by Cicero: A Comprehensive Overview
New Articles
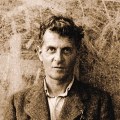
Which cookies do you want to accept?

Scientific Research in Education (2002)
Chapter: 3 guiding principles for scientific inquiry, 3 guiding principles for scientific inquiry.
In Chapter 2 we present evidence that scientific research in education accumulates just as it does in the physical, life, and social sciences. Consequently, we believe that such research would be worthwhile to pursue to build further knowledge about education, and about education policy and practice. Up to this point, however, we have not addressed the questions “What constitutes scientific research?” and “Is scientific research on education different from scientific research in the social, life, and physical sciences?” We do so in this chapter.
These are daunting questions that philosophers, historians, and scientists have debated for several centuries (see Newton-Smith [2000] for a current assessment). Merton (1973), for example, saw commonality among the sciences. He described science as having four aims: universalism, the quest for general laws; organization, the quest to organize and conceptualize a set of related facts or observations; skepticism, the norm of questioning and looking for counter explanations; and communalism, the quest to develop a community that shares a set of norms or principles for doing science. In contrast, some early modern philosophers (the logical positivists) attempted to achieve unity across the sciences by reducing them all to physics, a program that ran into insuperable technical difficulties (Trant, 1991).
In short, we hold that there are both commonalities and differences across the sciences. At a general level, the sciences share a great deal in common, a set of what might be called epistemological or fundamental
principles that guide the scientific enterprise. They include seeking conceptual (theoretical) understanding, posing empirically testable and refutable hypotheses, designing studies that test and can rule out competing counterhypotheses, using observational methods linked to theory that enable other scientists to verify their accuracy, and recognizing the importance of both independent replication and generalization. It is very unlikely that any one study would possess all of these qualities. Nevertheless, what unites scientific inquiry is the primacy of empirical test of conjectures and formal hypotheses using well-codified observation methods and rigorous designs, and subjecting findings to peer review. It is, in John Dewey’s expression, “competent inquiry” that produces what philosophers call “knowledge claims” that are justified or “warranted” by pertinent, empirical evidence (or in mathematics, deductive proof). Scientific reasoning takes place amid (often quantifiable) uncertainty (Schum, 1994); its assertions are subject to challenge, replication, and revision as knowledge is refined over time. The long-term goal of much of science is to produce theory that can offer a stable encapsulation of “facts” that generalizes beyond the particular. In this chapter, then, we spell out what we see as the commonalities among all scientific endeavors.
As our work began, we attempted to distinguish scientific investigations in education from those in the social, physical, and life sciences by exploring the philosophy of science and social science; the conduct of physical, life, and social science investigations; and the conduct of scientific research on education. We also asked a panel of senior government officials who fund and manage research in education and the social and behavioral sciences, and a panel of distinguished scholars from psychometrics, linguistic anthropology, labor economics and law, to distinguish principles of evidence across fields (see National Research Council, 2001d). Ultimately, we failed to convince ourselves that at a fundamental level beyond the differences in specialized techniques and objects of inquiry across the individual sciences, a meaningful distinction could be made among social, physical, and life science research and scientific research in education. At times we thought we had an example that would demonstrate the distinction, only to find our hypothesis refuted by evidence that the distinction was not real.
Thus, the committee concluded that the set of guiding principles that apply to scientific inquiry in education are the same set of principles that
can be found across the full range of scientific inquiry. Throughout this chapter we provide examples from a variety of domains—in political science, geophysics, and education—to demonstrate this shared nature. Although there is no universally accepted description of the elements of scientific inquiry, we have found it convenient to describe the scientific process in terms of six interrelated, but not necessarily ordered, 1 principles of inquiry:
Pose significant questions that can be investigated empirically.
Link research to relevant theory.
Use methods that permit direct investigation of the question.
Provide a coherent and explicit chain of reasoning.
Replicate and generalize across studies.
Disclose research to encourage professional scrutiny and critique.
We choose the phrase “guiding principles” deliberately to emphasize the vital point that they guide, but do not provide an algorithm for, scientific inquiry. Rather, the guiding principles for scientific investigations provide a framework indicating how inferences are, in general, to be supported (or refuted) by a core of interdependent processes, tools, and practices. Although any single scientific study may not fulfill all the principles—for example, an initial study in a line of inquiry will not have been replicated independently—a strong line of research is likely to do so (e.g., see Chapter 2 ).
We also view the guiding principles as constituting a code of conduct that includes notions of ethical behavior. In a sense, guiding principles operate like norms in a community, in this case a community of scientists; they are expectations for how scientific research will be conducted. Ideally, individual scientists internalize these norms, and the community monitors them. According to our analysis these principles of science are common to systematic study in such disciplines as astrophysics, political science, and economics, as well as to more applied fields such as medicine, agriculture, and education. The principles emphasize objectivity, rigorous thinking, open-mindedness, and honest and thorough reporting. Numerous scholars
have commented on the common scientific “conceptual culture” that pervades most fields (see, e.g., Ziman, 2000, p. 145; Chubin and Hackett, 1990).
These principles cut across two dimensions of the scientific enterprise: the creativity, expertise, communal values, and good judgment of the people who “do” science; and generalized guiding principles for scientific inquiry. The remainder of this chapter lays out the communal values of the scientific community and the guiding principles of the process that enable well-grounded scientific investigations to flourish.
THE SCIENTIFIC COMMUNITY
Science is a communal “form of life” (to use the expression of the philosopher Ludwig Wittgenstein [1968]), and the norms of the community take time to learn. Skilled investigators usually learn to conduct rigorous scientific investigations only after acquiring the values of the scientific community, gaining expertise in several related subfields, and mastering diverse investigative techniques through years of practice.
The culture of science fosters objectivity through enforcement of the rules of its “form of life”—such as the need for replicability, the unfettered flow of constructive critique, the desirability of blind refereeing—as well as through concerted efforts to train new scientists in certain habits of mind. By habits of mind, we mean things such as a dedication to the primacy of evidence, to minimizing and accounting for biases that might affect the research process, and to disciplined, creative, and open-minded thinking. These habits, together with the watchfulness of the community as a whole, result in a cadre of investigators who can engage differing perspectives and explanations in their work and consider alternative paradigms. Perhaps above all, the communally enforced norms ensure as much as is humanly possible that individual scientists—while not necessarily happy about being proven wrong—are willing to open their work to criticism, assessment, and potential revision.
Another crucial norm of the scientific “form of life,” which also depends for its efficacy on communal enforcement, is that scientists should be ethical and honest. This assertion may seem trite, even naïve. But scientific knowledge is constructed by the work of individuals, and like any other enterprise, if the people conducting the work are not open and candid, it
can easily falter. Sir Cyril Burt, a distinguished psychologist studying the heritability of intelligence, provides a case in point. He believed so strongly in his hypothesis that intelligence was highly heritable that he “doctored” data from twin studies to support his hypothesis (Tucker, 1994; Mackintosh, 1995); the scientific community reacted with horror when this transgression came to light. Examples of such unethical conduct in such fields as medical research are also well documented (see, e.g., Lock and Wells, 1996).
A different set of ethical issues also arises in the sciences that involve research with animals and humans. The involvement of living beings in the research process inevitably raises difficult ethical questions about a host of potential risks, ranging from confidentiality and privacy concerns to injury and death. Scientists must weigh the relative benefits of what might be learned against the potential risks to human research participants as they strive toward rigorous inquiry. (We consider this issue more fully in Chapters 4 and 6 .)
GUIDING PRINCIPLES
Throughout this report we argue that science is competent inquiry that produces warranted assertions (Dewey, 1938), and ultimately develops theory that is supported by pertinent evidence. The guiding principles that follow provide a framework for how valid inferences are supported, characterize the grounds on which scientists criticize one another’s work, and with hindsight, describe what scientists do. Science is a creative enterprise, but it is disciplined by communal norms and accepted practices for appraising conclusions and how they were reached. These principles have evolved over time from lessons learned by generations of scientists and scholars of science who have continually refined their theories and methods.
SCIENTIFIC PRINCIPLE 1 Pose Significant Questions That Can Be Investigated Empirically
This principle has two parts. The first part concerns the nature of the questions posed: science proceeds by posing significant questions about the world with potentially multiple answers that lead to hypotheses or conjectures that can be tested and refuted. The second part concerns how these questions are posed: they must be posed in such a way that it is
possible to test the adequacy of alternative answers through carefully designed and implemented observations.
Question Significance
A crucial but typically undervalued aspect of successful scientific investigation is the quality of the question posed. Moving from hunch to conceptualization and specification of a worthwhile question is essential to scientific research. Indeed, many scientists owe their renown less to their ability to solve problems than to their capacity to select insightful questions for investigation, a capacity that is both creative and disciplined:
The formulation of a problem is often more essential than its solution, which may be merely a matter of mathematical or experimental skill. To raise new questions, new possibilities, to regard old questions from a new angle, requires creative imagination and marks real advance in science (Einstein and Infeld, 1938, p. 92, quoted in Krathwohl, 1998).
Questions are posed in an effort to fill a gap in existing knowledge or to seek new knowledge, to pursue the identification of the cause or causes of some phenomena, to describe phenomena, to solve a practical problem, or to formally test a hypothesis. A good question may reframe an older problem in light of newly available tools or techniques, methodological or theoretical. For example, political scientist Robert Putnam challenged the accepted wisdom that increased modernity led to decreased civic involvement (see Box 3-1 ) and his work has been challenged in turn. A question may also be a retesting of a hypothesis under new conditions or circumstances; indeed, studies that replicate earlier work are key to robust research findings that hold across settings and objects of inquiry (see Principle 5 ). A good question can lead to a strong test of a theory, however explicit or implicit the theory may be.
The significance of a question can be established with reference to prior research and relevant theory, as well as to its relationship with important claims pertaining to policy or practice. In this way, scientific knowledge grows as new work is added to—and integrated with—the body of material that has come before it. This body of knowledge includes theo-
ries, models, research methods (e.g., designs, measurements), and research tools (e.g., microscopes, questionnaires). Indeed, science is not only an effort to produce representations (models) of real-world phenomena by going from nature to abstract signs. Embedded in their practice, scientists also engage in the development of objects (e.g., instruments or practices); thus, scientific knowledge is a by-product of both technological activities and analytical activities (Roth, 2001). A review of theories and prior research relevant to a particular question can simply establish that it has not been answered before. Once this is established, the review can help shape alternative answers, the design and execution of a study by illuminating if and how the question and related conjectures have already been examined, as well as by identifying what is known about sampling, setting, and other important context. 2
Donald Stokes’ work (Stokes, 1997) provides a useful framework for thinking about important questions that can advance scientific knowledge and method (see Figure 3-1 ). In Pasteur’s Quadrant , he provided evidence that the conception of research-based knowledge as moving in a linear progression from fundamental science to applied science does not reflect how science has historically advanced. He provided several examples demonstrating that, instead, many advancements in science occurred as a result of “use-inspired research,” which simultaneously draws on both basic and applied research. Stokes (1997, p. 63) cites Brooks (1967) on basic and applied work:
Work directed toward applied goals can be highly fundamental in character in that it has an important impact on the conceptual structure or outlook of a field. Moreover, the fact that research is of such a nature that it can be applied does not mean that it is not also basic.

FIGURE 3-1. Quadrant model of scientific research.
SOURCE: Stokes (1997, p. 73). Reprinted with permission.
Stokes’ model clearly applies to research in education, where problems of practice and policy provide a rich source for important—and often highly fundamental in character—research questions.
Empirically Based
Put simply, the term “empirical” means based on experience through the senses, which in turn is covered by the generic term observation. Since science is concerned with making sense of the world, its work is necessarily grounded in observations that can be made about it. Thus, research questions
must be posed in ways that potentially allow for empirical investigation. 3 For example, both Milankovitch and Muller could collect data on the Earth’s orbit to attempt to explain the periodicity in ice ages (see Box 3-2 ). Likewise, Putnam could collect data from natural variations in regional government to address the question of whether modernization leads to the demise of civic community ( Box 3-1 ), and the Tennessee state legislature could empirically assess whether reducing class size improves students’ achievement in early grades ( Box 3-3 ) because achievement data could be collected on students in classes of varying sizes. In contrast, questions such as: “ Should all students be required to say the pledge of allegiance?” cannot be submitted to empirical investigation and thus cannot be examined scientifically. Answers to these questions lie in realms other than science.
SCIENTIFIC PRINCIPLE 2 Link Research to Relevant Theory
Scientific theories are, in essence, conceptual models that explain some phenomenon. They are “nets cast to catch what we call ‘the world’…we endeavor to make the mesh ever finer and finer” (Popper, 1959, p. 59). Indeed, much of science is fundamentally concerned with developing and testing theories, hypotheses, models, conjectures, or conceptual frameworks that can explain aspects of the physical and social world. Examples of well-known scientific theories include evolution, quantum theory, and the theory of relativity.
In the social sciences and in education, such “grand” theories are rare. To be sure, generalized theoretical understanding is still a goal. However, some research in the social sciences seeks to achieve deep understanding of particular events or circumstances rather than theoretical understanding that will generalize across situations or events. Between these extremes lies the bulk of social science theory or models, what Merton (1973) called
mid-range theories that attempt to account for some aspect of the social world. Examples of such mid-range theories or explanatory models can be found in the physical and the social sciences.
These theories are representations or abstractions of some aspect of reality that one can only approximate by such models. Molecules, fields, or black holes are classic explanatory models in physics; the genetic code and the contractile filament model of muscle are two in biology. Similarly,
culture, socioeconomic status, and poverty are classical models in anthropology, sociology, and political science. In program evaluation, program developers have ideas about the mechanism by which program inputs affect targeted outcomes; evaluations translate and test these ideas through a “program theory” that guides the work (Weiss, 1998a).
Theory enters the research process in two important ways. First, scientific research may be guided by a conceptual framework, model, or theory
that suggests possible questions to ask or answers to the question posed. 4 The process of posing significant questions typically occurs before a study is conducted. Researchers seek to test whether a theory holds up under certain circumstances. Here the link between question and theory is straightforward. For example, Putnam based his work on a theoretical conception of institutional performance that related civic engagement and modernization.
A research question can also devolve from a practical problem (Stokes, 1997; see discussion above). In this case, addressing a complex problem like the relationship between class size and student achievement may require several theories. Different theories may give conflicting predictions about the problem’s solution, or various theories might have to be reconciled to address the problem. Indeed, the findings from the Tennessee class size reduction study (see Box 3-3 ) have led to several efforts to devise theoretical understandings of how class size reduction may lead to better student achievement. Scientists are developing models to understand differences in classroom behavior between large and small classes that may ultimately explain and predict changes in achievement (Grissmer and Flannagan, 2000).
A second more subtle way that theoretical understanding factors into the research process derives from the fact that all scientific observations are “theory laden” (Kuhn, 1962). That is, the choice of what to observe and how to observe it is driven by an organizing conception—explicit or tacit— of the problem or topic. Thus, theory drives the research question, the use of methods, and the interpretation of results.
SCIENTIFIC PRINCIPLE 3
Use methods that permit direct investigation of the question.
Research methods—the design for collecting data and the measurement and analysis of variables in the design—should be selected in light of a research question, and should address it directly. Methods linked directly to problems permit the development of a logical chain of reasoning based
on the interplay among investigative techniques, data, and hypotheses to reach justifiable conclusions. For clarity of discussion, we separate out the link between question and method (see Principle 3 ) and the rigorous reasoning from evidence to theory (see Principle 4 ). In the actual practice of research, such a separation cannot be achieved.
Debates about method—in many disciplines and fields—have raged for centuries as researchers have battled over the relative merit of the various techniques of their trade. The simple truth is that the method used to conduct scientific research must fit the question posed, and the investigator must competently implement the method. Particular methods are better suited to address some questions rather than others. The rare choice in the mid 1980s in Tennessee to conduct a randomized field trial, for example, enabled stronger inferences about the effects of class size reduction on student achievement (see Box 3-3 ) than would have been possible with other methods.
This link between question and method must be clearly explicated and justified; a researcher should indicate how a particular method will enable competent investigation of the question of interest. Moreover, a detailed description of method—measurements, data collection procedures, and data analyses—must be available to permit others to critique or replicate the study (see Principle 5 ). Finally, investigators should identify potential methodological limitations (such as insensitivity to potentially important variables, missing data, and potential researcher bias).
The choice of method is not always straightforward because, across all disciplines and fields, a wide range of legitimate methods—both quantitative and qualitative—are available to the researcher. For example when considering questions about the natural universe—from atoms to cells to black holes—profoundly different methods and approaches characterize each sub-field. While investigations in the natural sciences are often dependent on the use of highly sophisticated instrumentation (e.g., particle accelerators, gene sequencers, scanning tunneling microscopes), more rudimentary methods often enable significant scientific breakthroughs. For example, in 1995 two Danish zoologists identified an entirely new phylum of animals from a species of tiny rotifer-like creatures found living on the mouthparts of lobsters, using only a hand lens and light microscope (Wilson, 1998, p. 63).
If a research conjecture or hypothesis can withstand scrutiny by multiple methods its credibility is enhanced greatly. As Webb, Campbell, Schwartz, and Sechrest (1966, pp. 173-174) phrased it: “When a hypothesis can survive the confrontation of a series of complementary methods of testing, it contains a degree of validity unattainable by one tested within the more constricted framework of a single method.” Putnam’s study (see Box 3-1 ) provides an example in which both quantitative and qualitative methods were applied in a longitudinal design (e.g., interview, survey, statistical estimate of institutional performance, analysis of legislative docu-
ments) to generate converging evidence about the effects of modernization on civic community. New theories about the periodicity of the ice ages, similarly, were informed by multiple methods (e.g., astronomical observations of cosmic dust, measurements of oxygen isotopes). The integration and interaction of multiple disciplinary perspectives—with their varying methods—often accounts for scientific progress (Wilson, 1998); this is evident, for example, in the advances in understanding early reading skills described in Chapter 2 . This line of work features methods that range from neuroimaging to qualitative classroom observation.
We close our discussion of this principle by noting that in many sciences, measurement is a key aspect of research method. This is true for many research endeavors in the social sciences and education research, although not for all of them. If the concepts or variables are poorly specified or inadequately measured, even the best methods will not be able to support strong scientific inferences. The history of the natural sciences is one of remarkable development of concepts and variables, as well as the tools (instrumentation) to measure them. Measurement reliability and validity is particularly challenging in the social sciences and education (Messick, 1989). Sometimes theory is not strong enough to permit clear specification and justification of the concept or variable. Sometimes the tool (e.g., multiple-choice test) used to take the measurement seriously under-represents the construct (e.g., science achievement) to be measured. Sometimes the use of the measurement has an unintended social consequence (e.g., the effect of teaching to the test on the scope of the curriculum in schools).
And sometimes error is an inevitable part of the measurement process. In the physical sciences, many phenomena can be directly observed or have highly predictable properties; measurement error is often minimal. (However, see National Research Council [1991] for a discussion of when and how measurement in the physical sciences can be imprecise.) In sciences that involve the study of humans, it is essential to identify those aspects of measurement error that attenuate the estimation of the relationships of interest (e.g., Shavelson, Baxter, and Gao, 1993). By investigating those aspects of a social measurement that give rise to measurement error, the measurement process itself will often be improved. Regardless of field of study, scientific measurements should be accompanied by estimates of uncertainty whenever possible (see Principle 4 below).
SCIENTIFIC PRINCIPLE 4 Provide Coherent, Explicit Chain of Reasoning
The extent to which the inferences that are made in the course of scientific work are warranted depends on rigorous reasoning that systematically and logically links empirical observations with the underlying theory and the degree to which both the theory and the observations are linked to the question or problem that lies at the root of the investigation. There
is no recipe for determining how these ingredients should be combined; instead, what is required is the development of a logical “chain of reasoning” (Lesh, Lovitts, and Kelly, 2000) that moves from evidence to theory and back again. This chain of reasoning must be coherent, explicit (one that another researcher could replicate), and persuasive to a skeptical reader (so that, for example, counterhypotheses are addressed).
All rigorous research—quantitative and qualitative—embodies the same underlying logic of inference (King, Keohane, and Verba, 1994). This inferential reasoning is supported by clear statements about how the research conclusions were reached: What assumptions were made? How was evidence judged to be relevant? How were alternative explanations considered or discarded? How were the links between data and the conceptual or theoretical framework made?
The nature of this chain of reasoning will vary depending on the design of the study, which in turn will vary depending on the question that is being investigated. Will the research develop, extend, modify, or test a hypothesis? Does it aim to determine: What works? How does it work? Under what circumstances does it work? If the goal of the research is to test a hypothesis, stated in the form of an “if-then” rule, successful inference may depend on measuring the extent to which the rule predicts results under a variety of conditions. If the goal is to produce a description of a complex system, such as a subcellular organelle or a hierarchical social organization, successful inference may rather depend on issues of fidelity and internal consistency of the observational techniques applied to diverse components and the credibility of the evidence gathered. The research design and the inferential reasoning it enables must demonstrate a thorough understanding of the subtleties of the questions to be asked and the procedures used to answer them.
Muller (1994), for example, collected data on the inclination of the Earth’s orbit over a 100,000 year cycle, correlated it with the occurrence of ice ages, ruled out the plausibility of orbital eccentricity as a cause for the occurrence of ice ages, and inferred that the bounce in the Earth’s orbit likely caused the ice ages (see Box 3-2 ). Putnam used multiple methods to subject to rigorous testing his hypotheses about what affects the success or failure of democratic institutions as they develop in diverse social environments to rigorous testing, and found the weight of the evidence favored
the assertion that civic tradition matters more than economic affluence (see Box 3-1 ). And Baumeister, Bratslavsky, Muraven, and Tice (1998) compared three competing theories and used randomized experiments to conclude that a “psychic energy” hypothesis best explained the important psychological characteristic of “will power” (see “ Application of the Principles ”).
This principle has several features worthy of elaboration. Assumptions underlying the inferences made should be clearly stated and justified. Moreover, choice of design should both acknowledge potential biases and plan for implementation challenges.
Estimates of error must also be made. Claims to knowledge vary substantially according to the strength of the research design, theory, and control of extraneous variables and by systematically ruling out possible alternative explanations. Although scientists always reason in the presence of uncertainty, it is critical to gauge the magnitude of this uncertainty. In the physical and life sciences, quantitative estimates of the error associated with conclusions are often computed and reported. In the social sciences and education, such quantitative measures are sometimes difficult to generate; in any case, a statement about the nature and estimated magnitude of error must be made in order to signal the level of certainty with which conclusions have been drawn.
Perhaps most importantly, the reasoning about evidence should identify, consider, and incorporate, when appropriate, the alternative, competing explanations or rival “answers” to the research question. To make valid inferences, plausible counterexplanations must be dealt with in a rational, systematic, and compelling way. 5 The validity—or credibility—of a hypothesis is substantially strengthened if alternative counterhypotheses can be ruled out and the favored one thereby supported. Well-known research designs (e.g., Campbell and Stanley [1963] in educational psychology; Heckman [1979, 1980a, 1980b, 2001] and Goldberger [1972, 1983] in
economics; and Rosenbaum and Rubin [1983, 1984] in statistics) have been crafted to guard researchers against specific counterhypotheses (or “threats to validity”). One example, often called “selectivity bias,” is the counterhypothesis that differential selection (not the treatment) caused the outcome—that participants in the experimental treatment systematically differed from participants in the traditional (control) condition in ways that mattered importantly to the outcome. A cell biologist, for example, might unintentionally place (select) heart cells with a slight glimmer into an experimental group and others into a control group, thus potentially biasing the comparison between the groups of cells. The potential for a biased—or unfair—comparison arises because the shiny cells could differ systematically from the others in ways that affect what is being studied.
Selection bias is a pervasive problem in the social sciences and education research. To illustrate, in studying the effects of class-size reduction, credentialed teachers are more likely to be found in wealthy school districts that have the resources to reduce class size than in poor districts. This fact raises the possibility that higher achievement will be observed in the smaller classes due to factors other than class size (e.g.. teacher effects). Random assignment to “treatment” is the strongest known antidote to the problem of selection bias (see Chapter 5 ).
A second counterhypothesis contends that something in the research participants’ history that co-occurred with the treatment caused the outcome, not the treatment itself. For example, U.S. fourth-grade students outperformed students in others countries on the ecology subtest of the Third International Mathematics and Science Study. One (popular) explanation of this finding was that the effect was due to their schooling and the emphasis on ecology in U.S. elementary science curricula. A counter-hypothesis, one of history, posits that their high achievement was due to the prevalence of ecology in children’s television programming. A control group that has the same experiences as the experimental group except for the “treatment” under study is the best antidote for this problem.
A third prevalent class of alternative interpretations contends that an outcome was biased by the measurement used. For example, education effects are often judged by narrowly defined achievement tests that focus on factual knowledge and therefore favor direct-instruction teaching tech-
niques. Multiple achievement measures with high reliability (consistency) and validity (accuracy) help to counter potential measurement bias.
The Tennessee class-size study was designed primarily to eliminate all possible known explanations, except for reduced class size, in comparing the achievement of children in regular classrooms against achievement in reduced size classrooms. It did this. Complications remained, however. About ten percent of students moved out of their originally assigned condition (class size), weakening the design because the comparative groups did not remain intact to enable strict comparisons. However, most scholars who subsequently analyzed the data (e.g., Krueger and Whitmore, 2001), while limited by the original study design, suggested that these infidelities did not affect the main conclusions of the study that smaller class size caused slight improvements in achievement. Students in classes of 13-17 students outperformed their peers in larger classes, on average, by a small margin.
SCIENTIFIC PRINCIPLE 5 Replicate and Generalize Across Studies
Replication and generalization strengthen and clarify the limits of scientific conjectures and theories. By replication we mean, at an elementary level, that if one investigator makes a set of observations, another investigator can make a similar set of observations under the same conditions. Replication in this sense comes close to what psychometricians call reliability—consistency of measurements from one observer to another, from one task to another parallel task, from one occasion to another occasion. Estimates of these different types of reliability can vary when measuring a given construct: for example, in measuring performance of military personnel (National Research Council, 1991), multiple observers largely agreed on what they observed within tasks; however, enlistees’ performance across parallel tasks was quite inconsistent.
At a somewhat more complex level, replication means the ability to repeat an investigation in more than one setting (from one laboratory to another or from one field site to a similar field site) and reach similar conclusions. To be sure, replication in the physical sciences, especially with inanimate objects, is more easily achieved than in social science or education; put another way, the margin of error in social science replication is usually
much greater than in physical science replication. The role of contextual factors and the lack of control that characterizes work in the social realm require a more nuanced notion of replication. Nevertheless, the typically large margins of error in social science replications do not preclude their identification.
Having evidence of replication, an important goal of science is to understand the extent to which findings generalize from one object or person to another, from one setting to another, and so on. To this end, a substantial amount of statistical machinery has been built both to help ensure that what is observed in a particular study is representative of what is of larger interest (i.e., will generalize) and to provide a quantitative measure of the possible error in generalizing. Nonstatistical means of generalization (e.g., triangulation, analytic induction, comparative analysis) have also been developed and applied in genres of research, such as ethnography, to understand the extent to which findings generalize across time, space, and populations. Subsequent applications, implementations, or trials are often necessary to assure generalizability or to clarify its limits. For example, since the Tennessee experiment, additional studies of the effects of class size reduction on student learning have been launched in settings other than Tennessee to assess the extent to which the findings generalize (e.g., Hruz, 2000).
In the social sciences and education, many generalizations are limited to particular times and particular places (Cronbach, 1975). This is because the social world undergoes rapid and often significant change; social generalizations, as Cronbach put it, have a shorter “half-life” than those in the physical world. Campbell and Stanley (1963) dubbed the extent to which the treatment conditions and participant population of a study mirror the world to which generalization is desired the “external validity” of the study. Consider, again, the Tennessee class-size research; it was undertaken in a set of schools that had the desire to participate, the physical facilities to accommodate an increased number of classrooms, and adequate teaching staff. Governor Wilson of California “overgeneralized” the Tennessee study, ignoring the specific experimental conditions of will and capacity and implemented class-size reduction in more than 95 percent of grades K-3 in the state. Not surprisingly, most researchers studying California have
concluded that the Tennessee findings did not entirely generalize to a different time, place, and context (see, e.g., Stecher and Bohrnstedt, 2000). 6
SCIENTIFIC PRINCIPLE 6 Disclose Research to Encourage Professional Scrutiny and Critique
We argue in Chapter 2 that a characteristic of scientific knowledge accumulation is its contested nature. Here we suggest that science is not only characterized by professional scrutiny and criticism, but also that such criticism is essential to scientific progress. Scientific studies usually are elements of a larger corpus of work; furthermore, the scientists carrying out a particular study always are part of a larger community of scholars. Reporting and reviewing research results are essential to enable wide and meaningful peer review. Results are traditionally published in a specialty journal, in books published by academic presses, or in other peer-reviewed publications. In recent years, an electronic version may accompany or even substitute for a print publication. 7 Results may be debated at professional conferences. Regardless of the medium, the goals of research reporting are to communicate the findings from the investigation; to open the study to examination, criticism, review, and replication (see Principle 5 ) by peer investigators; and ultimately to incorporate the new knowledge into the prevailing canon of the field. 8
The goal of communicating new knowledge is self-evident: research results must be brought into the professional and public domain if they are to be understood, debated, and eventually become known to those who could fruitfully use them. The extent to which new work can be reviewed and challenged by professional peers depends critically on accurate, comprehensive, and accessible records of data, method, and inferential reasoning. This careful accounting not only makes transparent the reasoning that led to conclusions—promoting its credibility—but it also allows the community of scientists and analysts to comprehend, to replicate, and otherwise to inform theory, research, and practice in that area.
Many nonscientists who seek guidance from the research community bemoan what can easily be perceived as bickering or as an indication of “bad” science. Quite the contrary: intellectual debate at professional meetings, through research collaborations, and in other settings provide the means by which scientific knowledge is refined and accepted; scientists strive for an “open society” where criticism and unfettered debate point the way to advancement. Through scholarly critique (see, e.g., Skocpol, 1996) and debate, for example, Putnam’s work has stimulated a series of articles, commentary, and controversy in research and policy circles about the role of “social capital” in political and other social phenomena (Winter, 2000). And the Tennessee class size study has been the subject of much scholarly debate, leading to a number of follow-on analyses and launching new work that attempts to understand the process by which classroom behavior may shift in small classes to facilitate learning. However, as Lagemann (2000) has observed, for many reasons the education research community has not been nearly as critical of itself as is the case in other fields of scientific study.
APPLICATION OF THE PRINCIPLES
The committee considered a wide range of literature and scholarship to test its ideas about the guiding principles. We realized, for example, that empiricism, while a hallmark of science, does not uniquely define it. A poet can write from first-hand experience of the world, and in this sense is an empiricist. And making observations of the world, and reasoning about their experience, helps both literary critics and historians create the
interpretive frameworks that they bring to bear in their scholarship. But empirical method in scientific inquiry has different features, like codified procedures for making observations and recognizing sources of bias associated with particular methods, 9 and the data derived from these observations are used specifically as tools to support or refute knowledge claims. Finally, empiricism in science involves collective judgments based on logic, experience, and consensus.
Another hallmark of science is replication and generalization. Humanists do not seek replication, although they often attempt to create work that generalizes (say) to the “human condition.” However, they have no formal logic of generalization, unlike scientists working in some domains (e.g., statistical sampling theory). In sum, it is clear that there is no bright line that distinguishes science from nonscience or high-quality science from low-quality science. Rather, our principles can be used as general guidelines for understanding what can be considered scientific and what can be considered high-quality science (see, however, Chapters 4 and 5 for an elaboration).
To show how our principles help differentiate science from other forms of scholarship, we briefly consider two genres of education inquiry published in refereed journals and books. We do not make a judgment about the worth of either form of inquiry; although we believe strongly in the merits of scientific inquiry in education research and more generally, that “science” does not mean “good.” Rather, we use them as examples to illustrate the distinguishing character of our principles of science. The first— connoisseurship —grew out of the arts and humanities (e.g., Eisner, 1991) and does not claim to be scientific. The second— portraiture —claims to straddle the fence between humanistic and scientific inquiry (e.g., Lawrence-Lightfoot and Davis, 1997).
Eisner (1991, p. 7) built a method for education inquiry firmly rooted in the arts and humanities, arguing that “there are multiple ways in which the world can be known: Artists, writers, and dancers, as well as scientists, have important things to tell about the world.” His method of inquiry combines connoisseurship (the art of appreciation), which “aims to
appreciate the qualities . . . that constitute an act, work, or object and, typically . . . to relate these to the contextual and antecedent conditions” (p. 85) with educational criticism (the art of disclosure), which provides “connoisseurship with a public face” (p. 85). The goal of this genre of research is to enable readers to enter an event and to participate in it. To this end, the educational critic—through educational connoisseurship— must capture the key qualities of the material, situation, and experience and express them in text (“criticism”) to make what the critic sees clear to others. “To know what schools are like, their strengths and their weaknesses, we need to be able to see what occurs in them, and we need to be able to tell others what we have seen in ways that are vivid and insightful” (Eisner, 1991, p. 23, italics in original).
The grounds for his knowledge claims are not those in our guiding principles. Rather, credibility is established by: (1) structural corroboration—“multiple types of data are related to each other” (p. 110) and “ disconfirming evidence and contradictory interpretations ” (p. 111; italics in original) are considered; (2) consensual validation—“agreement among competent others that the description, interpretation, evaluation, and thematics of an educational situation are right” (p. 112); and (3) referential adequacy— “the extent to which a reader is able to locate in its subject matter the qualities the critic addresses and the meanings he or she ascribes to these” (p. 114). While sharing some features of our guiding principles (e.g., ruling out counterinterpretations to the favored interpretation), this humanistic approach to knowledge claims builds on a very different epistemology; the key scientific concepts of reliability, replication, and generalization, for example, are quite different. We agree with Eisner that such approaches fall outside the purview of science and conclude that our guiding principles readily distinguish them.
Portraiture (Lawrence-Lightfoot, 1994; Lawrence-Lightfoot and Davis, 1997) is a qualitative research method that aims to “record and interpret the perspectives and experience of the people they [the researchers] are studying, documenting their [the research participants’] voices and their visions—their authority, knowledge, and wisdom” (Lawrence-Lightfoot and Davis, 1997, p. xv). In contrast to connoisseurship’s humanist orientation, portraiture “seeks to join science and art” (Lawrence-Lightfoot and Davis, 1997, p. xv) by “embracing the intersection of aesthetics and empiricism” (p. 6). The standard for judging the quality of portraiture is authenticity,
“. . . capturing the essence and resonance of the actors’ experience and perspective through the details of action and thought revealed in context” (p. 12). When empirical and literary themes come together (called “resonance”) for the researcher, the actors, and the audience, “we speak of the portrait as achieving authenticity” (p. 260).
In I’ve Known Rivers , Lawrence-Lightfoot (1994) explored the life stories of six men and women:
. . . using the intensive, probing method of ‘human archeology’—a name I [Lawrence-Lightfoot] coined for this genre of portraiture as a way of trying to convey the depth and penetration of the inquiry, the richness of the layers of human experience, the search for ancestral and generational artifacts, and the painstaking, careful labor that the metaphorical dig requires. As I listen to the life stories of these individuals and participate in the ‘co-construction’ of narrative, I employ the themes, goals, and techniques of portraiture. It is an eclectic, interdisciplinary approach, shaped by the lenses of history, anthropology, psychology and sociology. I blend the curiosity and detective work of a biographer, the literary aesthetic of a novelist, and the systematic scrutiny of a researcher (p. 15).
Some scholars, then, deem portraiture as “scientific” because it relies on the use of social science theory and a form of empiricism (e.g., interview). While both empiricism and theory are important elements of our guiding principles, as we discuss above, they are not, in themselves, defining. The devil is in the details. For example, independent replication is an important principle in our framework but is absent in portraiture in which researcher and subject jointly construct a narrative. Moreover, even when our principles are manifest, the specific form and mode of application can make a big difference. For example, generalization in our principles is different from generalization in portraiture. As Lawrence-Lightfoot and Davis (1997) point out, generalization as used in the social sciences does not fit portraiture. Generalization in portraiture “. . . is not the classical conception . . . where the investigator uses codified methods for generalizing from specific findings to a universe, and where there is little interest in findings that reflect only the characteristics of the sample. . . .” By contrast, the portraitist seeks to “document and illuminate the complexity
and detail of a unique experience or place, hoping the audience will see itself reflected in it, trusting that the readers will feel identified. The portraitist is very interested in the single case because she believes that embedded in it the reader will discover resonant universal themes” (p. 15). We conclude that our guiding principles would distinguish portraiture from what we mean by scientific inquiry, although it, like connoisseurship, has some traits in common.
To this point, we have shown how our principles help to distinguish science and nonscience. A large amount of education research attempts to base knowledge claims on science; clearly, however, there is great variation with respect to scientific rigor and competence. Here we use two studies to illustrate how our principles demonstrate this gradation in scientific quality.
The first study (Carr, Levin, McConnachie, Carlson, Kemp, Smith, and McLaughlin, 1999) reported on an educational intervention carried out on three nonrandomly selected individuals who were suffering severe behavioral disorders and who were residing in group-home settings. Since earlier work had established remedial procedures involving “simulations and analogs of the natural environment” (p. 6), the focus of the study was on the generalizability (or external validity) to the “real world” of the intervention (places, caregivers).
Over a two to three week period, “baseline” frequencies of their problem behaviors were established, these behaviors were remeasured after an intervention lasting for some years was carried out. The researchers took a third measurement during the maintenance phase of the study. While care was taken in describing behavioral observations, variable construction and reliability, the paper reporting on the study did not provide clear, detailed depictions of the interventions or who carried them out (research staff or staff of the group homes). Furthermore, no details were given of the changes in staffing or in the regimens of the residential settings—changes that were inevitable over a period of many years (the timeline itself was not clearly described). Finally, in the course of daily life over a number of years, many things would have happened to each of the subjects, some of which might be expected to be of significance to the study, but none of them were documented. Over the years, too, one might expect some developmental changes to occur in the aggressive behavior displayed by the research subjects, especially in the two teenagers. In short, the study focused on
generalizability at too great an expense relative to internal validity. In the end, there were many threats to internal validity in this study, and so it is impossible to conclude (as the authors did) from the published report that the “treatment” had actually caused the improvement in behavior that was noted.
Turning to a line of work that we regard as scientifically more successful, in a series of four randomized experiments, Baumeister, Bratslavsky, Muraven, and Tice (1998) tested three competing theories of “will power” (or, more technically, “self-regulation”)—the psychological characteristic that is posited to be related to persistence with difficult tasks such as studying or working on homework assignments. One hypothesis was that will power is a developed skill that would remain roughly constant across repeated trials. The second theory posited a self-control schema “that makes use of information about how to alter one’s own response” (p. 1254) so that once activated on one trial, it would be expected to increase will power on a second trial. The third theory, anticipated by Freud’s notion of the ego exerting energy to control the id and superego, posits that will power is a depletable resource—it requires the use of “psychic energy” so that performance from trial 1 to trial 2 would decrease if a great deal of will power was called for on trial 1. In one experiment, 67 introductory psychology students were randomly assigned to a condition in which either no food was present or both radishes and freshly baked chocolate chip cookies were present, and the participants were instructed either to eat two or three radishes (resisting the cookies) or two or three cookies (resisting the radishes). Immediately following this situation, all participants were asked to work on two puzzles that unbeknownst to them, were unsolvable, and their persistence (time) in working on the puzzles was measured. The experimental manipulation was checked for every individual participating by researchers observing their behavior through a one-way window. The researchers found that puzzle persistence was the same in the control and cookie conditions and about 2.5 times as long, on average, as in the radish condition, lending support to the psychic energy theory—arguably, resisting the temptation to eat the cookies evidently had depleted the reserve of self-control, leading to poor performance on the second task. Later experiments extended the findings supporting the energy theory to situations involving choice, maladaptive performance, and decision making.
However, as we have said, no single study or series of studies satisfy all of our guiding principles, and these will power experiments are no exception. They all employed small samples of participants, all drawn from a college population. The experiments were contrived—the conditions of the study would be unlikely outside a psychology laboratory. And the question of whether these findings would generalize to more realistic (e.g., school) settings was not addressed.
Nevertheless, the contrast in quality between the two studies, when observed through the lens of our guiding principles, is stark. Unlike the first study, the second study was grounded in theory and identified three competing answers to the question of self-regulation, each leading to a different empirically refutable claim. In doing so, the chain of reasoning was made transparent. The second study, unlike the first, used randomized experiments to address counterclaims to the inference of psychic energy, such as selectivity bias or different history during experimental sessions. Finally, in the second study, the series of experiments replicated and extended the effects hypothesized by the energy theory.
CONCLUDING COMMENT
Nearly a century ago, John Dewey (1916) captured the essence of the account of science we have developed in this chapter and expressed a hopefulness for the promise of science we similarly embrace:
Our predilection for premature acceptance and assertion, our aversion to suspended judgment, are signs that we tend naturally to cut short the process of testing. We are satisfied with superficial and immediate short-visioned applications. If these work out with moderate satisfactoriness, we are content to suppose that our assumptions have been confirmed. Even in the case of failure, we are inclined to put the blame not on the inadequacy and incorrectness of our data and thoughts, but upon our hard luck and the hostility of circumstances. . . . Science represents the safeguard of the [human] race against these natural propensities and the evils which flow from them. It consists of the special appliances and methods... slowly worked out in order to conduct reflection under conditions whereby its procedures and results are tested.
Researchers, historians, and philosophers of science have debated the nature of scientific research in education for more than 100 years. Recent enthusiasm for "evidence-based" policy and practice in education—now codified in the federal law that authorizes the bulk of elementary and secondary education programs—have brought a new sense of urgency to understanding the ways in which the basic tenets of science manifest in the study of teaching, learning, and schooling.
Scientific Research in Education describes the similarities and differences between scientific inquiry in education and scientific inquiry in other fields and disciplines and provides a number of examples to illustrate these ideas. Its main argument is that all scientific endeavors share a common set of principles, and that each field—including education research—develops a specialization that accounts for the particulars of what is being studied. The book also provides suggestions for how the federal government can best support high-quality scientific research in education.
Welcome to OpenBook!
You're looking at OpenBook, NAP.edu's online reading room since 1999. Based on feedback from you, our users, we've made some improvements that make it easier than ever to read thousands of publications on our website.
Do you want to take a quick tour of the OpenBook's features?
Show this book's table of contents , where you can jump to any chapter by name.
...or use these buttons to go back to the previous chapter or skip to the next one.
Jump up to the previous page or down to the next one. Also, you can type in a page number and press Enter to go directly to that page in the book.
Switch between the Original Pages , where you can read the report as it appeared in print, and Text Pages for the web version, where you can highlight and search the text.
To search the entire text of this book, type in your search term here and press Enter .
Share a link to this book page on your preferred social network or via email.
View our suggested citation for this chapter.
Ready to take your reading offline? Click here to buy this book in print or download it as a free PDF, if available.
Get Email Updates
Do you enjoy reading reports from the Academies online for free ? Sign up for email notifications and we'll let you know about new publications in your areas of interest when they're released.

An official website of the United States government
The .gov means it’s official. Federal government websites often end in .gov or .mil. Before sharing sensitive information, make sure you’re on a federal government site.
The site is secure. The https:// ensures that you are connecting to the official website and that any information you provide is encrypted and transmitted securely.
- Publications
- Account settings
Preview improvements coming to the PMC website in October 2024. Learn More or Try it out now .
- Advanced Search
- Journal List

What influences students’ abilities to critically evaluate scientific investigations?
Ashley b. heim.
1 Department of Ecology and Evolutionary Biology, Cornell University, Ithaca, NY, United States of America
2 Laboratory of Atomic and Solid State Physics, Cornell University, Ithaca, NY, United States of America
David Esparza
Michelle k. smith, n. g. holmes, associated data.
All raw data files are available from the Cornell Institute for Social and Economic Research (CISER) data and reproduction archive ( https://archive.ciser.cornell.edu/studies/2881 ).
Critical thinking is the process by which people make decisions about what to trust and what to do. Many undergraduate courses, such as those in biology and physics, include critical thinking as an important learning goal. Assessing critical thinking, however, is non-trivial, with mixed recommendations for how to assess critical thinking as part of instruction. Here we evaluate the efficacy of assessment questions to probe students’ critical thinking skills in the context of biology and physics. We use two research-based standardized critical thinking instruments known as the Biology Lab Inventory of Critical Thinking in Ecology (Eco-BLIC) and Physics Lab Inventory of Critical Thinking (PLIC). These instruments provide experimental scenarios and pose questions asking students to evaluate what to trust and what to do regarding the quality of experimental designs and data. Using more than 3000 student responses from over 20 institutions, we sought to understand what features of the assessment questions elicit student critical thinking. Specifically, we investigated (a) how students critically evaluate aspects of research studies in biology and physics when they are individually evaluating one study at a time versus comparing and contrasting two and (b) whether individual evaluation questions are needed to encourage students to engage in critical thinking when comparing and contrasting. We found that students are more critical when making comparisons between two studies than when evaluating each study individually. Also, compare-and-contrast questions are sufficient for eliciting critical thinking, with students providing similar answers regardless of if the individual evaluation questions are included. This research offers new insight on the types of assessment questions that elicit critical thinking at the introductory undergraduate level; specifically, we recommend instructors incorporate more compare-and-contrast questions related to experimental design in their courses and assessments.
Introduction
Critical thinking and its importance.
Critical thinking, defined here as “the ways in which one uses data and evidence to make decisions about what to trust and what to do” [ 1 ], is a foundational learning goal for almost any undergraduate course and can be integrated in many points in the undergraduate curriculum. Beyond the classroom, critical thinking skills are important so that students are able to effectively evaluate data presented to them in a society where information is so readily accessible [ 2 , 3 ]. Furthermore, critical thinking is consistently ranked as one of the most necessary outcomes of post-secondary education for career advancement by employers [ 4 ]. In the workplace, those with critical thinking skills are more competitive because employers assume they can make evidence-based decisions based on multiple perspectives, keep an open mind, and acknowledge personal limitations [ 5 , 6 ]. Despite the importance of critical thinking skills, there are mixed recommendations on how to elicit and assess critical thinking during and as a result of instruction. In response, here we evaluate the degree to which different critical thinking questions elicit students’ critical thinking skills.
Assessing critical thinking in STEM
Across STEM (i.e., science, technology, engineering, and mathematics) disciplines, several standardized assessments probe critical thinking skills. These assessments focus on aspects of critical thinking and ask students to evaluate experimental methods [ 7 – 11 ], form hypotheses and make predictions [ 12 , 13 ], evaluate data [ 2 , 12 – 14 ], or draw conclusions based on a scenario or figure [ 2 , 12 – 14 ]. Many of these assessments are open-response, so they can be difficult to score, and several are not freely available.
In addition, there is an ongoing debate regarding whether critical thinking is a domain-general or context-specific skill. That is, can someone transfer their critical thinking skills from one domain or context to another (domain-general) or do their critical thinking skills only apply in their domain or context of expertise (context-specific)? Research on the effectiveness of teaching critical thinking has found mixed results, primarily due to a lack of consensus definition of and assessment tools for critical thinking [ 15 , 16 ]. Some argue that critical thinking is domain-general—or what Ennis refers to as the “general approach”—because it is an overlapping skill that people use in various aspects of their lives [ 17 ]. In contrast, others argue that critical thinking must be elicited in a context-specific domain, as prior knowledge is needed to make informed decisions in one’s discipline [ 18 , 19 ]. Current assessments include domain-general components [ 2 , 7 , 8 , 14 , 20 , 21 ], asking students to evaluate, for instance, experiments on the effectiveness of dietary supplements in athletes [ 20 ] and context-specific components, such as to measure students’ abilities to think critically in domains such as neuroscience [ 9 ] and biology [ 10 ].
Others maintain the view that critical thinking is a context-specific skill for the purpose of undergraduate education, but argue that it should be content accessible [ 22 – 24 ], as “thought processes are intertwined with what is being thought about” [ 23 ]. From this viewpoint, the context of the assessment would need to be embedded in a relatively accessible context to assess critical thinking independent of students’ content knowledge. Thus, to effectively elicit critical thinking among students, instructors should use assessments that present students with accessible domain-specific information needed to think deeply about the questions being asked [ 24 , 25 ].
Within the context of STEM, current critical thinking assessments primarily ask students to evaluate a single experimental scenario (e.g., [ 10 , 20 ]), though compare-and-contrast questions about more than one scenario can be a powerful way to elicit critical thinking [ 26 , 27 ]. Generally included in the “Analysis” level of Bloom’s taxonomy [ 28 – 30 ], compare-and-contrast questions encourage students to recognize, distinguish between, and relate features between scenarios and discern relevant patterns or trends, rather than compile lists of important features [ 26 ]. For example, a compare-and-contrast assessment may ask students to compare the hypotheses and research methods used in two different experimental scenarios, instead of having them evaluate the research methods of a single experiment. Alternatively, students may inherently recall and use experimental scenarios based on their prior experiences and knowledge as they evaluate an individual scenario. In addition, evaluating a single experimental scenario individually may act as metacognitive scaffolding [ 31 , 32 ]—a process which “guides students by asking questions about the task or suggesting relevant domain-independent strategies [ 32 ]—to support students in their compare-and-contrast thinking.
Purpose and research questions
Our primary objective of this study was to better understand what features of assessment questions elicit student critical thinking using two existing instruments in STEM: the Biology Lab Inventory of Critical Thinking in Ecology (Eco-BLIC) and Physics Lab Inventory of Critical Thinking (PLIC). We focused on biology and physics since critical thinking assessments were already available for these disciplines. Specifically, we investigated (a) how students critically evaluate aspects of research studies in biology and physics when they are individually evaluating one study at a time or comparing and contrasting two studies and (b) whether individual evaluation questions are needed to encourage students to engage in critical thinking when comparing and contrasting.
Providing undergraduates with ample opportunities to practice critical thinking skills in the classroom is necessary for evidence-based critical thinking in their future careers and everyday life. While most critical thinking instruments in biology and physics contexts have undergone some form of validation to ensure they are accurately measuring the intended construct, to our knowledge none have explored how different question types influence students’ critical thinking. This research offers new insight on the types of questions that elicit critical thinking, which can further be applied by educators and researchers across disciplines to measure cognitive student outcomes and incorporate more effective critical thinking opportunities in the classroom.
Ethics statement
The procedures for this study were approved by the Institutional Review Board of Cornell University (Eco-BLIC: #1904008779; PLIC: #1608006532). Informed consent was obtained by all participating students via online consent forms at the beginning of the study, and students did not receive compensation for participating in this study unless their instructor offered credit for completing the assessment.
Participants and assessment distribution
We administered the Eco-BLIC to undergraduate students across 26 courses at 11 institutions (six doctoral-granting, three Master’s-granting, and two Baccalaureate-granting) in Fall 2020 and Spring 2021 and received 1612 usable responses. Additionally, we administered the PLIC to undergraduate students across 21 courses at 11 institutions (six doctoral-granting, one Master’s-granting, three four-year colleges, and one 2-year college) in Fall 2020 and Spring 2021 and received 1839 usable responses. We recruited participants via convenience sampling by emailing instructors of primarily introductory ecology-focused courses or introductory physics courses who expressed potential interest in implementing our instrument in their course(s). Both instruments were administered online via Qualtrics and students were allowed to complete the assessments outside of class. The demographic distribution of the response data is presented in Table 1 , all of which were self-reported by students. The values presented in this table represent all responses we received.
Instrument description
Question types.
Though the content and concepts featured in the Eco-BLIC and PLIC are distinct, both instruments share a similar structure and set of question types. The Eco-BLIC—which was developed using a structure similar to that of the PLIC [ 1 ]—includes two predator-prey scenarios based on relationships between (a) smallmouth bass and mayflies and (b) great-horned owls and house mice. Within each scenario, students are presented with a field-based study and a laboratory-based study focused on a common research question about feeding behaviors of smallmouth bass or house mice, respectively. The prompts for these two Eco-BLIC scenarios are available in S1 and S2 Appendices. The PLIC focuses on two research groups conducting different experiments to test the relationship between oscillation periods of masses hanging on springs [ 1 ]; the prompts for this scenario can be found in S3 Appendix . The descriptive prompts in both the Eco-BLIC and PLIC also include a figure presenting data collected by each research group, from which students are expected to draw conclusions. The research scenarios (e.g., field-based group and lab-based group on the Eco-BLIC) are written so that each group has both strengths and weaknesses in their experimental designs.
After reading the prompt for the first experimental group (Group 1) in each instrument, students are asked to identify possible claims from Group 1’s data (data evaluation questions). Students next evaluate the strengths and weaknesses of various study features for Group 1 (individual evaluation questions). Examples of these individual evaluation questions are in Table 2 . They then suggest next steps the group should pursue (next steps items). Students are then asked to read about the prompt describing the second experimental group’s study (Group 2) and again answer questions about the possible claims, strengths and weaknesses, and next steps of Group 2’s study (data evaluation questions, individual evaluation questions, and next steps items). Once students have independently evaluated Groups 1 and 2, they answer a series of questions to compare the study approaches of Group 1 versus Group 2 (group comparison items). In this study, we focus our analysis on the individual evaluation questions and group comparison items.
The Eco-BLIC examples are derived from the owl/mouse scenario.
Instrument versions
To determine whether the individual evaluation questions impacted the assessment of students’ critical thinking, students were randomly assigned to take one of two versions of the assessment via Qualtrics branch logic: 1) a version that included the individual evaluation and group comparison items or 2) a version with only the group comparison items, with the individual evaluation questions removed. We calculated the median time it took students to answer each of these versions for both the Eco-BLIC and PLIC.
Think-aloud interviews
We also conducted one-on-one think-aloud interviews with students to elicit feedback on the assessment questions (Eco-BLIC n = 21; PLIC n = 4). Students were recruited via convenience sampling at our home institution and were primarily majoring in biology or physics. All interviews were audio-recorded and screen captured via Zoom and lasted approximately 30–60 minutes. We asked participants to discuss their reasoning for answering each question as they progressed through the instrument. We did not analyze these interviews in detail, but rather used them to extract relevant examples of critical thinking that helped to explain our quantitative findings. Multiple think-aloud interviews were conducted with students using previous versions of the PLIC [ 1 ], though these data are not discussed here.
Data analyses
Our analyses focused on (1) investigating the alignment between students’ responses to the individual evaluation questions and the group comparison items and (2) comparing student responses between the two instrument versions. If individual evaluation and group comparison items elicit critical thinking in the same way, we would expect to see the same frequency of responses for each question type, as per Fig 1 . For example, if students evaluated one study feature of Group 1 as a strength and the same study feature for Group 2 as a strength, we would expect that students would respond that both groups were highly effective for this study feature on the group comparison item (i.e., data represented by the purple circle in the top right quadrant of Fig 1 ). Alternatively, if students evaluated one study feature of Group 1 as a strength and the same study feature for Group 2 as a weakness, we would expect that students would indicate that Group 1 was more effective than Group 2 on the group comparison item (i.e., data represented by the green circle in the lower right quadrant of Fig 1 ).

The x- and y-axes represent rankings on the individual evaluation questions for Groups 1 and 2 (or field and lab groups), respectively. The colors in the legend at the top of the figure denote responses to the group comparison items. In this idealized example, all pie charts are the same size to indicate that the student answers are equally proportioned across all answer combinations.
We ran descriptive statistics to summarize student responses to questions and examine distributions and frequencies of the data on the Eco-BLIC and PLIC. We also conducted chi-square goodness-of-fit tests to analyze differences in student responses between versions within the relevant questions from the same instrument. In all of these tests, we used a Bonferroni correction to lower the chances of receiving a false positive and account for multiple comparisons. We generated figures—primarily multi-pie chart graphs and heat maps—to visualize differences between individual evaluation and group comparison items and between versions of each instrument with and without individual evaluation questions, respectively. All aforementioned data analyses and figures were conducted or generated in the R statistical computing environment (v. 4.1.1) and Microsoft Excel.
We asked students to evaluate different experimental set-ups on the Eco-BLIC and PLIC two ways. Students first evaluated the strengths and weaknesses of study features for each scenario individually (individual evaluation questions, Table 2 ) and, subsequently, answered a series of questions to compare and contrast the study approaches of both research groups side-by-side (group comparison items, Table 2 ). Through analyzing the individual evaluation questions, we found that students generally ranked experimental features (i.e., those related to study set-up, data collection and summary methods, and analysis and outcomes) of the independent research groups as strengths ( Fig 2 ), evidenced by the mean scores greater than 2 on a scale from 1 (weakness) to 4 (strength).

Each box represents the interquartile range (IQR). Lines within each box represent the median. Circles represent outliers of mean scores for each question.
Individual evaluation versus compare-and-contrast evaluation
Our results indicate that when students consider Group 1 or Group 2 individually, they mark most study features as strengths (consistent with the means in Fig 2 ), shown by the large circles in the upper right quadrant across the three experimental scenarios ( Fig 3 ). However, the proportion of colors on each pie chart shows that students select a range of responses when comparing the two groups [e.g., Group 1 being more effective (green), Group 2 being more effective (blue), both groups being effective (purple), and neither group being effective (orange)]. We infer that students were more discerning (i.e., more selective) when they were asked to compare the two groups across the various study features ( Fig 3 ). In short, students think about the groups differently if they are rating either Group 1 or Group 2 in the individual evaluation questions versus directly comparing Group 1 to Group 2.

The x- and y-axes represent students’ rankings on the individual evaluation questions for Groups 1 and 2 on each assessment, respectively, where 1 indicates weakness and 4 indicates strength. The overall size of each pie chart represents the proportion of students who responded with each pair of ratings. The colors in the pie charts denote the proportion of students’ responses who chose each option on the group comparison items. (A) Eco-BLIC bass-mayfly scenario (B) Eco-BLIC owl-mouse scenario (C) PLIC oscillation periods of masses hanging on springs scenario.
These results are further supported by student responses from the think-aloud interviews. For example, one interview participant responding to the bass-mayfly scenario of the Eco-BLIC explained that accounting for bias/error in both the field and lab groups in this scenario was a strength (i.e., 4). This participant mentioned that Group 1, who performed the experiment in the field, “[had] outliers, so they must have done pretty well,” and that Group 2, who collected organisms in the field but studied them in lab, “did a good job of accounting for bias.” However, when asked to compare between the groups, this student argued that Group 2 was more effective at accounting for bias/error, noting that “they controlled for more variables.”
Another individual who was evaluating “repeated trials for each mass” in the PLIC expressed a similar pattern. In response to ranking this feature of Group 1 as a strength, they explained: “Given their uncertainties and how small they are, [the group] seems like they’ve covered their bases pretty well.” Similarly, they evaluated this feature of Group 2 as a strength as well, simply noting: “Same as the last [group], I think it’s a strength.” However, when asked to compare between Groups 1 and 2, this individual argued that Group 1 was more effective because they conducted more trials.
Individual evaluation questions to support compare and contrast thinking
Given that students were more discerning when they directly compared two groups for both biology and physics experimental scenarios, we next sought to determine if the individual evaluation questions for Group 1 or Group 2 were necessary to elicit or helpful to support student critical thinking about the investigations. To test this, students were randomly assigned to one of two versions of the instrument. Students in one version saw individual evaluation questions about Group 1 and Group 2 and then saw group comparison items for Group 1 versus Group 2. Students in the second version only saw the group comparison items. We found that students assigned to both versions responded similarly to the group comparison questions, indicating that the individual evaluation questions did not promote additional critical thinking. We visually represent these similarities across versions with and without the individual evaluation questions in Fig 4 as heat maps.

The x-axis denotes students’ responses on the group comparison items (i.e., whether they ranked Group 1 as more effective, Group 2 as more effective, both groups as highly effective, or neither group as effective/both groups were minimally effective). The y-axis lists each of the study features that students compared between the field and lab groups. White and lighter shades of red indicate a lower percentage of student responses, while brighter red indicates a higher percentage of student responses. (A) Eco-BLIC bass-mayfly scenario. (B) Eco-BLIC owl-mouse scenario. (C) PLIC oscillation periods of masses hanging on springs scenario.
We ran chi-square goodness-of-fit tests on the answers between student responses on both instrument versions and there were no significant differences on the Eco-BLIC bass-mayfly scenario ( Fig 4A ; based on an adjusted p -value of 0.006) or owl-mouse questions ( Fig 4B ; based on an adjusted p-value of 0.004). There were only three significant differences (out of 53 items) in how students responded to questions on both versions of the PLIC ( Fig 4C ; based on an adjusted p -value of 0.0005). The items that students responded to differently ( p <0.0005) across both versions were items where the two groups were identical in their design; namely, the equipment used (i.e., stopwatches), the variables measured (i.e., time and mass), and the number of bounces of the spring per trial (i.e., five bounces). We calculated Cramer’s C (Vc; [ 33 ]), a measure commonly applied to Chi-square goodness of fit models to understand the magnitude of significant results. We found that the effect sizes for these three items were small (Vc = 0.11, Vc = 0.10, Vc = 0.06, respectively).
The trend that students answer the Group 1 versus Group 2 comparison questions similarly, regardless of whether they responded to the individual evaluation questions, is further supported by student responses from the think-aloud interviews. For example, one participant who did not see the individual evaluation questions for the owl-mouse scenario of the Eco-BLIC independently explained that sampling mice from other fields was a strength for both the lab and field groups. They explained that for the lab group, “I think that [the mice] coming from multiple nearby fields is good…I was curious if [mouse] behavior was universal.” For the field group, they reasoned, “I also noticed it was just from a single nearby field…I thought that was good for control.” However, this individual ultimately reasoned that the field group was “more effective for sampling methods…it’s better to have them from a single field because you know they were exposed to similar environments.” Thus, even without individual evaluation questions available, students can still make individual evaluations when comparing and contrasting between groups.
We also determined that removing the individual evaluation questions decreased the duration of time students needed to complete the Eco-BLIC and PLIC. On the Eco-BLIC, the median time to completion for the version with individual evaluation and group comparison questions was approximately 30 minutes, while the version with only the group comparisons had a median time to completion of 18 minutes. On the PLIC, the median time to completion for the version with individual evaluation questions and group comparison questions was approximately 17 minutes, while the version with only the group comparisons had a median time to completion of 15 minutes.
To determine how to elicit critical thinking in a streamlined manner using introductory biology and physics material, we investigated (a) how students critically evaluate aspects of experimental investigations in biology and physics when they are individually evaluating one study at a time versus comparing and contrasting two and (b) whether individual evaluation questions are needed to encourage students to engage in critical thinking when comparing and contrasting.
Students are more discerning when making comparisons
We found that students were more discerning when comparing between the two groups in the Eco-BLIC and PLIC rather than when evaluating each group individually. While students tended to independently evaluate study features of each group as strengths ( Fig 2 ), there was greater variation in their responses to which group was more effective when directly comparing between the two groups ( Fig 3 ). Literature evaluating the role of contrasting cases provides plausible explanations for our results. In that work, contrasting between two cases supports students in identifying deep features of the cases, compared with evaluating one case after the other [ 34 – 37 ]. When presented with a single example, students may deem certain study features as unimportant or irrelevant, but comparing study features side-by-side allows students to recognize the distinct features of each case [ 38 ]. We infer, therefore, that students were better able to recognize the strengths and weaknesses of the two groups in each of the assessment scenarios when evaluating the groups side by side, rather than in isolation [ 39 , 40 ]. This result is somewhat surprising, however, as students could have used their knowledge of experimental designs as a contrasting case when evaluating each group. Future work, therefore, should evaluate whether experts use their vast knowledge base of experimental studies as discerning contrasts when evaluating each group individually. This work would help determine whether our results here suggest that students do not have a sufficient experiment-base to use as contrasts or if the students just do not use their experiment-base when evaluating the individual groups. Regardless, our study suggests that critical thinking assessments should ask students to compare and contrast experimental scenarios, rather than just evaluate individual cases.
Individual evaluation questions do not influence answers to compare and contrast questions
We found that individual evaluation questions were unnecessary for eliciting or supporting students’ critical thinking on the two assessments. Students responded to the group comparison items similarly whether or not they had received the individual evaluation questions. The exception to this pattern was that students responded differently to three group comparison items on the PLIC when individual evaluation questions were provided. These three questions constituted a small portion of the PLIC and showed a small effect size. Furthermore, removing the individual evaluation questions decreased the median time for students to complete the Eco-BLIC and PLIC. It is plausible that spending more time thinking about the experimental methods while responding to the individual evaluation questions would then prepare students to be better discerners on the group comparison questions. However, the overall trend is that individual evaluation questions do not have a strong impact on how students evaluate experimental scenarios, nor do they set students up to be better critical thinkers later. This finding aligns with prior research suggesting that students tend to disregard details when they evaluate a single case, rather than comparing and contrasting multiple cases [ 38 ], further supporting our findings about the effectiveness of the group comparison questions.
Practical implications
Individual evaluation questions were not effective for students to engage in critical thinking nor to prepare them for subsequent questions that elicit their critical thinking. Thus, researchers and instructors could make critical thinking assessments more effective and less time-consuming by encouraging comparisons between cases. Additionally, the study raises a question about whether instruction should incorporate more experimental case studies throughout their courses and assessments so that students have a richer experiment-base to use as contrasts when evaluating individual experimental scenarios. To help students discern information about experimental design, we suggest that instructors consider providing them with multiple experimental studies (i.e., cases) and asking them to compare and contrast between these studies.
Future directions and limitations
When designing critical thinking assessments, questions should ask students to make meaningful comparisons that require them to consider the important features of the scenarios. One challenge of relying on compare-and-contrast questions in the Eco-BLIC and PLIC to elicit students’ critical thinking is ensuring that students are comparing similar yet distinct study features across experimental scenarios, and that these comparisons are meaningful [ 38 ]. For example, though sample size is different between experimental scenarios in our instruments, it is a significant feature that has implications for other aspects of the research like statistical analyses and behaviors of the animals. Therefore, one limitation of our study could be that we exclusively focused on experimental method evaluation questions (i.e., what to trust), and we are unsure if the same principles hold for other dimensions of critical thinking (i.e., what to do). Future research should explore whether questions that are not in a compare-and-contrast format also effectively elicit critical thinking, and if so, to what degree.
As our question schema in the Eco-BLIC and PLIC were designed for introductory biology and physics content, it is unknown how effective this question schema would be for upper-division biology and physics undergraduates who we would expect to have more content knowledge and prior experiences for making comparisons in their respective disciplines [ 18 , 41 ]. For example, are compare-and-contrast questions still needed to elicit critical thinking among upper-division students, or would critical thinking in this population be more effectively assessed by incorporating more sophisticated data analyses in the research scenarios? Also, if students with more expert-like thinking have a richer set of experimental scenarios to inherently use as contrasts when comparing, we might expect their responses on the individual evaluation questions and group comparisons to better align. To further examine how accessible and context-specific the Eco-BLIC and PLIC are, novel scenarios could be developed that incorporate topics and concepts more commonly addressed in upper-division courses. Additionally, if instructors offer students more experience comparing and contrasting experimental scenarios in the classroom, would students be more discerning on the individual evaluation questions?
While a single consensus definition of critical thinking does not currently exist [ 15 ], continuing to explore critical thinking in other STEM disciplines beyond biology and physics may offer more insight into the context-specific nature of critical thinking [ 22 , 23 ]. Future studies should investigate critical thinking patterns in other STEM disciplines (e.g., mathematics, engineering, chemistry) through designing assessments that encourage students to evaluate aspects of at least two experimental studies. As undergraduates are often enrolled in multiple courses simultaneously and thus have domain-specific knowledge in STEM, would we observe similar patterns in critical thinking across additional STEM disciplines?
Lastly, we want to emphasize that we cannot infer every aspect of critical thinking from students’ responses on the Eco-BLIC and PLIC. However, we suggest that student responses on the think-aloud interviews provide additional qualitative insight into how and why students were making comparisons in each scenario and their overall critical thinking processes.

Conclusions
Overall, we found that comparing and contrasting two different experiments is an effective and efficient way to elicit context-specific critical thinking in introductory biology and physics undergraduates using the Eco-BLIC and the PLIC. Students are more discerning (i.e., critical) and engage more deeply with the scenarios when making comparisons between two groups. Further, students do not evaluate features of experimental studies differently when individual evaluation questions are provided or removed. These novel findings hold true across both introductory biology and physics, based on student responses on the Eco-BLIC and PLIC, respectively—though there is much more to explore regarding critical thinking processes of students across other STEM disciplines and in more advanced stages of their education. Undergraduate students in STEM need to be able to critically think for career advancement, and the Eco-BLIC and PLIC are two means of measuring students’ critical thinking in biology and physics experimental contexts via comparing and contrasting. This research offers new insight on the types of questions that elicit critical thinking, which can further be applied by educators and researchers across disciplines to teach and measure cognitive student outcomes. Specifically, we recommend instructors incorporate more compare-and-contrast questions related to experimental design in their courses to efficiently elicit undergraduates’ critical thinking.
Supporting information
S1 appendix, s2 appendix, s3 appendix, acknowledgments.
We thank the members of the Cornell Discipline-based Education Research group for their feedback on this article, as well as our advisory board (Jenny Knight, Meghan Duffy, Luanna Prevost, and James Hewlett) and the AAALab for their ideas and suggestions. We also greatly appreciate the instructors who shared the Eco-BLIC and PLIC in their classes and the students who participated in this study.
Funding Statement
This work was supported by the National Science Foundation under grants DUE-1909602 (MS & NH) and DUE-1611482 (NH). NSF: nsf.gov The funders had no role in study design, data collection and analysis, decision to publish, or preparation of the manuscript.
Data Availability
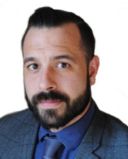
Open-Mindedness and Skepticism in Critical Thinking
How the two traits work together..
Posted April 26, 2019
In my most recent post, 12 Important Dispositions for Critical Thinking, I presented a list of dispositions that are likely to enhance the quality of one’s thinking—specifically, disposition toward critical thinking refers to an inclination, tendency, or willingness to perform a given thinking skill (Dwyer, 2017; Facione, Facione & Giancarlo, 1997; Ku, 2009; Norris, 1992; Siegel, 1999; Valenzuela, Nieto & Saiz, 2011). Though there is overlap among some of the dispositions (e.g., inquisitiveness, truth-seeking, and resourcefulness), there are, of course, important distinctions. However, in one particular case—open-mindedness and scepticism—it almost seems that the dispositions are at odds with one another.
I received feedback on the piece, and one reader recommended that, though they agree that it's good to have an open mind, some viewpoints are simply foolish, and it would be a waste of time to dwell on them. I responded with agreement, to some extent. However, even if an idea appears foolish, sometimes its consideration can lead to an intelligent, critically considered conclusion. In this way, open-mindedness follows the same mechanics as ‘brainstorming’ ideas, in that no idea is a bad one because the ‘bad ones’ sometimes provide a foundation for a ‘good one.’ I advised, furthermore, that there are important subtleties that require consideration with respect to understanding the relationship between scepticism and open-mindedness.
To better understand this relationship, it is important to first operationally define the two dispositions. Open-mindedness refers to an inclination to be cognitively flexible and avoid rigidity in thinking; to tolerate divergent or conflicting views and treat all viewpoints alike, prior to subsequent analysis and evaluation; to detach from one’s own beliefs and consider, seriously, points of view other to one’s own without bias or self-interest; to be open to feedback by accepting positive feedback and to not reject criticism or constructive feedback without thoughtful consideration; to amend existing knowledge in light of new ideas and experiences; and to explore such new, alternative or ‘unusual’ ideas. On the other hand, seemingly, the disposition towards scepticism refers to an inclination to challenge ideas; to withhold judgment before engaging all the evidence or when the evidence and reasons are insufficient; to take a position and be able to change position when the evidence and reasons are sufficient; and to look at findings from various perspectives.
Though on a foundational level, the two dispositions may seem to reside on a kind of continuum (e.g., scepticism at one end and open-mindedness at the other end), they are distinct concepts, even if there is overlap. That is, an individual can be both sceptical and open-minded at the same time. Perhaps the key issue here is to recognise that open-mindedness doesn’t mean you have to accept divergent ideas, rather just consider them.
Even with that, isn’t consideration of a foolish idea still a ‘waste of time?' Well, the decision-making behind determining whether or not something is foolish is still consideration—some level of evaluation, no matter how easy, was required to make the decision. That’s where the scepticism comes in: rejection of the ‘foolish’ idea is the outcome of appropriate evaluation. However, knowing that the idea is foolish isn’t necessarily the end of the story. You may ask yourself whether anything can be salvaged from the bad idea or the thought process behind it, for the purpose of turning it into a good one; thus, being open-minded through idea generation, such as in the aforementioned example of the mechanics behind brainstorming. But with that, there’s more to open-mindedness than that.
Open-mindedness is about being open to changing your mind in light of new evidence. It’s about detaching from your beliefs and focusing on unbiased thinking void of self-interest. It’s about being open to constructive criticism and new ideas. People who are sceptical do all of this as well—they challenge ideas and they withhold judgment until sufficient evidence is provided—they are open to all possibilities until sufficient evidence is presented. Scepticism and open-mindedness go hand-in-hand, but they may not seem that way from the surface—not until they are adequately and comprehensively defined. Once described accordingly, it is hard not to equate both with critical thinking. Well, I’d be sceptical of it, anyway.
Dwyer, C.P. (2017). Critical thinking: Conceptual perspectives and practical guidelines. UK: Cambridge University Press.
Facione, P. A., Facione, N. C., & Giancarlo, C. A. (1997). Setting expectations for student learning: New directions for higher education. Millbrae: California Academic Press.
Ku, K. Y. L. (2009). Assessing students’ critical thinking performance: Urging for measurements using multi-response format. Thinking Skills and Creativity, 4(1), 70–76.
Norris, S. P. (Ed.). (1992). The generalizability of critical thinking: Multiple perspectives on an educational ideal. New York: Teachers College Press.
Siegel, H. (1999). What (good) are thinking dispositions? Educational Theory, 49(2), 207–221.
Valenzuela, J., Nieto, A. M., & Saiz, C. (2011). Critical thinking motivational scale: A contribution to the study of relationship between critical thinking and motivation. Journal of Research in Educational Psychology, 9(2), 823–848.
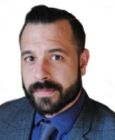
Christopher Dwyer, Ph.D., is a lecturer at the Technological University of the Shannon in Athlone, Ireland.
- Find a Therapist
- Find a Treatment Center
- Find a Psychiatrist
- Find a Support Group
- Find Teletherapy
- United States
- Brooklyn, NY
- Chicago, IL
- Houston, TX
- Los Angeles, CA
- New York, NY
- Portland, OR
- San Diego, CA
- San Francisco, CA
- Seattle, WA
- Washington, DC
- Asperger's
- Bipolar Disorder
- Chronic Pain
- Eating Disorders
- Passive Aggression
- Personality
- Goal Setting
- Positive Psychology
- Stopping Smoking
- Low Sexual Desire
- Relationships
- Child Development
- Therapy Center NEW
- Diagnosis Dictionary
- Types of Therapy
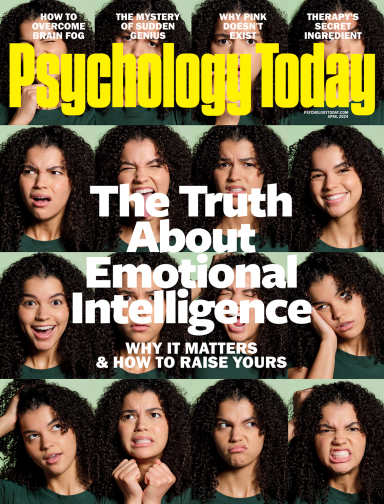
Understanding what emotional intelligence looks like and the steps needed to improve it could light a path to a more emotionally adept world.
- Coronavirus Disease 2019
- Affective Forecasting
- Neuroscience
What is Skepticism
The word “skepticism” comes from the ancient Greek skepsis, meaning “inquiry.” Skepticism is, therefore, not a cynical rejection of new ideas, as the popular stereotype goes, but rather an attitude of both open mind and critical sense.
The ancient skeptics simply doubted that human beings can achieve certain knowledge, and preferred to be agnostic about a number of notions which they felt we just did not grasp securely.
That philosophical tradition eventually informed the beginnings of science in the 17th and 18th centuries, and it is best captured by David Hume’s advice that wise persons proportion their beliefs to the evidence. Or, as Carl Sagan put it much later, extraordinary claims require extraordinary evidence.
The modern skeptical movement is a grassroots phenomenon that aims at helping the public navigate the complex borderlands between sense and nonsense, science and pseudoscience.
Skepticism does so by way of investigation of alleged extraordinary phenomena, mindful cultivation of critical thinking, and an honest attitude toward intellectual inquiry.
The Burden of Skepticism
Following is an excerpt from the Skeptical Inquirer archives ( Vol. 12 Issue No.1 ) where Carl Sagan describes skepticism in his own words.
What Is Skepticism? It’s nothing very esoteric. We encounter it every day. When we buy a used car, if we are the least bit wise we will exert some residual skeptical powers—whatever our education has left to us. You could say, “Here’s an honest-looking fellow. I’ll just take whatever he offers me.” Or you might say, “Well, I’ve heard that occasionally there are small deceptions involved in the sale of a used car, perhaps inadvertent on the part of the salesperson,” and then you do something. You kick the tires, you open the doors, you look under the hood. (You might go through the motions even if you don’t know what is supposed to be under the hood, or you might bring a mechanically inclined friend.) You know that some skepticism is required, and you understand why. It’s upsetting that you might have to disagree with the used-car salesman or ask him questions that he is reluctant to answer. There is at least a small degree of interpersonal confrontation involved in the purchase of a used car and nobody claims it is especially pleasant. But there is a good reason for it—because if you don’t exercise some minimal skepticism, if you have an absolutely untrammeled credulity, there is probably some price you will have to pay later. Then you’ll wish you had made a small investment of skepticism early. Now this is not something that you have to go through four years of graduate school to understand. Everybody understands this. The trouble is, a used car is one thing but television commercials or pronouncements by presidents and party leaders are another. We are skeptical in some areas but unfortunately not in others.
Skeptics and Science Deniers
Public discussion of scientific topics such as global warming is confused by misuse of the term “skeptic.” A Nov. 10, 2014, New York Times article incorrectly referred to Sen. James Inhofe as “a prominent skeptic of climate change.” Two days later Scott Horsley of NPR’s Morning Edition called him “one of the leading climate change deniers in Congress.” These are not equivalent statements.
There is a concern that “skeptic” and “denier” have been conflated. Proper skepticism promotes scientific inquiry, critical investigation, and the use of reason in examining controversial and extraordinary claims.
It is foundational to the scientific method. Denial, on the other hand, is the a priori rejection of ideas without objective consideration.
As scientific skeptics, we are well aware of political efforts to undermine climate science by those who deny reality but do not engage in scientific research or consider evidence that their deeply held opinions are wrong.
The most appropriate word to describe the behavior of those individuals is “denial.” Not all individuals who call themselves climate change skeptics are deniers.
Skeptics are those who have devoted much of their careers to practicing and promoting scientific skepticism.
CFI and Skepticism
CFI is committed to the pursuit of scientific skepticism as part of our core mission.
Through our TIES Program , CFI gives middle school teachers the tools they need to effectively teach evolution to children. Our vision is to build a generation of thinkers that approach natural world through a scientific lens.
If you found this definition helpful you can help the Center for Inquiry by sharing on Facebook or Twitter

Much of epistemology has arisen either in defense of or in opposition to various forms of skepticism. Indeed, one could classify various theories of knowledge by their responses to skepticism. For example, rationalists could be viewed as skeptical about the possibility of empirical knowledge while not being skeptical with regard to a priori knowledge and empiricists could be seen as skeptical about the possibility of a priori knowledge but not so with regard to empirical knowledge. In addition, many traditional problems, for example the problem of other minds or the problem of our knowledge of God's existence, can be seen as restricted forms of skepticism which hold that we cannot have knowledge of any propositions in some particular domain thought to be within our ken. This essay will focus on the general forms of skepticism which question our knowledge in many, if not all, domains in which we ordinarily think knowledge is possible. Since this essay is not primarily devoted to a discussion of the history of philosophical skepticism, the general forms of skepticism to be discussed are those which contemporary philosophers still find the most interesting.
1. Philosophical Skepticism vs. Ordinary Incredulity
2. two basic forms of philosophical skepticism, 3. academic skepticism, 4. the argument for academic skepticism employing the closure principle.
- 5. The Cartesian-style Argument for Academic Skepticism Employing the Eliminate All Doubts Principle
- 6. Contextualism
7. Pyrrhonism
8. the mode to respond to the foundationalist, 9. the mode to respond to the coherentist, 10. the mode to respond to the infinitist, 11. the overall effect of the modes, bibliography, other internet resources, related entries.
Even before examining the various general forms of skepticism, it is crucial that we distinguish between philosophical skepticism and ordinary incredulity because doing so will help to explain why philosophical skepticism is so intriguing. Consider an ordinary case in which we think someone fails to have knowledge. Suppose Anne claims that she knows that the bird she is looking at is a robin and that I believe that if Anne were to look carefully, she would see that its coloration is not quite that of a robin. Its breast is too orange. Further, I believe that it flies somewhat differently than robins do. This bird seems to flitter more than a typical robin.
Thus, there are two grounds for doubting that Anne knows that this is a robin:
- The color of this bird isn't typical of robins.
- The flight pattern of this bird is not typical of robins.
Now, what makes this a case of ordinary doubt is that there are, in principle, two ways of removing the basis for doubt:
- Anne could show that the alleged grounds for doubt are false; or
- Anne could show that the grounds for doubt, though true, can be neutralized. [ 1 ]
Taking alternative (1), Anne could show that there are many robins with the coloration of the bird in question by citing the Audubon Field Guide for Birds in which many of the pictured robins have very orange breasts. In other words, Anne could show that (a) is false.
But in order to remove grounds for doubt, it is not necessary that Anne show that the alleged grounds are false. Alternative (2) is available. Consider ground (b). It could be granted that the bird in question flies in a way that is not at all typical of robins. But suppose that on closer inspection we see that some of its tail feathers have been damaged in a way that could account for the unusual flight pattern. Because the bird has difficulty gliding and flying in a straight line, it flaps its wings much more rapidly than is typical of robins. Thus, although we can grant that (b) is true, we would have explained away, or neutralized, the grounds for doubt.
The point here is that in this case, and in all ordinary cases of incredulity, the grounds for the doubt can, in principle, be removed. As Wittgenstein would say, doubt occurs within the context of things undoubted. If something is doubted, something else must be held fast because doubt presupposes that there are means of removing the doubt. [ 2 ] We doubt that the bird is a robin because, at least in part, we think we know how robins typically fly and what their typical coloration is. That is, we think our general picture of the world is right — or right enough — so that it does provide us with both the grounds for doubt and the means for potentially removing the doubt. Thus, ordinary incredulity, say about some feature of the world, occurs against a background of sequestered beliefs about the world. We are not doubting that we have any knowledge of the world. Far from it, we are presupposing that we do know some things about the world. To quote Wittgenstein, “A doubt without an end is not even a doubt” (Wittgenstein 1969, ¶ 625).
In contrast, philosophical skepticism attempts to render doubtful every member of a class of propositions that we think falls within our ken. One member of the class is not pitted against another. The grounds for either withholding assent to the claim that we can have such knowledge or denying that we can have such knowledge are such that there is no possible way to either answer them or neutralize them by appealing to another member of the class. Thus, philosophic doubt or philosophical skepticism, as opposed to ordinary incredulity, does not, in principle, come to an end. Or so the philosophic skeptic will claim!
To clarify the distinction between ordinary incredulity and philosophical doubt, let us consider two recent movies: “The Truman Show” and “The Matrix.” In the former, a character is placed, without his knowledge, in a contrived environment so that his “life” can be broadcast on television. But he begins to wonder whether the world surrounding him is, in fact, what it appears to be. Some events seem to happen too regularly and many other things are just not quite as they should be. Eventually, Truman obtains convincing evidence that all his world is a stage and all the men and women are merely players. The crucial point is that even had he not developed any doubts, there is, in principle, a way to resolve them had they arisen. Such doubts, though quite general, are examples of ordinary incredulity.
Contrast this with the deception practiced in The Matrix. When everything is running as programmed by the machines, there is no possible way for the “people” in the matrix to determine that the world as experienced is only a “dream world” and not the real world (the world of causes and effects). The only “reality” that it is possible to investigate is a computer generated one. (See Irwin 2002, 2005 for collections of articles on The Matrix.)
The Truman Story is a depiction of a case of ordinary incredulity because there is some evidence available for determining what's really the case; whereas The Matrix depicts a situation similar to that imagined by the philosophic skeptic in which it is not possible to obtain evidence for determining that things are not as they seem (at least when the virtual reality is perfectly created). Put another way, the philosophic skeptic challenges our ordinary assumption that there is evidence available that can help us to discriminate between the real world and some counterfeit world that appears in all ways to be identical to the real world. Ordinary incredulity arises within the context of other propositions of a similar sort taken to be known, and it can be removed by discovering the truth of some further proposition of the relevant type. On the other hand, philosophical skepticism about a proposition of a certain type derives from considerations that are such that they cannot be removed by appealing to additional propositions of that type — or so the skeptic claims.
These movies illustrate one other fundamental feature of the philosophical arguments for skepticism, namely, that the debate between the skeptics and their opponents takes place within the evidentialist account of knowledge which holds that knowledge is at least true, sufficiently justified belief. The debate is over whether the grounds are such that they can make a belief sufficiently justified so that a responsible epistemic agent is entitled to assent to the proposition. [ 3 ] The basic issue at stake is whether the justification condition can be fulfilled. A corollary of this is that strictly reliabilist or externalist responses to philosophic skepticism constitute a change of subject. A belief could be reliably produced, i.e., its causal pedigree could be such that anything having that causal etiology is sufficiently likely to be true, but the reasons available for it could fail to satisfy the standards agreed upon by both the skeptics and their opponents.
Consider some proposition, p . There are just three possible propositional attitudes one can have with regard to p 's truth when considering whether p is true. One can either assent to p , or assent to ~ p or withhold assenting both to p and to ~ p . Of course, there are other attitudes one could have toward p . One could just be uninterested that p or be excited or depressed that p . But those attitudes are either ones we have when we are not considering whether p is true or they are attitudes that result from our believing, denying or withholding p . For example, I might be happy or sorry that p is true when I come to believe that it is.
I just spoke of “assent” and I mean to be using it to depict the pro-attitude, whatever it is, toward a proposition that is required for knowing that proposition. Philosophers have differed about what that attitude is. Some take it to be something akin to being certain that p or guaranteeing that p (Malcolm 1963, 58-72). Others have taken it not to be a form of belief at all because, for example, one can know that p without believing it as in cases in which I might in fact remember that Queen Victoria died in 1901 but not believe that I remember it and hence might be said not to believe it (Radford 1966). For the purposes of this essay we need not attempt to pin down precisely the nature of the pro-attitude toward p that is necessary for knowing that p . It is sufficient for our purposes to stipulate that assent is the pro-attitude toward p required to know that p .
Let us use “EI-type” propositions to refer to epistemically interesting types of propositions. Such types of propositions contain tokens some of which are generally thought to be known given what we ordinarily take knowledge to be. Thus, it would not be epistemically interesting if we did not know exactly what the rainfall will be on March 3 ten years from now. That kind of thing (a fine grained distant future state) is not generally thought to be known given what we ordinarily take knowledge to be. But it would be epistemically interesting if we cannot know anything about the future, or anything about the contents of someone else's mind, or anything about the past, or anything at all about the “external world.” We think we know many propositions about those types of things.
Now, consider the (meta) proposition concerning the scope of our knowledge, namely: We can have knowledge of EI-type propositions. Given that there are just three stances we can have toward any proposition when considering whether to assent to it, we can:
- Assent that we can have knowledge of EI-type propositions.
- Assent that we cannot have knowledge of EI-type propositions.
- Withhold assent to both that we can and that we cannot have knowledge of EI-type propositions.
Let us call someone with the attitude depicted in (i) an “Epistemist.” [ 4 ] Such a person assents to the claim that we can have knowledge of EI-type propositions.
The attitude portrayed in (ii) has gone under many names. I will follow the terminology suggested by Sextus Empiricus. He used the term “Academics” to refer to the leaders of the Academy (founded by Plato) during the 3rd to 1st century B.C. According to Sextus, they assented to the claim that we cannot have knowledge of what I have called EI-type propositions — although it is far from clear that this was an accurate description of their views. (See the entry on ancient skepticism .) Perhaps the prime example was Carneades (214-129 B.C.). Other philosophers will refer to this view as “Cartesian skepticism” because of the skeptical arguments investigated by Descartes and his critics in the mid-17th century. And still others will refer to it as “switched world skepticism” or “possible world skepticism” because the arguments for it typically involve imagining oneself to be in some possible world that is both vastly different from the actual world and at the same time absolutely indistinguishable (at least by us) from the actual world. What underlies this form of skepticism is assent to the proposition that we cannot know EI-type propositions because our evidence is inadequate.
Those assenting neither to the proposition that knowledge of EI-type propositions is possible nor to the proposition that such knowledge is not possible can be called “Pyrrhonian Skeptics” after Pyrrho who lived between ca 365 - ca 275 B.C. The primary source of Pyrrhonian Skepticism is the writing of Sextus Empiricus who lived at the end of the second century AD. The Pyrrhonians withheld assent to every non-evident proposition. That is, they withheld assent to all propositions about which genuine dispute was possible, and they took that class of propositions to include the (meta) proposition that we can have knowledge of EI-type propositions. Indeed, they sometimes classified the Epistemists and the Academic Skeptics together as dogmatists because the Epistemists assented to the proposition that we can have knowledge, while the Academic Skeptics assented to the denial of that claim. [ 5 ]
Another difference between Academic and Pyrrhonian Skepticism is closely related to the charge by the latter that the former is really a disguised type of dogmatism. The Academic Skeptic thinks that her view can be shown to be the correct one by an argument (or by arguments). The Pyrrhonian would point out that the Academic Skeptic maintains confidence in the ability of reason to settle matters — at least with regard to the extent of our knowledge of propositions in the EI-class. One way of understanding the so-called problem of the “Cartesian circle” illustrates the Pyrrhonian point: Descartes is relying throughout the Meditations on his power of reasoning to remove the skeptical doubts that he raises, but to do so means that he has exempted the faculty of reasoning from the doubts that he raised in the “First Meditation” about the epistemic reliability of our faculties. A Cartesian reply could be as simple as paraphrasing Luther: Here I stand, as a philosopher with confidence in reason, and as such I can do no other. [ 6 ] Regardless of the adequacy of that kind of response, the point here is that the Pyrrhonians did not think that they had a convincing argument whose conclusion was that withholding assent to non-evident propositions was the appropriate epistemic attitude to have.
I think it is fair to say that Academic Skepticism is usually what is meant when most contemporary philosophers write about skepticism. Thus, it is that form of skepticism to which we will now turn and it is that form that will be the primary focus of this essay, although I will discuss some aspects of Pyrrhonism later.
A way to motivate Academic Skepticism and to clearly distinguish it from ordinary incredulity is to trace the way in which Descartes expanded the realm of what was doubtful (and hence not worthy of assent) in the “First Meditation.” [ 7 ] Descartes begins by noting that the senses have deceived him on some occasions and, in the voice of his skeptical interlocutor, he conjectures that it is never prudent to trust what occasionally misleads. So, we don't have “certain” knowledge of the external world based upon the testimony of our senses. However, in the voice of the non-skeptical interlocutor, he replies that even though the senses have misled him, he can neutralize that purported basis for doubt by pointing out that we are able to determine when our senses are not trustworthy. Thus, this is a case of ordinary incredulity because he appeals to some knowledge of the world gained through our senses to neutralize this basis for doubt. For example, in looking at a straight stick in water, even though it appears bent, we know not to accept the testimony of our senses at face value. We can neutralize the potentially knowledge-robbing proposition that my senses have deceived me on some occasions by adding to it another proposition to which we assent, namely, I can distinguish between the occasions when my senses are trustworthy and those when they are not. Some propositions in the EI-type (propositions about the external world) can be used to rebuff the grounds for ordinary incredulity. Thus, no basis for (philosophical) Academic Skepticism has been located.
Descartes next considers dreaming. What if he were dreaming at that very moment? Would he still have some knowledge of the external world? Yes; because in dreams and in waking life there are some common general features. So, if he were dreaming, he would not know in particular what is going on about him at that moment, but that does not imply that he fails to have any knowledge at that moment. For example, he still could know that there are hands. More importantly, even more simple things about nature “in general” are not thereby made doubtful. We have not found any reason for doubting that there are material objects or that they have a spatial location, or are in motion or at rest, or can exist for a long or short period of time. Again, no basis for Academic Skepticism has been established.
But then Descartes thinks of a grounds for doubt for which he says he “certainly has no reply.” He puts it this way:
… In whatever way [it is supposed] that I have arrived at the state of being that I have reached — whether [it is attributed] to fate or to accident, or [made] out that it is by a continual succession of antecedents, or by some other method — since to err and deceive oneself is a defect, it is clear that the greater will be the probability of my being so imperfect as to deceive myself ever, as is the Author of my being to whom [is assigned] my origin the less powerful. ( Meditations , 147)
In other words, at this point in the Meditations , since he lacks an argument for the claim that whatever is causally responsible for his “state of being” is capable of making his being such that to err would not be natural for it, assenting to propositions arrived at by his “state of being” is not legitimate. Thus, Descartes believes that he has located a basis for doubting all of his supposed former knowledge of the external world that cannot be repulsed by locating another such proposition to which he is entitled. He has found a proposition that, if true, would (by itself) defeat the justification he has for his assenting to propositions about the external world and which is such that (1) he does not (at least at this point in the Meditations ) have a way to reject it and such that (2) he has no way to neutralize its effect. Thus, a basis for philosophical skepticism has been found because an entire class of EI-type propositions — propositions that his “nature” has led him to assent to — is now thrown into doubt because he cannot use one member of the class to reject or neutralize the basis for doubting another member of the class.
Descartes apparently thinks that something is worthy of assent only if it is immune to genuine doubt. It appears that he thinks that something, d , is a grounds for genuine doubt of p for S iff:
- d added to S 's beliefs makes assent to p no longer adequately justified;
- S is not justified in denying d ; [ 8 ]
- S has no way to neutralize d . [ 9 ]
The final step is to say that some proposition is not worthy of assent if there are genuine grounds for doubting it. Indeed, Descartes grants that even after d is located, p might still be more reasonable to believe than to deny ( Meditations , 148). The point is that the pro-attitude should not rise to the level required for knowledge because there is a genuine ground for doubt. Thus, a crucial feature of the Cartesian-style argument for Academic Skepticism is that it employs a very stringent requirement on the type of evidence required for knowledge. It must make a proposition immune to genuine doubt.
To make that clear, let us state the epistemic principle, which we can call the “Eliminate All Doubt Principle,” that apparently informs the Cartesian-style argument:
Eliminate All Doubt Principle [ EAD ]: For all propositions x and d , if d satisfies condition 1. in the definition of genuine doubt that x , then if assenting to x is adequately justified for S , then S is adequately justified in eliminating d (either by denying or neutralizing it).
In more contemporary terminology, the ground for doubt proposed by Descartes can be put like this:
U : My epistemic equipment is untrustworthy.
The Cartesian-style argument for Academic Skepticism can now be put like this:
- If I know that p , then there are no genuine grounds for doubting that p .
- U is a genuine ground for doubting that p .
- Therefore, I do not know that p .
The Cartesian-style argument does not readily lend itself to the objection that by employing it the skeptic is contradicting herself on the grounds that the argument purportedly shows that she fails to know because her epistemic equipment is untrustworthy while at the same time she is employing the very equipment that, were the argument sound, would be unreliable. The reason is that she is neither asserting that her equipment is untrustworthy nor claiming that there is an argument which shows that her equipment is untrustworthy. She is merely claiming that U is a genuine ground for doubt. Thus she neither is holding contradictory beliefs nor is her practice somehow incompatible with her beliefs.
The Cartesian-style argument for Academic Skepticism should be contrasted with what many contemporary philosophers take to be the canonical argument for Academic Skepticism which employs the Closure Principle (CP). [ 10 ] Letting “ h ” stand for an EI-type proposition, for example, G. E. Moore's famous “here's a hand” and letting “ sk ” stand for “I am in a switched-world in which there are no hands, but it appears just as though there were hands,” we can state the canonical CP-style argument for Academic Skepticism as follows:
CP1. If I am justified in believing that h , then I am justified in believing that ~ sk . CP2. I am not justified in believing that ~ sk . Therefore, I am not justified in believing that h .
This argument appeals to a form of the Closure Principle in Premise 1. Letting “ Jsx ” stand for “ S is justified in having some pro-attitude, J , regarding x ,” that principle can be stated as:
Closure Principle [ CP ]: For all propositions x and y , if x entails y , and Jsx , then Jsy .
(In the CP-style argument: x = h and y = ~ sk .)
A crucial feature of CP is that it does not depend upon employing a stringent notion of justification. Suppose that (positive) justification comes in degrees, where the lowest degree is something like mere plausibility and the highest degree is absolute certainty. CP could be recast as follows:
CP*: For all propositions, x and y , if x entails y , and Jsx to degree u , then Jsy to degree v (where u ≤ v ).
Thus, when the Academic Skeptic employs CP (or CP*), she need not be employing a very stringent notion of justification. That is a primary difference between the CP-style and the Cartesian-style argument for Academic Skepticism.
Another difference is that the Cartesian-style argument concerns knowledge, whereas the CP-style argument concerns justification (to whatever degree). Nevertheless, that difference is insignificant because the debate about the merits of skepticism takes place within the evidentialist account of knowledge. Knowledge is taken to entail adequately justified assent and, hence, “knowledge” could be replaced by “adequately justified assent” in the Cartesian style argument.
Let us return to the central difference between Cartesian and CP-style arguments, namely the former employs EAD while the later employs CP (or CP*). EAD requires that we eliminate any genuine grounds for doubt and those include more than mere contraries (propositions which are such that they both cannot be true, but they both could be false). In addition, recall that according to the Cartesian to be adequately justified in eliminating d as a ground for doubt for x , either S is adequately justified in denying d (assenting to ~ d ) or S is adequately justified in assenting to some neutralizing proposition, n , such that adding ( n & d ) to S 's beliefs fails to make it the case that x is no longer adequately justified. [ 11 ] Thus, since every contrary of some proposition is a potential genuine ground for doubt in virtue of satisfying condition 1. in the definition of genuine doubt, EAD entails CP but CP does not entail EAD. [ 12 ] To see that, consider any contrary, say c , of a proposition, say h . The proposition, c , would be a potential genuine ground for doubting h since if c were added to S 's beliefs, h would no longer be adequately justified because S 's beliefs would then contain a proposition, c , that entailed the denial of h . Furthermore, the only way S could eliminate c as a ground for doubt would be by denying it, since nothing could neutralize it. Thus, EAD has the consequence that if S is justified in assenting to h , then S is justified in denying every contrary of h . But that is just an instance of CP, since (by hypothesis) h entails ~ c . That CP does not entail EAD should be clear since there are grounds for doubting h that are not contraries of h . For example, the proposition, U , considered above is a grounds for doubting h , but h and U could both be true.
Thus, there are two basic forms of Academic Skepticism: The Cartesian-style argument that employs the strong EAD principle and the CP-style that employs the weaker CP. Since the CP-style skeptic employs the weaker epistemic principle, it will be best to begin by focusing on it because any criticisms of it are likely to redound to the stronger form.
There appear to be only three ways that one can respond to the CP-style skeptical argument: deny at least one premise, deny that the argument is valid, or reluctantly accept the conclusion — if neither of the first two alternatives succeeds. (I say “appear” because I will mention later a fourth alternative that is available to the Pyrrhonian Skeptic.) The second alternative — denying the validity of the argument — has not been taken seriously by the anti-skeptic because it would lead to embracing an extremely severe form of skepticism. If one were to deny that modus tollens is a valid form of inference, one would also have to deny the validity of (i) disjunctive syllogism or (ii) modus ponens and contraposition, since it is easy to transform modus tollens arguments into ones employing the other forms of inference. Hence, if this alternative were chosen, reasoning would apparently come to a complete standstill. That, presumably, is why no one has ever seriously considered this alternative.
So, if we are not to reluctantly embrace the conclusion, it appears as though we must reject either the first premise — an instantiation of closure — or the second premise.
Consideration of CP1
Let us begin an examination of CP1 and the general closure principle of which it is an instantiation. The basic issue is this: Does closure hold for justified belief?
Closure certainly does hold for some properties, for example, truth. If p is true and it strictly implies q , then q is true. It just as clearly does not hold for other properties. If p is a belief of mine, and p strictly implies q , it does not follow that q is a belief of mine. I might fail to see the implication or I might be “wired” incorrectly (from birth or as the result of an injury) or I simply might be epistemically perverse. I might, for example, believe all of the axioms of Euclidean plane geometry, but fail to believe (or perhaps even refuse to believe) that the exterior angle of a triangle is equivalent to the sum of the two opposite interior angles.
What about justified belief? It is easy to see that, as stated above, CP (or CP*) is clearly false. Every necessary truth is entailed by every proposition, and we can be justified in believing a false proposition. But one surely does not want to claim that S is justified in believing every necessary truth whenever S has some justified belief in a false proposition. In addition, some entailments might be beyond S 's capacity to grasp. Finally, there might even be some contingent propositions that are beyond S's capacity to grasp which are entailed by some propositions that S does, indeed, grasp. And it might be thought that S is not entitled to believe anything that S cannot grasp.
But it also appears that CP can easily be repaired. We can stipulate (i) that the domain of the propositions in the generalization of CP includes only contingent propositions that are within S 's capacity to grasp and (ii) that the entailment is “obvious” to S . The skeptic can agree to those restrictions because the skeptical scenarios are posited in such a way as to render it obvious that our ordinary beliefs are false in those scenarios, and it is taken to be a contingent claim that S is in the actual circumstances as described in the antecedent.
There is one other required clarification of the restricted version of CP. “Justified belief” is ambiguous. It could be used to refer to a species of actually held beliefs — namely, those actually held beliefs of S that are justified. Or it could refer to propositions that S is entitled to hold — regardless of whether S does indeed hold them. If CP is to be acceptable, “justified belief” must be used so as to mean the latter for a reason already cited, i.e., belief does not transmit through entailment.
We are now in a position to ask: Does the restricted form of closure hold regarding what we are entitled to believe — even if we don't, in fact, believe it?
There appears to be a perfectly general argument for the restricted version. Let p entail q , and let us suppose that S is entitled to believe that p iff S has (non-overridden) grounds that make p sufficiently likely to be true: [ 13 ]
- If S is entitled to believe that p , then S has (non-overridden) grounds that make p sufficiently likely to be true. [by the supposition]
- If S has (non-overridden) grounds that make p sufficiently likely to be true, then S has (non- overridden) grounds making q sufficiently likely to be true. [because p entails q ]
- If S is entitled to believe that p , then S has (non-overridden) grounds making q sufficiently likely to be true. [from 1,2]
- If S has (non-overridden) grounds making q sufficiently likely to be true, then S is entitled to believe that q . [by the supposition]
- Therefore, if S is entitled to believe that p , S is entitled to believe that q . [from 2,3]
The supposition mentioned above seems plausible given that the debate over the merits of Academic Skepticism employs an evidentialist account of justification. That is, the debate between the Academic Skeptic and the Epistemist is over whether S has adequate grounds for EI-type propositions such that those grounds make p sufficiently likely to be true.
Premise 2 contains the key claim. In spite of the fact that the probabilities (whether subjective or objective) transmit through entailment, it has been challenged. Fred Dretske and others have produced cases in which they believe CP fails and fails precisely because Premise 2 in the general argument for CP is false. [ 14 ] Dretske writes:
… something's being a zebra implies that it is not a mule … cleverly disguised by the zoo authorities to look like a zebra. Do you know that these animals are not mules cleverly disguised? If you are tempted to say “Yes” to this question, think a moment about what reasons you have, what evidence you can produce in favor of this claim. The evidence you had for thinking them zebras has been effectively neutralized, since it does not count toward their not being mules cleverly disguised to look like zebras. (Dretske 1970, 1015-1016)
Dretske is speaking of “knowledge” rather than beliefs to which one is entitled, but that seems irrelevant since the issue concerns the supposed lack of a sufficient source of evidence or reasons for the claim that the animal is not a cleverly disguised mule. In other words, Dretske grants that S has (non-overridden) grounds that make it sufficiently likely that the animals are zebras, but he holds that S does not have (non-overridden) grounds making it sufficiently likely that the animals are not cleverly disguised mules because S's evidence for the former has been “effectively neutralized.”
The crucial thing to note about this proposed counterexample is that it works only if the Closure Principle entails that the very same source of evidence that justifies S in believing that the animals are zebras must justify S in believing that they are not cleverly disguised mules. Since the “evidence” for the former has been “effectively neutralized,” it is not available for the latter. Now, in response one could claim that once the question of whether the animals are disguised mules has been raised, the evidence is “effectively neutralized” for both the former and the latter, and S is no longer justified in believing that the animals are zebras. Thus, it could be held that this example could actually be used to support CP.
Nevertheless, let us grant that S 's evidence for the claim that the animals are zebras cannot be used to show that they are not cleverly disguised mules. It could be argued that this would not force giving up Premise 2 in the general argument for CP.
Such an argument could begin by recalling that Premise 2 claimed merely that whenever S had (non-overridden) grounds that make p sufficiently likely to be true, then S has (non-overridden) grounds for making q sufficiently likely to be true. It did not require that it was the very same grounds in both cases. Dretske's purported counterexample seems to require that CP implies that the adequate source of evidence is the same for both propositions. Thus, letting “ xRy ” mean that x provides an adequate evidence for y , the counter example depends upon assuming that if closure holds between p and q , then the evidence “path” must look like this:
Pattern 1 … Rp / / … Re \ \ … Rq
No doubt this constraint sometimes correctly portrays the relevant evidential relationships when some proposition, p , entails some other proposition, q . For example, suppose I have adequate evidence for the claim that Anne has two brothers, then it would seem that the very same evidence would be adequate for believing that Anne has at least one brother. But the defender of CP, and more particularly the Academic Skeptic, could point out that closure need not require that type of evidence path in all cases in which one proposition entails another.
Two are two other possibilities for instantiating closure that are captured by Premise 2 that can be depicted as follows:
Pattern 2 … ReRp … Rq pdf include--> Pattern 3 … Re (where e includes q ) Rp
In Pattern 2 cases there is some adequate evidence, e , for p ; and p , itself, is the adequate evidence for q , since p strictly implies q . For example, if I have adequate evidence for believing that 2 is a prime number, I can use that proposition as an adequate reason for believing that there is at least one even prime. Indeed, consider any belief arrived at as a result of deductive inference. In such a case, we legitimately infer the entailed proposition from the conjunction of the premises that entails it. The plausibility of the famous Gettier cases depends upon Pattern 2 type cases in which closure holds. Gettier says:
… for any proposition p , if S is justified in believing p , and p entails q , and S deduces q from p and accepts q as a result of this deduction, then S is justified in believing q . (Gettier 1963, 122) [ 15 ]
In Pattern 3 cases the order of the evidence is reversed because q serves as part of the evidence for p . For example, I am justified in believing that water is present if I am justified in believing that there is present a clear, odorless, watery-tasting and watery-looking fluid at standard temperature and pressure. This pattern is typical of abductive inferences. In addition, there are cases in which some contraries of h need to be eliminated prior to h 's being justified. For example, in the zebra-in-the-zoo case, if I had some reason to think that the animals were cleverly disguised mules, then it could be argued that such a contrary would need to be eliminated before I would be justified in believing that the animals were zebras.
The crucial point for the discussion here is that granting that there is no Pattern 1 type evidence path available to S in the zebra-in-the-zoo case does not require relinquishing premise 2 in the general argument for CP. The reason is simply that CP does not entail that there is Pattern 1 type evidence available in every case in which p entails q . Indeed, it could be suggested that the animals looking like zebras in a pen marked “zebras” is, ceteris paribus , adequate evidence to justify the claim that they are zebras; and once S is entitled to believe that the animals are zebras, S can, using the principle stated by Gettier, justifiably deduce that they are not cleverly disguised mules. That is, S can employ an evidence path like that depicted in Pattern 2. (See Klein 1981, 1995, and 2000a.) Further, if S had some reason to think that the animals were cleverly disguised mules, then S might have to eliminate that possibility before she could justifiably believe that they are zebras. In other words, S might have to employ an evidence path like the one depicted in Pattern 3. The point is that the Dretske-like counterexamples appear to depend upon the false claim that if Premise 2 in the general argument for CP is true, then the evidential relationship between the entailing and the entailed proposition is always correctly depicted by Pattern 1.
In addition to the purported counterexamples to closure that we have just examined, there are some general theories of knowledge in which closure fails. Robert Nozick's account of knowledge is the best such example. Roughly his account is this (Nozick 1981, 172-187):
S knows that p iff : S believes p ; p is true; if p were true, S would believe p ; if p were not true, S would not believe p .
This account is often referred to as a tracking account of knowledge because whenever S knows that p , S 's beliefs track p . Think of a guided missile tracking its target. If the target moves left, the missile moves left. If the target moves right, the missile moves to the right. According to the tracking account of knowledge our beliefs must track the truth as a guided missile tracks its target.
There is one important clarification of conditions 3 and 4 discussed by Nozick, namely, that the method by which S acquires the belief must be held constant from the actual world to the possible world. A doting grandmother might know that her grandchild is not a thief on the basis of very good evidence, but would still believe that he wasn't a thief, even if he were, because she loves him. So, we must require that the grandmother use the same method in both the actual and the near possible worlds, for otherwise condition 4 would exclude some clear cases of knowledge. This is not the place to provide a full examination of Nozick's account of knowledge. [ 16 ] What is crucial for our discussion is that it is easy to see that closure will fail for knowledge in just the kind of case that the Academic Skeptic is putting forward because of condition 4. Suppose S knows that there is a chair before her. Would she know that she is not in a skeptical scenario in which it merely appears that there is a chair? If the fourth condition were true, she would not know that because if she were in such a scenario, she would be fooled into thinking that she wasn't. Thus, either condition 4 is too strong or CP fails.
There are some reasons for thinking that condition 4 is too strong. Consider a relatively simple case in which S seems to have knowledge but condition 4 does not obtain. S looks at a thermometer that is displaying the temperature as 72 degrees. The thermometer is working perfectly and S comes to believe that the temperature is 72 degrees by reading the thermometer and coming to believe what it says. But if the temperature were not 72, suppose that something would affect the thermometer in a way that made it read “72,” so that by employing the same method (looking at the thermometer and coming to believe what it reads) S would still believe that it was 72. (One could imagine all kinds of circumstances that would have that causal result. A comical one: Imagine a lizard that is now sleeping on the thermometer that would stir were the temperature to rise, thus dislodging a small rock that hits the thermometer breaking the mercury column in a way that makes the thermometer still read 72.)
Or consider this case in the literature: You put a glass of ice-cold lemonade on a picnic table in your back yard. You go inside and get a telephone call from a friend and talk for half an hour. When you hang up you remember that you had left the ice-cold lemonade outside exposed to the hot sun and come to believe that it isn't ice-cold anymore. It would seem that you could know that even if in some near world a friend of yours who just happened to be walking by noticed the glass and happening to have a cooler full of ice with him put the glass of lemonade in the cooler to keep it ice-cold for you. Thus, if the lemonade were still ice-cold, you would believe that it wasn't. (See Vogel 1987, 206.)
The moral of these cases seems to be that S can know that p even if there are some near possible worlds in which p is false but S still believes that p (employing the same method of belief formation). Indeed, it could plausibly be maintained that what is required for knowledge is that the method of belief formation work in this world — exactly as it is — even if the method would fail were there to be some slight variation in the actual world.
Further Clarification of Closure
In order to clarify CP further, it would be useful to contrast it with a stronger principle. I have already pointed out that in some cases some contraries of h need to be eliminated before h becomes justified. Suppose, however, that the skeptic requires that all contraries to h be eliminated before h is justified. That is much stronger than CP because CP is compatible with Pattern 1 and Pattern 2 type evidential relationships. In neither is every contrary to h eliminated prior to h . In Pattern 2, the contrary of h is eliminated after h ; in Pattern 1, h is arrived at and its contrary is eliminated simultaneously. Keith Lehrer might be appealing to the stronger principle when he writes:
… generally arguments about where the burden of proof lies are unproductive. It is more reasonable to suppose that such questions are best left to courts of law where they have suitable application. In philosophy [emphasis added] a different principle of agnoiology [the study of ignorance] is appropriate, to wit, that no hypothesis should be rejected as unjustified without argument against it. Consequently, if the sceptic puts forth a hypothesis inconsistent with the hypothesis of common sense, then there is no burden of proof on either side … . (Lehrer 1971, 53)
The passage is open to more than one interpretation, but it will serve to illustrate my point, namely that there is a very strong principle — call it the “ Eliminate All Contraries First Principle ” [EACF Principle] — which requires that all evidence paths exhibit Pattern 3 and that the denials of all contraries to a given proposition appear prior to that proposition.
If EACF were accepted, there is a really easy route to Academic Skepticism. If it were required that the evidence, e , for some hypothesis, h , must contain the denials of all the contraries of h , it is clear that e would have to entail h . To see that, note that (~ h & p ) as well as (~ h & ~ p ) are contraries of h , and that it is not possible for both ~(~ h & p ) and ~(~ h & ~ p ) to be true and h to be false. Thus, if the skeptic were to adopt the EACF Principle, the evidence for h would have to entail h . (See Klein 1981, 100-104.) That requirement seems to be too strong for many, if not most, empirically contingent propositions. Hence, it could be plausibly argued that this is an inappropriate way to motivate skepticism because in so far as skepticism remains an interesting philosophical position, the skeptic cannot impose such an outrageous departure from our ordinary epistemic practices.
There is a related point worth mentioning. Note that even EAD, although requiring that we be able to reject or neutralize every potential ground for doubt (i.e., a proposition satisfying condition 1. in the definition of genuine doubt), does not require what EACF does. EAD does not require that we eliminate all of the grounds for doubt (including contraries) before we are justified in believing a hypothesis. Indeed, EAD allows for the possibility that we could use h , itself, or something that h justifies as the basis for rejecting or neutralizing some grounds for doubt.
Consideration of CP2
Now, with those clarifications of CP (and EAD) in mind, we can turn to CP2. It claims that we are not justified in denying the skeptical hypothesis — in other words that we are not justified in believing that we are not being deceived. What arguments can be given for CP2? It is tempting to suggest something like this: The skeptical scenarios are developed in such a way that it is supposed that we could not tell that we were being deceived. For example, we are asked to consider that there is an Evil Genius “so powerful” that it could (1) make me believe that there were hands when there were none and (2) make it such that I could not detect the illusion. But the skeptic must be very careful here. She cannot require that in order for S to know (or be justified in assenting to) something, say x , that if x were false, she would not still assent to x . We have just seen (while examining Nozick's account of knowledge) that this requirement is too strong. So the mere fact that there could be skeptical scenarios in which S still believes that she is not in such a scenario cannot provide the skeptic with a basis for thinking that she fails to know that she is not (actually) in a skeptical scenario. But even more importantly , were that a requirement of knowledge (or justification), then we have seen that closure would fail and, consequently, the basis for the first premise in the CP-style argument for Academic Skepticism would be forfeited. [ 17 ]
In addition, we have also seen that if CP is true, and it did seem to be true, then there is one evidence pattern between entailing and entailed propositions that might prove useful to the Epistemist at this point in the discussion. If S could be justified in believing some proposition that entailed the denial of the skeptical hypothesis, then S could be justified in denying that hypothesis by employing evidence Pattern 2. Indeed, as G. E Moore suggested (1962, 242), what is to prevent the Epistemist from claiming that S is justified in denying that she is in a skeptical scenario because S is justified in believing that she has hands and CP is true? A plausible answer to Moore seems to be something like this: The issue that is under dispute is whether S is justified in assenting to (or knows that) she has hands. Thus, the Epistemist cannot reject CP2 by assuming the denial of the conclusion of the skeptical argument. All well and good. But the same sauce cooks the gander, and the skeptic cannot claim as the reason for CP2 that since S is not justified in believing that she has hands, she cannot avail herself of that as her reason for being justified in believing that she is not in a skeptical scenario.
So, what reason can the skeptic give for CP2? I do not know of one that has been offered that is consistent with the defense of CP and that does not beg the question. That is not to say that CP2 is false. Far from it. Perhaps it is true. The issue here is whether we are justified in accepting or rejecting it. It seems that in order to accept it and CP, the skeptic would have to assert that S is not justified in believing that she has hands because evidence Pattern 2 depicts one way in which S could be justified in denying the skeptical scenario. But that would beg the question because the conclusion of the CP-style argument is nothing other than S is not justified in believing that she has hands. [ 18 ]
I had mentioned earlier that although there seemed to be only three responses available when confronting the CP-style argument for Academic Skepticism (accept the conclusion, reject one or both of the premises, or deny the validity of the argument), there is, in fact, a fourth alternative. That alternative is simply to point out that given the required defense of CP1 against the counterexample proposed by Dretske, there is no good argument for CP2 (because it would beg the question), and, hence, there is no good way to motivate Academic Skepticism with a CP-style argument.
Of course, the Pyrrhonian Skeptic might point to the possibility that there is also no good argument to the conclusion that we do have knowledge of EI-type propositions. Some might think that the Academic Skeptic wins in such a stand-off. But recall that what distinguishes the Academic Skeptic from the Pyrrhonian Skeptic is that only the Academic Skeptic assents to the claim that we cannot have knowledge. The Pyrrhonian Skeptic withholds judgement regarding whether we can have knowledge. And in a stand-off, the Pyrrhonian would seem to have appropriate attitude.
This concludes the discussion of CP-style skepticism. I would now like to briefly consider the second form of Academic Skepticism, namely the Cartesian-style that employs the Eliminate All Doubt Principle. Then, before we conclude our discussion of Academic Skepticism, I would like to consider one quite popular response to it — contextualism.
5. The Cartesian-style Argument for Academic Skepticism Employing the Eliminate All Doubt Principle
This section can be brief because we can apply the lessons learned in the discussion of CP-style arguments to an evaluation of the Cartesian-style arguments that employ EAD. First, it should be clear that the general argument for the Closure Principle, considered earlier, cannot be used as a model for a general argument for EAD. That argument depended crucially on the fact that h entailed ~ sk . (That is what provided the basis for premise 2 in the general argument for CP.) As we saw, the negation of a genuine ground for doubt need not be entailed by h . So, the skeptic has a much harder task in motivating EAD.
Nevertheless, let us grant that some argument could be provided that makes EAD plausible. The same dialectical issues that we have considered in discussing potential counterexamples to CP will recur regarding EAD. Reconsider the zebra-in-the-zoo case. But this time instead of the (contrary) proposition “the animals (I am seeing) are cleverly disguised mules,” consider a potential ground for doubt, i.e., “there are cleverly disguised mules within my perceptual field,” which according to EAD would have to be rejected or neutralized. Now, if the evidence I had for believing that the animals are zebras wasn't adequate to deny the former, it is certainly not adequate for the denying the later. So the EAD skeptic will have to appeal to the analogs of Pattern 2 and Pattern 3 type cases in order to save the principle from a Dretske-like counterexample. Thus, the skeptic employing EAD would be put in the same dialectical situation as the CP-style skeptic because she must provide a basis for the second premise in her argument for Academic Skepticism that (1) is compatible with her required defense of EAD against Dretske-like objections and (2) does not beg the question or appeal to a requirement that all grounds for doubt must be eliminated prior to a proposition being justified.
To sum up: The Cartesian-style skeptic employing EAD is in a worse dialectical position than the skeptic employing CP. Whatever problems are associated with CP skepticism transfer to EAD skepticism and, in addition, there appears to be no plausible general argument for EAD while there was one for CP.
6. Contextualism [ 19 ]
Examining the contextualist diagnosis of Academic Skepticism and its suggested solution will allow us to explore a question that remains concerning CP and EAD. It could be held that such skeptics need not employ CP or EAD in general, but rather more restricted versions, namely merely their instantiations as they appear in their respective arguments. The skeptic could maintain that there is something quite special about the skeptical hypothesis such that even though closure might not hold in general between any entailing proposition and every proposition it entails, it does hold between such propositions as “here's a hand” and “it does not merely appear that here is a hand.” Even more strongly, the skeptic could maintain that only the Pattern 3 type evidence path correctly depicts the evidential relationships between those propositions. Hence, in order to be justified in believing the former I must first eliminate the latter, where to eliminate a proposition means (here) nothing more than to be justified in denying it. The requirement that we eliminate all contraries to some proposition, h , before we are entitled to believe that h is too stringent for ordinary contexts, for the reasons cited, but perhaps when engaged in philosophy we have to be justified in believing that the skeptical hypothesis is false before the propositions of common sense are justified. That is essentially what the contextualists claim. They hold that in some contexts — philosophical ones — more stringent standards of evidence obtain than obtain in ordinary contexts. (For defenses of contextualism, see Cohen 1987, 1988; Lewis 1996; DeRose 1992, 1995)
There are two questions we should consider: Is contextualism about knowledge (or justified belief) the correct view to hold? If so, will it shed light on Academic Skepticism?
In answering the first question, it could be argued that contextualism with regard to the attribution of virtually any property is true. (Perhaps it doesn't apply to highly technical ones that only occur in one type of context.) For example, suppose that Mr. Lax says that Sam is happy. We discover that Lax is using “happy” to mean that a person is happy just in case he/she has had more happy moments than unhappy moments during a lifetime. Mr. Stringent demurs. For him, a person is happy only if he/she hardly ever experiences an unhappy moment.
Who is right about whether Sam is happy? Contextualists would say that they both could be because they are not using “happy” with the same criteria in mind. But it is crucial to note that given that each person recognizes that the other is applying different standards, Mr. Lax and Mr. Stringent can agree that, given what Lax means , Sam is happy and that, given what Stringent means , Sam is not happy.
Now, of course, we cannot employ any standards we please and still be speaking a common language. For example, Mr. Lax cannot legitimately lower the standards so as to make it the case that Sam is happy simply because he once was happy for a very short period and, similarly, Mr. Stringent cannot require that Sam is happy only if it is logically impossible that Sam experience an unhappy moment. There is a limited range, albeit rather wide, of appropriate standards for the application of a term.
The predicates “having knowledge,” “having adequate evidence,” “being justified,” and the like, do appear to be similar to most other predicates in this respect: Within a wide but non-arbitrary range of standards, speakers can legitimately demand that S have more or less of the relevant evidence for p before they will agree that “ S knows that p ” or “ S has adequate evidence for p .” So, the answer to the first question about the truth of contextualism seems to be: Contextualism about knowledge attributions is correct. It is just one instance of the general truth that standards for the application of a term vary within a wide but non-arbitrary range as determined by various features of the context.
Let us turn to the second and much more philosophically interesting question: Does the truth of this version of contextualism shed much, if any, light on Academic Skepticism? If it did, then the correct way to diagnose the dispute between the Academic Skeptic and the Epistemist would be to note that the Epistemist is using a lax standard and the skeptic a more stringent one. Having one's ordinary cake is compatible with eating one's skeptical cake because in the ordinary context we do have knowledge, but as standards rise to those employed by the skeptics, we do not have knowledge.
In response, it might be objected that this is not the proper diagnosis of the disagreement between the Academic Skeptic and the Epistemist. What the Academic Skeptic seems to be claiming is that we do not know what we ordinarily claim to know. We don't know EI-type propositions. That is, the Academic Skeptic claims that our ordinary knowledge claims are false. If she is merely claiming that on her standards we don't know, the skeptic's claims — like those of Mr. Stringent — can be granted and then promptly ignored because nothing that we formerly believed that we knew turns out not to have been known. The scope of our knowledge or justified beliefs in the ordinary context is left intact.
Thus, the parallel with the case of Sam's putative happiness seems to break down. In that case, Mr. Stringent would grant that Mr. Lax is correct given what Lax meant by “happy.” But the Academic Skeptic will not grant that the Epistemist is correct when he asserts that he has knowledge. The skeptic reasons that the Epistemist doesn't know that h , even given what the Epistemist means by “know,” because the Epistemist's justification for h isn't good enough. Indeed, the Academic Skeptic employing CP (or the stronger EAD) thinks that there cannot be any evidence for ~ sk no matter how low the standards are set; thus, h could not be known.
The issue seems to boil down to this: In the ordinary context is it true — as the Academic Skeptic claims — that in order to know that there are hands, we must first eliminate the skeptical hypothesis?
The Epistemist could argue that this is not required. Suppose we are looking at Dretske's zebras and someone asks whether we have eliminated the possibility that those are cleverly disguised aliens from some planet thousands of light years from our solar system? Or that they are not super-robots newly invented by some very clever third graders in Mrs. Johnson's English class? Or that they are not members of the lost tribe of Israel disguised as zebras who have been hiding out from the Assyrians since the 8th century BC.
Those are so far-fetched, the Epistemist could claim, that even if someone advancing those alternatives happens to believe them, there appears to be no reason why one should have to rise to the bait and eliminate those alternatives prior to being justified in believing that the animals are zebras. The Epistemist could continue by claiming that the skeptical hypothesis — that we are not in the actual world but rather in one which seems identical to it — is just as, or possibly even more, farfetched.
Thus the Epistemist could argue that try as she might, the Academic Skeptic cannot impose the burden of eliminating a farfetched hypothesis merely by raising it, even were she to believe it. On the other hand, the Epistemist could agree that in Dretske's zebra-in-the zoo case, if there really were some evidence, however slight, for the claim that the animals are painted mules, then Mr. Stringent might be able to legitimately require that S rule out that possibility prior to being justified in believing that the animals are zebras. But absent any evidence of that sort, the skeptic's requirements will fall on deaf ears. In parallel fashion, if there really were some evidence, however slight, that there is an evil genius making it merely appear that there are hands, then, perhaps the Academic Skeptic could legitimately require that S eliminate that possibility prior to being justified in believing that there are hands.
Put another way: The Epistemist can claim that the range of relevant alternatives is bounded by those propositions for which there is some, even minimal, evidence. The Epistemist will claim that it is a context-invariant feature of knowledge attributions that the relevant evidence does not include the denial of contraries for which there is no evidence whatsoever. The issue seems to be whether our ordinary knowledge claims are true — not whether they would be true in some context with requirements more stringent that those ordinarily applied.
As mentioned at the beginning of this essay, what distinguishes Pyrrhonian Skepticism from Academic Skepticism is that the former does not deny that we can have knowledge of what I have called EI-type propositions. They also would not assent to the Epistemist's claim that we can have such knowledge. Let us see how they arrived at that position.
To deny something is merely to assent to its negation. Since the Pyrrhonians took assent, i.e., the pro-attitude required for knowledge, to involve a kind of certainty that the matter had been finally and fully resolved, they did not assent to what they took to be non-evident propositions.
In distinguishing Pyrrhonism from the Academic Skeptics (in particular, Carneades and Cleitomachus), Sextus writes in Outlines of Pyrrhonism , [ PH ]:
… although both the Academics and the [Pyrrhonian] Skeptics say that they believe some things, yet here too the difference between the two philosophies is quite plain. For the word “believe” has different meanings; it means not to resist but simply to follow without any strong impulse or inclination, as the boy is said to believe his tutor; but sometimes it means to assent to a thing of deliberate choice and with a kind of sympathy due to strong desire, as when the incontinent man believes him who approves of an extravagant mode of life. Since, therefore, Carneades and Cleitomachus declare that a strong inclination accompanies their credence … while we say that our belief is a matter of simply yielding without any consent, here too there must be difference between us and them. ( PH I:230)
So, the Pyrrhonians would not assent to non-evident propositions. Of course, a crucial issue concerns the scope of the non-evident. To try to resolve that is beyond the scope of this essay (but see Burnyeat & Frede 1997). For our discussion we can suppose that a sufficient condition for some proposition being non-evident obtains whenever there can legitimately be disagreement about it. And, taking the cue from our discussion of Academic Skepticism, I think we can also safely stipulate that there can be legitimate disagreement about some proposition if there is some evidence for it and some evidence against it. So, the question is whether the proposition S can have knowledge of EI-type propositions can be the subject of legitimate disagreement.
Putting the matter that way seems to make the answer obvious. There are arguments for Academic Skepticism which have some plausibility, and some plausible objections to those arguments that support the Epistemist's view. Plausible arguments for something constitute some evidence for it. So, we can safely conjecture both that it is not evident that we can have knowledge of EI-type propositions and that it is not evident that such propositions necessarily fall outside our cognizance. Thus, the primary question then becomes this: What prompted the Pyrrhonian to withhold assent to all non-evident propositions?
The answer is that they found over and over again that neither experience nor reason was able to settle disputes about the non-evident. But the Pyrrhonians did not eschew what they called “appearances” or reasoning. Quite the contrary, the Greek for “skeptic” is closely related to the verb “ sképtomai ” which means “to inquire.” Thus, calling oneself a Pyrrhonian Skeptic did not imply a disregard for inquiry or reasoning. Indeed, the modes, to be discussed later, were not designed to inhibit reasoning. Rather, they were designed to assist the Pyrrhonian in continuing to inquire by shielding her from the disquieting state of dogmatism.
Pyrrhonian skepticism was thus a way of life without assent. As such, it has been ridiculed. The Pyrrhonian was likened to someone with Alzheimer's — surviving only if someone else were around to save him from all sorts of perils: falling into pits, being attacked by a dog or run over by a chariot. That caricature seems to miss the point that the Pyrrhonian only withheld assent with regard to the non-evident propositions. [ 20 ] Assent to what was evident (i.e., what appears to be) or a weaker pro-attitude toward the non-evident were commonplace.
As mentioned above, the Pyrrhonians would practice what they called the “modes” in order to try to assure that they were not “perturbed” by assenting. Like piano exercises for the fingers that would result in semi-automatic responses to the printed notes on a sheet of music, the modes were mental exercises that would result in semi-automatic responses to claims being made by the dogmatists — those who assented to the non-evident.
The Pyrrhonians believed that (but would not have assented to the claim that) there were two potential sources of knowledge: perception and reasoning. When the results of perception were introduced to settle a non-evident matter — say the actual color of an object (as opposed to how it appeared to someone), they would point out some or all of the following (Sextus Empiricus, PH I:40-128):
- Members of different species of animals probably perceive colors quite differently because their eyes are constructed differently.
- Members of the same species would have different perceptions of the color depending upon such things as the condition of their eyes, the nature of the medium of perception (varying light conditions for example), and the order in which objects were perceived.
Being reminded of the relativity of perception could incline a person to refrain from assenting to judgements of perception, when those judgements were about the “real” properties of the objects. As Sextus wrote:
… When we question whether the underlying object is such as it appears, we grant the fact that it appears, and our doubt does not concern the appearance itself, but the account given of the appearance. ( PH I:19-20)
Now, perhaps a careful analysis of what is meant by “real” properties coupled with a Cartesian-like answer to some of the doubts raised earlier in the Meditations would suffice to respond to the Pyrrhonian concerning the relativity of our senses. For example, if we took the “real” color of objects to be that property (or state) of the object, whatever it is, that produces perceptions of a certain sort in humans under “normal” circumstances and if we could distinguish (as Descartes suggested) normal from abnormal circumstances, then we might have a basis for resisting the Pyrrhonian modes concerning perception. But be that as it may, whether we can have knowledge of EI-type propositions is not a matter that is potentially resolvable by direct appeal to our senses. It will only be resolved if either the Epistemist or the Academic Skeptic has a compelling argument. Thus, the issue here is whether reasoning can settle matters.
The Pyrrhonians thought that there were modes which could induce withholding assent to the results of reasoning. It is to those modes that we should turn.
Perhaps the most influential passage in the corpus of the Pyrrhonian literature is a section from PH entitled “Five Modes of Agrippa.” Although the chapter title mentions five modes, two of them repeat those found elsewhere and are similar to the ones just discussed concerning perception. They are the modes of discrepancy and relativity and are important because they provide the background for understanding the description of the three modes concerning reasoning. Specifically, it is presumed that the relevant object of inquiry is subject to legitimate dispute and that reasoning is employed to resolve the dispute. The issue before us then is whether reasoning can legitimately lead to assent. Sextus writes:
The Mode based upon regress ad infinitum is that whereby we assert that the thing adduced as a proof of the matter proposed needs a further proof, and this again another, and so on ad infinitum , so that the consequence is suspension [of assent], as we possess no starting-point for our argument … We have the Mode based upon hypothesis when the Dogmatists, being forced to recede ad infinitum , take as their starting-point something which they do not establish but claim to assume as granted simply and without demonstration. The Mode of circular reasoning is the form used when the proof itself which ought to establish the matter of inquiry requires confirmation derived from the matter; in this case, being unable to assume either in order to establish the other, we suspend judgement about both. ( PH I:166-169)
The question is this: Supposing that the dogmatist assents to something, say p , on the basis of a reason, say q , and gives r as his reason for q , etc., how should the Pyrrhonian react in order to avoid the snares of dogmatism? The suggestion in this passage appears to be to force the dogmatist into either an apparently never ending regress or an arbitrary assertion or begging the question.
This strategy seems to be based upon the claim that there are (only) three possible patterns which any instance of reasoning can take. I will call the first pattern “infinitism.” Today we commonly refer to the second account as “foundationalism.” Finally, I will refer to the third possibility as “coherentism.”
The so-called “regress problem,” can be stated briefly in this way: There are only three possible patterns of reasoning. Either the process of producing reasons stops at a purported foundational proposition or it doesn't. If it does, then the reasoner is employing a foundationalist pattern. If it doesn't, then either the reasoning is circular, or it is infinite and non-repeating. There are no other significant possibilities. [ 21 ] Thus, if none of these forms of reasoning can properly lead to assent, then no form can.
So, we must look briefly at the reasons that a Pyrrhonian might have for thinking that foundationalism, coherentism and infinitism are inherently incapable of providing an adequate basis for assent. [ 22 ]
The Pyrrhonian is not (and cannot consistently be) assenting to the claim that foundationalism is false. Rather, a Pyrrhonian employing this mode would be attempting to reassure herself (and perhaps show the Epistemist) that the so-called foundational proposition stands in need of further support. In other words, the Pyrrhonian believes that a foundationalist cannot rationally practice his foundationalism because it inevitably leads to arbitrariness — i. e., assenting to a proposition which can legitimately be questioned but is, nevertheless, assented to without rational support.
So, how could the Pyrrhonian proceed? To begin to answer that question it is important to note that foundationalism comes in many forms. But all forms hold that the set of propositions can be partitioned into basic and non-basic propositions. Basic propositions have some autonomous bit of warrant that does not depend (at all) upon the warrant of any other proposition. [ 23 ] Non-basic propositions depend (directly or indirectly) upon basic propositions for all of their warrant.
Suppose that an inquirer, say Fred D'Foundationalist, has given some reasons for his beliefs. Fred offers q (where q could be a conjunction) for his belief that p , and he offers r (which could also be a conjunction) as his reason for q . Etc. Now, being a foundationalist, Fred finally offers some basic proposition, say b , as his reason for the immediately preceding belief. Sally D'Pyrrhonian asks Fred why he believes that b is true. Sally adds the “is true” to make clear to Fred that she is not asking what causes Fred to believe that b . She wants to know why Fred thinks that b is true. Now, Fred could respond by giving some reason for thinking that b is true even if b is basic, because basic propositions could have some non-autonomous warrant that depends upon the warrant of other propositions. But that is merely a delaying tactic since Fred is not a coherentist. In other words, he might be able to appeal to the conjunction of some other basic propositions and the non-basic propositions that they warrant as a reason for thinking that b is true. But Sally D'Pyrrhonian will ask whether he has any reason that does not appeal to another member in the set of basic propositions for thinking that each member in the set is true. If he says that he has none, then he has forfeited his foundationalism because he is really a closet coherentist. Being true to his foundationalism, he must think that there is some warrant that each basic proposition has that does not depend upon the warrant possessed by any other proposition.
The crucial point to note here is that Sally can grant that the proposition has autonomous warrant but continue to press the issue because she can ask Fred whether the possession of autonomous warrant is at all truth conducive. That is, she can ask whether a proposition with autonomous warrant is, ipso facto , at all likely to be true. If Fred says “yes,” then the regress will have continued. For he has this reason for thinking that b is true: “ b has autonomous warrant and propositions with autonomous warrant are somewhat likely to be true.” If he says “no” then Sally can point out that he is being arbitrary since she has asked why he thinks b is true and he has not been able to provide an answer.
Let us look at an example. Often it is held that first-person introspective reports are basic because they have some “privileged” status. My basic reason for thinking that there is an “external” object of a certain sort is that I am having an experience of a certain sort. Now, what Sally should ask is this: “Why do you think you are having an experience of that sort?” Or, again to emphasize that she is not asking for an explanation of the etiology of Fred's belief that he is having an experience of that sort, she could ask: “Why do you think that the proposition ‘I am having an experience of a certain sort’ is true?”
The dilemma is that either Fred has a reason for thinking that proposition is true or he doesn't. If he does, then the regress has not stopped — in practice . If he doesn't, then he is being arbitrary — in practice .
Once again, it is crucial to recall that Pyrrhonians are not claiming that foundationalism is false. They could grant that some propositions do have autonomous warrant which is truth-conducive and that all other propositions depend for some of their warrant upon those basic propositions. What lies at the heart of their view is that there is a deep irrationality in being a practicing self-conscious foundationalist. The question to Fred can be put this way: On the assumption that you cannot appeal to any other proposition, do you have any reason for thinking that b is true? Fred not only won't have any such reason for thinking b is true, given that assumption, he cannot have one (if he remains true to his foundationalism). Arbitrariness seems inevitable. Of course, foundationalists typically realize this and, in order to avoid arbitrariness, tell some story (for example, about privileged access) that, if true, would provide a reason for thinking basic propositions are at least somewhat likely to be true. But then, the regress of reasons has continued.
At its base, coherentism holds that there are no propositions with autonomous warrant. But it is important to note that coherentism comes in two forms. What I choose to call the “warrant-transfer form” responds to the regress problem by suggesting that the propositions are arranged in a circle and that warrant is transferred within the circle — just as basketball players standing in a circle pass the ball from one player to another. (See Sosa 1980, and BonJour 1978.) I could, for example, reason that it rained last night by calling forth my belief that there is water on the grass and I could reason that there is water (as opposed to some other liquid, say glycerin, that looks like water) on the grass by calling forth my belief that it rained last night.
Long ago, Aristotle pointed out that this process of reasoning could not resolve matters. As he put it: This is a “simple way of proving anything” ( Posterior Analytics , I, iii, 73a5). The propositions in the circle might be mutually probability enhancing, but the point is that we could just as well have circular reasoning to the conclusion that it did not rain last night because the liquid is not water and the liquid is not water because it did not rain last night. In this fashion anything could be justified — too simply! It is ultimately arbitrary which set of mutually probability enhancing propositions we believe because there is no basis for preferring one over the other.
The warrant-transfer coherentist could reply to this objection by claiming that there is some property, P , in one of the two competing circles that is not present in the other and the presence of that property makes the propositions in one and only one of the circles worthy of assent. For example, in one and only one of the circles are there propositions that we actually believe, or perhaps believe spontaneously. [ 24 ] But, then, it seems clear that the warrant-transfer coherentist has adopted a form of foundationalism since he is now claiming that all and only the propositions in circles with P have some autonomous bit of warrant. And, all that we have said about the dilemma facing the foundationalist transfers immediately. Is the possession of P truth conducive or not? If it is … You can see how that would go.
So much for the warrant-transfer version of coherentism. The second form of coherentism, what we can call the “warrant-emergent form” does not imagine the circle as consisting of propositions that transfer their warrant from one proposition to another. Rather warrant for each proposition in the circle obtains because it is a member of a set of mutually probability enhancing propositions. Coherence itself is the property in virtue of which each member of the set of propositions has warrant. Warrant emerges all at once, so to speak, from the web-like structure of the set of propositions. The coherentist can then argue that the fact that the propositions cohere provides each of them with some prima facie credibility.
This might initially seem to be a more plausible view since it avoids the circularity charge. But, aside from the problem that are too many competing circles that are coherent, the coherentist has, once again, embraced foundationalism. The coherentist is now explicitly assigning some initial positive warrant to all of the individual propositions in a set of coherent propositions that does not depend upon the warrant of any other proposition in the set. In other words, he is assigning to them what we have called the autonomous bit of warrant and, once again, the dilemma facing the foundationalist returns.
The third mode is designed to provide the Pyrrhonian with a way of responding to a dogmatist who assents to some EI-type proposition, x , and ceaselessly provides new answers to the question “What reason do you have for x ?” Since there is always another reason, one that has not already been employed, that needs to be given for any offered reason, assenting to x would be inappropriate. Since the Pyrrhonian (or the Epistemist) does not know either whether there is such an infinite set of reasons available or whether there is no such set available, withholding assent to the proposition that knowledge of EI-type propositions is possible seems appropriate.
For those reasons, infinitism (as far as I can tell) has never been seriously considered as a model of reasoning suitable for the dogmatist because it is obvious that it cannot provide a model of reasoning that could lead to assent. A disputed proposition could never be fully justified for whenever a reason is provided the infinitist is committed to thinking that in order to settle the matter another, as yet “unused,” reason must be provided. Since that process can never be completed, infinitism cannot provide the dogmatist with a model that will settle matters. [ 25 ]
It appears that the Pyrrhonian has a viable strategy for resisting dogmatism because no process of reasoning is such that assent — that is, holding that the matter in question has been settled — is the appropriate attitude to have toward any non-evident proposition.
- Audi, R., 1988, Belief, Justification and Knowledge , Belmont, California: Wadsworth.
- BonJour, L., 1978, “Can Empirical Knowledge Have a Foundation?” American Philosophical Quarterly , 15/1: 1-13.
- ------, 1985, The Structure of Empirical Knowledge , Cambridge, Mass: Harvard University Press.
- Burnyeat, M., and Frede, M., 1997, The Original Sceptics: A Controversy , Indianapolis/Cambridge: Hackett Publishing Co.
- Cohen, S., 1987, “Knowledge, Context and Social Standards,” Synthese , 73: 3-26.
- -----, 1988, “How to be a Fallibilist,” Philosophical Perspectives , 2: 91-123.
- DeRose K., 1992, “Contextualism and Knowledge Attributions,” Philosophy and Phenomenological Research , 52: 913-929.
- -----, 1995, “Solving the Skeptical Problem,” Philosophical Review , 104: 1-52.
- DeRose, K., and Warfield, T. (eds.), 1999, Skepticism: A Contemporary Reader , New York and Oxford: Oxford University Press.
- Descartes, R., Meditations on First Philosophy , in E. Haldane and G. Ross (eds.), Philosophical Works of Descartes , Volume 1, Dover Publications, 1931.
- Dretske, F., 1970, “Epistemic Operators,” Journal of Philosophy , 67: 1007-1023.
- Gettier, E., 1963, “Is Knowledge True Justified Belief?” Analysis , 23: 121-123.
- Irwin, W., 2002, The Matrix and Philosophy , La Salle: Open Court.
- -----, 2005, More Matrix and Philosophy , La Salle: Open Court.
- Klein, P., 2003, “How a Pyrrhonian Skeptic Might Respond to Academic Skepticism,” in The Skeptics: Contemporary Essays , Steven Luper (ed.), London: Ashgate Press, pp. 75-94
- -----, 2002, “Skepticism” in The Oxford Handbook of Epistemology , P. Moser (ed.), Oxford: Oxford University Press, 336-361.
- -----, 2000a, “Contextualism and the Real Nature of Academic Skepticism,” Philosophical Issues , 10: 108-116.
- -----, 2000b, “Why Not Infinitism?” Epistemology: Proceedings of the Twentieth World Congress in Philosophy , R. Cobb-Stevens (ed.), 2000, Volume 5, pp. 199-208.
- -----, 1999, “Human Knowledge and the Infinite Regress of Reasons,” Philosophical Perspectives , 13: 297-325.
- -----, 1995, “Skepticism and Closure: Why the Evil Genius Argument Fails,” Philosophical Topics , 23/1 (Spring): 213-236.
- -----, 1981, Certainty: A Refutation of Scepticism , Minneapolis: University of Minnesota Press.
- Lehrer, K., 2000, Theory of Knowledge , Boulder, Colorado: Westview Press, second edition.
- -----, K., 1971, “Why Not Skepticism?” The Philosophical Forum , 2/3, 283-298. (Page reference is to the reprint in The Theory of Knowledge , L. Pojman (ed.), Belmont, CA: Wadsworth Publishing Company, 1993.)
- -----, 1997, Self-Trust: A Study of Reason, Knowledge and Autonomy , Oxford: Clarendon Press, Oxford University Press.
- Lewis, D., 1996, “Elusive Knowledge,” Australian Journal of Philosophy , 74: 549-67.
- Luper-Foy, S., (ed.), 1987, The Possibility of Knowledge , Totowa, NJ: Rowman & Littlefield.
- Malcolm, N., 1963, Knowledge and Certainty , Englewood Cliffs, NJ: Prentice-Hall.
- Moore, G.E., 1962, “Certainty” in Philosophical Papers , New York, NY: Collier Books.
- Nozick, R., 1981, Philosophical Explanations , Cambridge, Massachusetts: Harvard University Press.
- Radford, C., 1966, “Knowledge -- By Example,” Analysis , 27: 1-11.
- Sextus Empiricus, Outlines of Pyrrhonism [PH], R. G. Bury, trans., Cambridge, Massachusetts: Harvard University Press, 1967.
- Sextus Empiricus, Against the Logicians , R. G. Bury, trans., Cambridge, Massachusetts: Harvard University Press, 1967.
- Sosa, E., 1980, “The Raft and the Pyramid,” Midwest Studies in Philosophy , 5: 3-25.
- Stroud, B., 1984, The Significance of Philosophical Scepticism , Oxford: Clarendon Press.
- Unger, P., 1975, Ignorance: A Case for Scepticism , Oxford: Oxford University Press.
- Vogel, J., 1987, “Tracking, Closure and Inductive Knowledge” in Luper-Foy 1987.
- Wittgenstein, L., 1969, On Certainty , New York: Harper Torchbooks.
- Links to papers on Skepticism , in the Epistemology Research Guide, maintained by Keith Korcz (U. Louisiana/Fayetteville)
Descartes, René: epistemology | justification, epistemic: coherentist theories of | justification, epistemic: foundationalist theories of | justification, epistemic: internalist vs. externalist conceptions of | skepticism: ancient
Acknowledgments
I wish to thank Anne Ashbaugh and Laurence BonJour for their help with this entry. I should also note that some parts of the entry rely upon and, in some cases, significantly repeat sections of Klein 2002. I also relied on parts of Klein 1995, 1999, 2000a, and 2003.
Why Skepticism?
Steven novella.
Twenty years ago, I became actively involved in the skeptical movement when I and several others founded a humble local skeptical group. We were inspired by CSICOP (now CSI) and Skeptical Inquirer to add what we could to efforts to make the world a more skeptical place.
Over the past two decades, the skeptical landscape has changed quite a bit, but one constant has been the endless question: What is skepticism? What exactly do we do and why? As the movement has grown and diversified, the question has become only more complex.
What Is the Mission of the Skeptical Movement?
I have come to understand that scientific skepticism is a weird beast that is often difficult to understand, especially from the outside. We are not exactly scientists or journalists or lobbyists or educators, and yet we are all of those things to some extent.
I think the best way to explain scientific skepticism is that it is expertise in everything that can go wrong with science and belief, and it includes execution, communication, education, and regulation. It combines knowledge of science, philosophy, and critical thinking with special expertise in flawed reasoning and deception.
To understand this better, here is a list of what scientific skeptics promote and do.
Respect for Knowledge and Truth : Skeptics value reality and what is true. We therefore endeavor to be as reality-based as possible in our beliefs and opinions. This means subjecting all claims to a valid process of evaluation.
Methodological Naturalism : Skeptics believe that the world is knowable because it follows certain rules or laws of nature. The only legitimate methods for knowing anything empirical about the universe follows this naturalistic assumption. In other words, within the realm of the empirical you don’t get to invoke magic or the supernatural.
Promotion of Science : Science is the only set of methods for investigating and understanding the natural world. Science is therefore a powerful tool and one of the best developments of human civilization. We therefore endeavor to promote the role of science in our society, public understanding of the findings and methods of science, and high-quality science education. This includes protecting the integrity of science and education from ideological intrusion or antiscientific attacks. This also includes promoting high-quality science, which requires examining the process, culture, and institutions of science for flaws, biases, weaknesses, conflicts of interest, and fraud.
Promotion of Reason and Critical Thinking : Science works hand-in-hand with logic and philosophy, and therefore skeptics also promote understanding of these fields and the promotion of critical thinking skills.
Science vs. Pseudoscience : Skeptics seek to identify and elucidate the borders between legitimate science and pseudoscience, to expose pseudoscience for what it is, and to promote knowledge of how to tell the difference.
Ideological Freedom/Free Inquiry : Science and reason can flourish only in a secular society in which no ideology (religious or otherwise) is imposed upon individuals or the process of science or free inquiry.
Neuropsychological Humility : Being a functional skeptic requires knowledge of all the various ways in which we deceive ourselves, the limits and flaws in human perception and memory, the inherent biases and fallacies in cognition, and the methods that can help mitigate all these flaws and biases.
Consumer Protection : Skeptics endeavor to protect themselves and others from fraud and deception by exposing fraud and educating the public and policy-makers to recognize deceptive or misleading claims or practices.
Addressing Specific Claims : Skeptics combine all of the above to address specific claims that are flawed, biased, or pseudoscientific and to engage in the public discussion of these claims.
Cultural Memory : Skeptics as a whole act as the cultural memory for pseudosciences and scams of the past. Such beliefs tend to repeat themselves, and remembering the past can be very useful in quickly putting such beliefs into their proper perspective.
Science Journalism : Many skeptics spend a large portion of their time doing straight science communication and journalism, which is important because science is so central to our mission. This is also an important skill to explore and develop because it is so rarely done well. Correcting and criticizing bad science news reporting, especially in the Internet age, has become a large part of what skeptics do.
What Topics Do We Cover?
Traditional skepticism addresses a very broad range of topics: all of alternative medicine, parapsychology, cryptozoology, conspiracy theories, scams, postmodernism, self-help, education, science and the media, neuroscience and self-deception, fringe science, and a long list of topics that have political, religious, or social implications: genetically modified foods, organic farming, free energy and other energy issues, climate change, creationism, miracle claims, faith-healing, prophesy, channeling—the list is massive.
There has been frequent discussion about which topics skeptics “should” cover. My approach has always been that everyone, of course, should feel free to cover whatever topics suit their interests, motivations, and talents. There are no right or wrong topics to cover.
There are, however, many considerations worth discussing. Skepticism is a method of applying science and critical thinking to all areas. It is worth thinking about how those methods relate to any particular topic of interest.
Here are some of the factors I consider when deciding what topics to address as part of my skeptical activism.
Teachable Moment : One very important criterion is this: Would addressing a claim or topic provide a useful teachable moment? Since one (if not the) primary goal of skepticism is education, this is a crucial criterion, and in fact it is often sufficient reason to address a topic.
This is the primary reason I have never addressed issues such as ghosts, Bigfoot, astrology, or the Bermuda Triangle (classic skeptical topics all). I honestly don’t care at all about ghosts, and I agree that this has extremely low priority as an issue. However, ghost hunters engage in a variety of pseudoscientific activities and defend their claims with numerous logical fallacies.
There are many generic lessons about science and critical thinking that can be learned by examining any pseudoscience, and often the most obvious ones are the best examples.
I have also found that by examining the full spectrum of pseudoscience, I have been able to see recurring patterns that enable me to understand pseudoscience much more thoroughly and then apply those lessons to more important areas such as medicine.
Interest : Related to the teachable moment criterion is public interest. The whole point is to engage the public, and one technique for doing so is to go to where the people already are. The public is interested in ghosts, cryptids, and UFOs, and in fact they often learn pathological science from popular treatments of these topics.
If we leave these popular subjects to the charlatans, they will happily spread scientific illiteracy unopposed. This is, however, a great opportunity to teach the public about how science actually operates, mechanisms of self-deception, how to tell if a claim is valid, and how to detect pseudoscience.
Addressing pseudoscience and the paranormal is a way to popularize science, such as writing about the physics of Star Trek or the philosophy of The Simpsons . Ghosts and UFOs are the hook; the payoff is scientific literacy and the ability to think a bit more critically.
Impact : The relative impact or importance of an issue is definitely important, and nothing I write here should be interpreted as dismissing or minimizing that point. In fact, as the skeptical movement has matured over the past few decades I have noticed a definite shift to issues of greater social importance.
My primary issue is alternative medicine, the abject infiltration of fraud and pseudoscience into the institutions of healthcare. This results in the wasting of billions of dollars and diverting of research funds, and it causes direct harm to the health of individuals.
Other important issues we tackle regularly are vaccine refusal, global climate change, genetically modified foods, our energy infrastructure, future technology, teaching creationism and other pseudoscience in science classes, issues surrounding mental illness, the self-help industry, scams, racial or gender pseudoscience, and other issues that have a direct impact on people’s lives and our civilization. We also may consider how much of an effect we can have. Some issues are more amenable to scientific information than others.
Expertise : The world needs all kinds of experts, and scientific skepticism is a legitimate area of expertise. It involves a deep knowledge of pseudoscience, the philosophy of science, mechanisms of deception, neuropsychological humility, scams, logic, and other aspects of critical thinking. This includes knowledge of the history of pseudoscience.
Within skepticism, individuals also tend to focus their writing and speaking on their area of scientific expertise. So, skeptical doctors focus on medicine, astronomers on astronomy, biologists on issues such as evolution and creation, physicists on free energy, and so on.
If we have a bias, it is toward the areas of expertise that also tend to attract people to the skeptical movement itself, but this is hard to avoid. It is also not simple to correct, and straying outside of our areas of expertise is not a good solution. At the very least, it takes a lot more work to address an issue about which I am not already fairly expert.
Filling a Need : Very relevant to the question of what targets skeptics choose is who else, if anyone, is already addressing those problems. For example, reviewing evidence and establishing a standard of care for a particular issue within mainstream medicine is very important, but there are already professional societies that do that. All physicians and scientists should be skeptical, but in areas where mainstream scientists are already doing just fine at addressing misperceptions, physicians who have an expertise in skepticism are not needed and have nothing particular to add.
We tend to focus our efforts where there is the most need, meaning where there is a current lack of attention. Fringe ideas tend not to get attention from scientists, who don’t want to waste their time. Whether or not this is a reasonable position is debatable, but meanwhile skeptics are happy to fill the void. As a skeptical neurologist, for example, I am not going to spend my time delving into and engaging in debate over the possible mechanisms of Parkinson’s disease. There are scientists who are doing that. But I will engage with those claiming that near-death experiences are evidence for an afterlife because most scientists don’t bother to do that.
Journalistic Integrity : This last criterion is a bit of a personal choice. Some journalists and outlets unapologetically advocate for a political ideology. Everyone knows the Huffington Post is a liberal news source, for example. Some journalists, however, try to be as politically neutral as possible so that they will be viewed as a fair arbiter of factual information and analysis.
Similarly, some skeptics combine their skeptical activism with ideological activism. I have no problem with this, and most are upfront about it. Some skeptics, however, choose to be political or ideologically neutral in their activism, except for a defense of science and reason. I think this can be helpful.
While I certainly do have political opinions, I try to keep them separate from questions of science and evidence. If, for example, I am discussing global warming, I want to focus on the science and not be dismissed as liberal. Or if I am writing about GMOs, I do not want to be dismissed as conservative or libertarian. That can still happen, sometimes simultaneously, because people make unwarranted self-serving assumptions, but it helps when it is untrue. My opinions on these and similar topics are informed by the science, not my politics. This becomes a harder sell when you are also advocating for a political position. I also think it is helpful to have a movement that is based upon evidence and logic and is agnostic toward ideological positions or values, which are tangential.
Finally, it is more challenging to be neutral and unbiased when dealing with an issue about which you have a passionate ideological belief. You can make a reasonable argument for steering clear of such issues when trying to communicate objective science, or at least proceeding especially carefully. Otherwise you risk damaging your reputation as a science communicator.
What about Religion?
More often than not, the question of what skeptics do and what topics we address comes to the topic of religion. While I believe I have addressed all the relevant issues above, this is a common enough question that it is worth special mention.
No skeptical activist I know treats religious claims differently from any other type of claims. Any claim to empirical truth or scientific knowledge, whether based ultimately in religious ideology or social or political ideology, is fair game. The criteria I outlined above apply. Philosophical arguments are also fair game, as the tools of logic and critical thinking apply.
However, it is important to recognize that faith statements are simply different from scientific or philosophical statements. This does not mean they are exempt from critical thinking; it just means you need to be aware of the context and address them properly. This distinction, however, is often misinterpreted as avoiding religious claims, which is patently not true.
When a believer states that they believe something to be true based upon their own personal faith, there are a number of valid approaches. It is important to point out that the principles of freedom of religion and separation of church and state require that their personal faith not be imposed upon others. They don’t have a right to make other people follow their faith, to deprive their children of the basic necessities of life, or expect government to legislate their faith.
It is also useful to point out that beliefs based purely on faith are not subject to scientific analysis, and therefore they do not belong in the arena of science. They therefore cannot mix faith and science. Either the science stands on its own or it doesn’t. You cannot legitimately use faith to rescue bad science from refutation or to render it immune to falsification.
This applies to faith-based beliefs that are not overtly religious. It is not uncommon for believers in alien visitation or extrasensory perception (ESP) to retreat to faith-based claims when the evidence does not support their position. They are effectively refuted by simply stating that they have left the arena of science and therefore have ceded this territory. If they wish to have a religion of ESP, then so be it, but they cannot simultaneously claim to be backed by science.
In other words, skeptics can address faith claims epistemologically without making the same epistemological error as the believer in order to falsely claim that empirical methods can disprove beliefs that are not empirically based.
Being part of the skeptical movement for most of my adult life has been extremely fulfilling and tremendously educational. After two decades, I still find it among the most rewarding work that I do. There is also a continued great need for the expertise and work of activist skeptics. We are engaged in work that will never be completed. Our goals are generational, to slowly move our species in the direction of science and reason.
Count me in until entropy prevails over my temporary biological processes.
Steven Novella, MD, is an assistant professor of neurology at Yale University School of Medicine. He is the host of the Skeptics’ Guide to the Universe podcast, author of the NeuroLogica blog, executive editor of the Science-Based Medicine blog, and president of The New England Skeptical Society .
Advertisement
Scientific Thinking and Critical Thinking in Science Education
Two Distinct but Symbiotically Related Intellectual Processes
- Open access
- Published: 05 September 2023
Cite this article
You have full access to this open access article
- Antonio García-Carmona ORCID: orcid.org/0000-0001-5952-0340 1
3922 Accesses
Explore all metrics
Scientific thinking and critical thinking are two intellectual processes that are considered keys in the basic and comprehensive education of citizens. For this reason, their development is also contemplated as among the main objectives of science education. However, in the literature about the two types of thinking in the context of science education, there are quite frequent allusions to one or the other indistinctly to refer to the same cognitive and metacognitive skills, usually leaving unclear what are their differences and what are their common aspects. The present work therefore was aimed at elucidating what the differences and relationships between these two types of thinking are. The conclusion reached was that, while they differ in regard to the purposes of their application and some skills or processes, they also share others and are related symbiotically in a metaphorical sense; i.e., each one makes sense or develops appropriately when it is nourished or enriched by the other. Finally, an orientative proposal is presented for an integrated development of the two types of thinking in science classes.
Similar content being viewed by others
Philosophical Inquiry and Critical Thinking in Primary and Secondary Science Education
Fostering scientific literacy and critical thinking in elementary science education.
Rui Marques Vieira & Celina Tenreiro-Vieira
Enhancing Scientific Thinking Through the Development of Critical Thinking in Higher Education
Avoid common mistakes on your manuscript.
Education is not the learning of facts, but the training of the mind to think. Albert Einstein
1 Introduction
In consulting technical reports, theoretical frameworks, research, and curricular reforms related to science education, one commonly finds appeals to scientific thinking and critical thinking as essential educational processes or objectives. This is confirmed in some studies that include exhaustive reviews of the literature in this regard such as those of Bailin ( 2002 ), Costa et al. ( 2020 ), and Santos ( 2017 ) on critical thinking, and of Klarh et al. ( 2019 ) and Lehrer and Schauble ( 2006 ) on scientific thinking. However, conceptualizing and differentiating between both types of thinking based on the above-mentioned documents of science education are generally difficult. In many cases, they are referred to without defining them, or they are used interchangeably to represent virtually the same thing. Thus, for example, the document A Framework for K-12 Science Education points out that “Critical thinking is required, whether in developing and refining an idea (an explanation or design) or in conducting an investigation” (National Research Council (NRC), 2012 , p. 46). The same document also refers to scientific thinking when it suggests that basic scientific education should “provide students with opportunities for a range of scientific activities and scientific thinking , including, but not limited to inquiry and investigation, collection and analysis of evidence, logical reasoning, and communication and application of information” (NRC, 2012 , p. 251).
A few years earlier, the report Science Teaching in Schools in Europe: Policies and Research (European Commission/Eurydice, 2006 ) included the dimension “scientific thinking” as part of standardized national science tests in European countries. This dimension consisted of three basic abilities: (i) to solve problems formulated in theoretical terms , (ii) to frame a problem in scientific terms , and (iii) to formulate scientific hypotheses . In contrast, critical thinking was not even mentioned in such a report. However, in subsequent similar reports by the European Commission/Eurydice ( 2011 , 2022 ), there are some references to the fact that the development of critical thinking should be a basic objective of science teaching, although these reports do not define it at any point.
The ENCIENDE report on early-year science education in Spain also includes an explicit allusion to critical thinking among its recommendations: “Providing students with learning tools means helping them to develop critical thinking , to form their own opinions, to distinguish between knowledge founded on the evidence available at a certain moment (evidence which can change) and unfounded beliefs” (Confederation of Scientific Societies in Spain (COSCE), 2011 , p. 62). However, the report makes no explicit mention to scientific thinking. More recently, the document “ Enseñando ciencia con ciencia ” (Teaching science with science) (Couso et al., 2020 ), sponsored by Spain’s Ministry of Education, also addresses critical thinking:
(…) with the teaching approach through guided inquiry students learn scientific content, learn to do science (procedures), learn what science is and how it is built, and this (...) helps to develop critical thinking , that is, to question any statement that is not supported by evidence. (Couso et al., 2020 , p. 54)
On the other hand, in referring to what is practically the same thing, the European report Science Education for Responsible Citizenship speaks of scientific thinking when it establishes that one of the challenges of scientific education should be: “To promote a culture of scientific thinking and inspire citizens to use evidence-based reasoning for decision making” (European Commission, 2015 , p. 14). However, the Pisa 2024 Strategic Vision and Direction for Science report does not mention scientific thinking but does mention critical thinking in noting that “More generally, (students) should be able to recognize the limitations of scientific inquiry and apply critical thinking when engaging with its results” (Organization for Economic Co-operation and Development (OECD), 2020 , p. 9).
The new Spanish science curriculum for basic education (Royal Decree 217/ 2022 ) does make explicit reference to scientific thinking. For example, one of the STEM (Science, Technology, Engineering, and Mathematics) competency descriptors for compulsory secondary education reads:
Use scientific thinking to understand and explain the phenomena that occur around them, trusting in knowledge as a motor for development, asking questions and checking hypotheses through experimentation and inquiry (...) showing a critical attitude about the scope and limitations of science. (p. 41,599)
Furthermore, when developing the curriculum for the subjects of physics and chemistry, the same provision clarifies that “The essence of scientific thinking is to understand what are the reasons for the phenomena that occur in the natural environment to then try to explain them through the appropriate laws of physics and chemistry” (Royal Decree 217/ 2022 , p. 41,659). However, within the science subjects (i.e., Biology and Geology, and Physics and Chemistry), critical thinking is not mentioned as such. Footnote 1 It is only more or less directly alluded to with such expressions as “critical analysis”, “critical assessment”, “critical reflection”, “critical attitude”, and “critical spirit”, with no attempt to conceptualize it as is done with regard to scientific thinking.
The above is just a small sample of the concepts of scientific thinking and critical thinking only being differentiated in some cases, while in others they are presented as interchangeable, using one or the other indistinctly to talk about the same cognitive/metacognitive processes or practices. In fairness, however, it has to be acknowledged—as said at the beginning—that it is far from easy to conceptualize these two types of thinking (Bailin, 2002 ; Dwyer et al., 2014 ; Ennis, 2018 ; Lehrer & Schauble, 2006 ; Kuhn, 1993 , 1999 ) since they feed back on each other, partially overlap, and share certain features (Cáceres et al., 2020 ; Vázquez-Alonso & Manassero-Mas, 2018 ). Neither is there unanimity in the literature on how to characterize each of them, and rarely have they been analyzed comparatively (e.g., Hyytinen et al., 2019 ). For these reasons, I believed it necessary to address this issue with the present work in order to offer some guidelines for science teachers interested in deepening into these two intellectual processes to promote them in their classes.
2 An Attempt to Delimit Scientific Thinking in Science Education
For many years, cognitive science has been interested in studying what scientific thinking is and how it can be taught in order to improve students’ science learning (Klarh et al., 2019 ; Zimmerman & Klarh, 2018 ). To this end, Kuhn et al. propose taking a characterization of science as argument (Kuhn, 1993 ; Kuhn et al., 2008 ). They argue that this is a suitable way of linking the activity of how scientists think with that of the students and of the public in general, since science is a social activity which is subject to ongoing debate, in which the construction of arguments plays a key role. Lehrer and Schauble ( 2006 ) link scientific thinking with scientific literacy, paying especial attention to the different images of science. According to those authors, these images would guide the development of the said literacy in class. The images of science that Leherer and Schauble highlight as characterizing scientific thinking are: (i) science-as-logical reasoning (role of domain-general forms of scientific reasoning, including formal logic, heuristic, and strategies applied in different fields of science), (ii) science-as-theory change (science is subject to permanent revision and change), and (iii) science-as-practice (scientific knowledge and reasoning are components of a larger set of activities that include rules of participation, procedural skills, epistemological knowledge, etc.).
Based on a literature review, Jirout ( 2020 ) defines scientific thinking as an intellectual process whose purpose is the intentional search for information about a phenomenon or facts by formulating questions, checking hypotheses, carrying out observations, recognizing patterns, and making inferences (a detailed description of all these scientific practices or competencies can be found, for example, in NRC, 2012 ; OECD, 2019 ). Therefore, for Jirout, the development of scientific thinking would involve bringing into play the basic science skills/practices common to the inquiry-based approach to learning science (García-Carmona, 2020 ; Harlen, 2014 ). For other authors, scientific thinking would include a whole spectrum of scientific reasoning competencies (Krell et al., 2022 ; Moore, 2019 ; Tytler & Peterson, 2004 ). However, these competences usually cover the same science skills/practices mentioned above. Indeed, a conceptual overlap between scientific thinking, scientific reasoning, and scientific inquiry is often found in science education goals (Krell et al., 2022 ). Although, according to Leherer and Schauble ( 2006 ), scientific thinking is a broader construct that encompasses the other two.
It could be said that scientific thinking is a particular way of searching for information using science practices Footnote 2 (Klarh et al., 2019 ; Zimmerman & Klarh, 2018 ; Vázquez-Alonso & Manassero-Mas, 2018 ). This intellectual process provides the individual with the ability to evaluate the robustness of evidence for or against a certain idea, in order to explain a phenomenon (Clouse, 2017 ). But the development of scientific thinking also requires metacognition processes. According to what Kuhn ( 2022 ) argues, metacognition is fundamental to the permanent control or revision of what an individual thinks and knows, as well as that of the other individuals with whom it interacts, when engaging in scientific practices. In short, scientific thinking demands a good connection between reasoning and metacognition (Kuhn, 2022 ). Footnote 3
From that perspective, Zimmerman and Klarh ( 2018 ) have synthesized a taxonomy categorizing scientific thinking, relating cognitive processes with the corresponding science practices (Table 1 ). It has to be noted that this taxonomy was prepared in line with the categorization of scientific practices proposed in the document A Framework for K-12 Science Education (NRC, 2012 ). This is why one needs to understand that, for example, the cognitive process of elaboration and refinement of hypotheses is not explicitly associated with the scientific practice of hypothesizing but only with the formulation of questions. Indeed, the K-12 Framework document does not establish hypothesis formulation as a basic scientific practice. Lederman et al. ( 2014 ) justify it by arguing that not all scientific research necessarily allows or requires the verification of hypotheses, for example, in cases of exploratory or descriptive research. However, the aforementioned document (NRC, 2012 , p. 50) does refer to hypotheses when describing the practice of developing and using models , appealing to the fact that they facilitate the testing of hypothetical explanations .
In the literature, there are also other interesting taxonomies characterizing scientific thinking for educational purposes. One of them is that of Vázquez-Alonso and Manassero-Mas ( 2018 ) who, instead of science practices, refer to skills associated with scientific thinking . Their characterization basically consists of breaking down into greater detail the content of those science practices that would be related to the different cognitive and metacognitive processes of scientific thinking. Also, unlike Zimmerman and Klarh’s ( 2018 ) proposal, Vázquez-Alonso and Manassero-Mas’s ( 2018 ) proposal explicitly mentions metacognition as one of the aspects of scientific thinking, which they call meta-process . In my opinion, the proposal of the latter authors, which shells out scientific thinking into a broader range of skills/practices, can be more conducive in order to favor its approach in science classes, as teachers would have more options to choose from to address components of this intellectual process depending on their teaching interests, the educational needs of their students and/or the learning objectives pursued. Table 2 presents an adapted characterization of the Vázquez-Alonso and Manassero-Mas’s ( 2018 ) proposal to address scientific thinking in science education.
3 Contextualization of Critical Thinking in Science Education
Theorization and research about critical thinking also has a long tradition in the field of the psychology of learning (Ennis, 2018 ; Kuhn, 1999 ), and its application extends far beyond science education (Dwyer et al., 2014 ). Indeed, the development of critical thinking is commonly accepted as being an essential goal of people’s overall education (Ennis, 2018 ; Hitchcock, 2017 ; Kuhn, 1999 ; Willingham, 2008 ). However, its conceptualization is not simple and there is no unanimous position taken on it in the literature (Costa et al., 2020 ; Dwyer et al., 2014 ); especially when trying to relate it to scientific thinking. Thus, while Tena-Sánchez and León-Medina ( 2022 ) Footnote 4 and McBain et al. ( 2020 ) consider critical thinking to be the basis of or forms part of scientific thinking, Dowd et al. ( 2018 ) understand scientific thinking to be just a subset of critical thinking. However, Vázquez-Alonso and Manassero-Mas ( 2018 ) do not seek to determine whether critical thinking encompasses scientific thinking or vice versa. They consider that both types of knowledge share numerous skills/practices and the progressive development of one fosters the development of the other as a virtuous circle of improvement. Other authors, such as Schafersman ( 1991 ), even go so far as to say that critical thinking and scientific thinking are the same thing. In addition, some views on the relationship between critical thinking and scientific thinking seem to be context-dependent. For example, Hyytine et al. ( 2019 ) point out that in the perspective of scientific thinking as a component of critical thinking, the former is often used to designate evidence-based thinking in the sciences, although this view tends to dominate in Europe but not in the USA context. Perhaps because of this lack of consensus, the two types of thinking are often confused, overlapping, or conceived as interchangeable in education.
Even with such a lack of unanimous or consensus vision, there are some interesting theoretical frameworks and definitions for the development of critical thinking in education. One of the most popular definitions of critical thinking is that proposed by The National Council for Excellence in Critical Thinking (1987, cited in Inter-American Teacher Education Network, 2015 , p. 6). This conceives of it as “the intellectually disciplined process of actively and skillfully conceptualizing, applying, analyzing, synthesizing, and/or evaluating information gathered from, or generated by, observation, experience, reflection, reasoning, or communication, as a guide to belief and action”. In other words, critical thinking can be regarded as a reflective and reasonable class of thinking that provides people with the ability to evaluate multiple statements or positions that are defensible to then decide which is the most defensible (Clouse, 2017 ; Ennis, 2018 ). It thus requires, in addition to a basic scientific competency, notions about epistemology (Kuhn, 1999 ) to understand how knowledge is constructed. Similarly, it requires skills for metacognition (Hyytine et al., 2019 ; Kuhn, 1999 ; Magno, 2010 ) since critical thinking “entails awareness of one’s own thinking and reflection on the thinking of self and others as objects of cognition” (Dean & Kuhn, 2003 , p. 3).
In science education, one of the most suitable scenarios or resources, but not the only one, Footnote 5 to address all these aspects of critical thinking is through the analysis of socioscientific issues (SSI) (Taylor et al., 2006 ; Zeidler & Nichols, 2009 ). Without wishing to expand on this here, I will only say that interesting works can be found in the literature that have analyzed how the discussion of SSIs can favor the development of critical thinking skills (see, e.g., López-Fernández et al., 2022 ; Solbes et al., 2018 ). For example, López-Fernández et al. ( 2022 ) focused their teaching-learning sequence on the following critical thinking skills: information analysis, argumentation, decision making, and communication of decisions. Even some authors add the nature of science (NOS) to this framework (i.e., SSI-NOS-critical thinking), as, for example, Yacoubian and Khishfe ( 2018 ) in order to develop critical thinking and how this can also favor the understanding of NOS (Yacoubian, 2020 ). In effect, as I argued in another work on the COVID-19 pandemic as an SSI, in which special emphasis was placed on critical thinking, an informed understanding of how science works would have helped the public understand why scientists were changing their criteria to face the pandemic in the light of new data and its reinterpretations, or that it was not possible to go faster to get an effective and secure medical treatment for the disease (García-Carmona, 2021b ).
In the recent literature, there have also been some proposals intended to characterize critical thinking in the context of science education. Table 3 presents two of these by way of example. As can be seen, both proposals share various components for the development of critical thinking (respect for evidence, critically analyzing/assessing the validity/reliability of information, adoption of independent opinions/decisions, participation, etc.), but that of Blanco et al. ( 2017 ) is more clearly contextualized in science education. Likewise, that of these authors includes some more aspects (or at least does so more explicitly), such as developing epistemological Footnote 6 knowledge of science (vision of science…) and on its interactions with technology, society, and environment (STSA relationships), and communication skills. Therefore, it offers a wider range of options for choosing critical thinking skills/processes to promote it in science classes. However, neither proposal refers to metacognitive skills, which are also essential for developing critical thinking (Kuhn, 1999 ).
3.1 Critical thinking vs. scientific thinking in science education: differences and similarities
In accordance with the above, it could be said that scientific thinking is nourished by critical thinking, especially when deciding between several possible interpretations and explanations of the same phenomenon since this generally takes place in a context of debate in the scientific community (Acevedo-Díaz & García-Carmona, 2017 ). Thus, the scientific attitude that is perhaps most clearly linked to critical thinking is the skepticism with which scientists tend to welcome new ideas (Normand, 2008 ; Sagan, 1987 ; Tena-Sánchez and León-Medina, 2022 ), especially if they are contrary to well-established scientific knowledge (Bell, 2009 ). A good example of this was the OPERA experiment (García-Carmona & Acevedo-Díaz, 2016a ), which initially seemed to find that neutrinos could move faster than the speed of light. This finding was supposed to invalidate Albert Einstein’s theory of relativity (the finding was later proved wrong). In response, Nobel laureate in physics Sheldon L. Glashow went so far as to state that:
the result obtained by the OPERA collaboration cannot be correct. If it were, we would have to give up so many things, it would be such a huge sacrifice... But if it is, I am officially announcing it: I will shout to Mother Nature: I’m giving up! And I will give up Physics. (BBVA Foundation, 2011 )
Indeed, scientific thinking is ultimately focused on getting evidence that may support an idea or explanation about a phenomenon, and consequently allow others that are less convincing or precise to be discarded. Therefore when, with the evidence available, science has more than one equally defensible position with respect to a problem, the investigation is considered inconclusive (Clouse, 2017 ). In certain cases, this gives rise to scientific controversies (Acevedo-Díaz & García-Carmona, 2017 ) which are not always resolved based exclusively on epistemic or rational factors (Elliott & McKaughan, 2014 ; Vallverdú, 2005 ). Hence, it is also necessary to integrate non-epistemic practices into the framework of scientific thinking (García-Carmona, 2021a ; García-Carmona & Acevedo-Díaz, 2018 ), practices that transcend the purely rational or cognitive processes, including, for example, those related to emotional or affective issues (Sinatra & Hofer, 2021 ). From an educational point of view, this suggests that for students to become more authentically immersed in the way of working or thinking scientifically, they should also learn to feel as scientists do when they carry out their work (Davidson et al., 2020 ). Davidson et al. ( 2020 ) call it epistemic affect , and they suggest that it could be approach in science classes by teaching students to manage their frustrations when they fail to achieve the expected results; Footnote 7 or, for example, to moderate their enthusiasm with favorable results in a scientific inquiry by activating a certain skepticism that encourages them to do more testing. And, as mentioned above, for some authors, having a skeptical attitude is one of the actions that best visualize the application of critical thinking in the framework of scientific thinking (Normand, 2008 ; Sagan, 1987 ; Tena-Sánchez and León-Medina, 2022 ).
On the other hand, critical thinking also draws on many of the skills or practices of scientific thinking, as discussed above. However, in contrast to scientific thinking, the coexistence of two or more defensible ideas is not, in principle, a problem for critical thinking since its purpose is not so much to invalidate some ideas or explanations with respect to others, but rather to provide the individual with the foundations on which to position themself with the idea/argument they find most defensible among several that are possible (Ennis, 2018 ). For example, science with its methods has managed to explain the greenhouse effect, the phenomenon of the tides, or the transmission mechanism of the coronavirus. For this, it had to discard other possible explanations as they were less valid in the investigations carried out. These are therefore issues resolved by the scientific community which create hardly any discussion at the present time. However, taking a position for or against the production of energy in nuclear power plants transcends the scope of scientific thinking since both positions are, in principle, equally defensible. Indeed, within the scientific community itself there are supporters and detractors of the two positions, based on the same scientific knowledge. Consequently, it is critical thinking, which requires the management of knowledge and scientific skills, a basic understanding of epistemic (rational or cognitive) and non-epistemic (social, ethical/moral, economic, psychological, cultural, ...) aspects of the nature of science, as well as metacognitive skills, which helps the individual forge a personal foundation on which to position themself in one place or another, or maintain an uncertain, undecided opinion.
In view of the above, one can summarize that scientific thinking and critical thinking are two different intellectual processes in terms of purpose, but are related symbiotically (i.e., one would make no sense without the other or both feed on each other) and that, in their performance, they share a fair number of features, actions, or mental skills. According to Cáceres et al. ( 2020 ) and Hyytine et al. ( 2019 ), the intellectual skills that are most clearly common to both types of thinking would be searching for relationships between evidence and explanations , as well as investigating and logical thinking to make inferences . To this common space, I would also add skills for metacognition in accordance with what has been discussed about both types of knowledge (Khun, 1999 , 2022 ).
In order to compile in a compact way all that has been argued so far, in Table 4 , I present my overview of the relationship between scientific thinking and critical thinking. I would like to point out that I do not intend to be extremely extensive in the compilation, in the sense that possibly more elements could be added in the different sections, but rather to represent above all the aspects that distinguish and share them, as well as the mutual enrichment (or symbiosis) between them.
4 A Proposal for the Integrated Development of Critical Thinking and Scientific Thinking in Science Classes
Once the differences, common aspects, and relationships between critical thinking and scientific thinking have been discussed, it would be relevant to establish some type of specific proposal to foster them in science classes. Table 5 includes a possible script to address various skills or processes of both types of thinking in an integrated manner. However, before giving guidance on how such skills/processes could be approached, I would like to clarify that while all of them could be dealt within the context of a single school activity, I will not do so in this way. First, because I think that it can give the impression that the proposal is only valid if it is applied all at once in a specific learning situation, which can also discourage science teachers from implementing it in class due to lack of time or training to do so. Second, I think it can be more interesting to conceive the proposal as a set of thinking skills or actions that can be dealt with throughout the different science contents, selecting only (if so decided) some of them, according to educational needs or characteristics of the learning situation posed in each case. Therefore, in the orientations for each point of the script or grouping of these, I will use different examples and/or contexts. Likewise, these orientations in the form of comments, although founded in the literature, should be considered only as possibilities to do so, among many others possible.
Motivation and predisposition to reflect and discuss (point i ) demands, on the one hand, that issues are chosen which are attractive for the students. This can be achieved, for example, by asking the students directly what current issues, related to science and its impact or repercussions, they would like to learn about, and then decide on which issue to focus on (García-Carmona, 2008 ). Or the teacher puts forward the issue directly in class, trying for it be current, to be present in the media, social networks, etc., or what they think may be of interest to their students based on their teaching experience. In this way, each student is encouraged to feel questioned or concerned as a citizen because of the issue that is going to be addressed (García-Carmona, 2008 ). Also of possible interest is the analysis of contemporary, as yet unresolved socioscientific affairs (Solbes et al., 2018 ), such as climate change, science and social justice, transgenic foods, homeopathy, and alcohol and drug use in society. But also, everyday questions can be investigated which demand a decision to be made, such as “What car to buy?” (Moreno-Fontiveros et al., 2022 ), or “How can we prevent the arrival of another pandemic?” (Ushola & Puig, 2023 ).
On the other hand, it is essential that the discussion about the chosen issue is planned through an instructional process that generates an environment conducive to reflection and debate, with a view to engaging the students’ participation in it. This can be achieved, for example, by setting up a role-play game (Blanco-López et al., 2017 ), especially if the issue is socioscientific, or by critical and reflective reading of advertisements with scientific content (Campanario et al., 2001 ) or of science-related news in the daily media (García-Carmona, 2014 , 2021a ; Guerrero-Márquez & García-Carmona, 2020 ; Oliveras et al., 2013 ), etc., for subsequent discussion—all this, in a collaborative learning setting and with a clear democratic spirit.
Respect for scientific evidence (point ii ) should be the indispensable condition in any analysis and discussion from the prisms of scientific and of critical thinking (Erduran, 2021 ). Although scientific knowledge may be impregnated with subjectivity during its construction and is revisable in the light of new evidence ( tentativeness of scientific knowledge), when it is accepted by the scientific community it is as objective as possible (García-Carmona & Acevedo-Díaz, 2016b ). Therefore, promoting trust and respect for scientific evidence should be one of the primary educational challenges to combating pseudoscientists and science deniers (Díaz & Cabrera, 2022 ), whose arguments are based on false beliefs and assumptions, anecdotes, and conspiracy theories (Normand, 2008 ). Nevertheless, it is no simple task to achieve the promotion or respect for scientific evidence (Fackler, 2021 ) since science deniers, for example, consider that science is unreliable because it is imperfect (McIntyre, 2021 ). Hence the need to promote a basic understanding of NOS (point iii ) as a fundamental pillar for the development of both scientific thinking and critical thinking. A good way to do this would be through explicit and reflective discussion about controversies from the history of science (Acevedo-Díaz & García-Carmona, 2017 ) or contemporary controversies (García-Carmona, 2021b ; García-Carmona & Acevedo-Díaz, 2016a ).
Also, with respect to point iii of the proposal, it is necessary to manage basic scientific knowledge in the development of scientific and critical thinking skills (Willingham, 2008 ). Without this, it will be impossible to develop a minimally serious and convincing argument on the issue being analyzed. For example, if one does not know the transmission mechanism of a certain disease, it is likely to be very difficult to understand or justify certain patterns of social behavior when faced with it. In general, possessing appropriate scientific knowledge on the issue in question helps to make the best interpretation of the data and evidence available on this issue (OECD, 2019 ).
The search for information from reliable sources, together with its analysis and interpretation (points iv to vi ), are essential practices both in purely scientific contexts (e.g., learning about the behavior of a given physical phenomenon from literature or through enquiry) and in the application of critical thinking (e.g., when one wishes to take a personal, but informed, position on a particular socio-scientific issue). With regard to determining the credibility of information with scientific content on the Internet, Osborne et al. ( 2022 ) propose, among other strategies, to check whether the source is free of conflicts of interest, i.e., whether or not it is biased by ideological, political or economic motives. Also, it should be checked whether the source and the author(s) of the information are sufficiently reputable.
Regarding the interpretation of data and evidence, several studies have shown the difficulties that students often have with this practice in the context of enquiry activities (e.g., Gobert et al., 2018 ; Kanari & Millar, 2004 ; Pols et al., 2021 ), or when analyzing science news in the press (Norris et al., 2003 ). It is also found that they have significant difficulties in choosing the most appropriate data to support their arguments in causal analyses (Kuhn & Modrek, 2022 ). However, it must be recognized that making interpretations or inferences from data is not a simple task; among other reasons, because their construction is influenced by multiple factors, both epistemic (prior knowledge, experimental designs, etc.) and non-epistemic (personal expectations, ideology, sociopolitical context, etc.), which means that such interpretations are not always the same for all scientists (García-Carmona, 2021a ; García-Carmona & Acevedo-Díaz, 2018 ). For this reason, the performance of this scientific practice constitutes one of the phases or processes that generate the most debate or discussion in a scientific community, as long as no consensus is reached. In order to improve the practice of making inferences among students, Kuhn and Lerman ( 2021 ) propose activities that help them develop their own epistemological norms to connect causally their statements with the available evidence.
Point vii refers, on the one hand, to an essential scientific practice: the elaboration of evidence-based scientific explanations which generally, in a reasoned way, account for the causality, properties, and/or behavior of the phenomena (Brigandt, 2016 ). In addition, point vii concerns the practice of argumentation . Unlike scientific explanations, argumentation tries to justify an idea, explanation, or position with the clear purpose of persuading those who defend other different ones (Osborne & Patterson, 2011 ). As noted above, the complexity of most socioscientific issues implies that they have no unique valid solution or response. Therefore, the content of the arguments used to defend one position or another are not always based solely on purely rational factors such as data and scientific evidence. Some authors defend the need to also deal with non-epistemic aspects of the nature of science when teaching it (García-Carmona, 2021a ; García-Carmona & Acevedo-Díaz, 2018 ) since many scientific and socioscientific controversies are resolved by different factors or go beyond just the epistemic (Vallverdú, 2005 ).
To defend an idea or position taken on an issue, it is not enough to have scientific evidence that supports it. It is also essential to have skills for the communication and discussion of ideas (point viii ). The history of science shows how the difficulties some scientists had in communicating their ideas scientifically led to those ideas not being accepted at the time. A good example for students to become aware of this is the historical case of Semmelweis and puerperal fever (Aragón-Méndez et al., 2019 ). Its reflective reading makes it possible to conclude that the proposal of this doctor that gynecologists disinfect their hands, when passing from one parturient to another to avoid contagions that provoked the fever, was rejected by the medical community not only for epistemic reasons, but also for the difficulties that he had to communicate his idea. The history of science also reveals that some scientific interpretations were imposed on others at certain historical moments due to the rhetorical skills of their proponents although none of the explanations would convincingly explain the phenomenon studied. An example is the case of the controversy between Pasteur and Liebig about the phenomenon of fermentation (García-Carmona & Acevedo-Díaz, 2017 ), whose reading and discussion in science class would also be recommended in this context of this critical and scientific thinking skill. With the COVID-19 pandemic, for example, the arguments of some charlatans in the media and on social networks managed to gain a certain influence in the population, even though scientifically they were muddled nonsense (García-Carmona, 2021b ). Therefore, the reflective reading of news on current SSIs such as this also constitutes a good resource for the same educational purpose. In general, according to Spektor-Levy et al. ( 2009 ), scientific communication skills should be addressed explicitly in class, in a progressive and continuous manner, including tasks of information seeking, reading, scientific writing, representation of information, and representation of the knowledge acquired.
Finally (point ix ), a good scientific/critical thinker must be aware of what they know, of what they have doubts about or do not know, to this end continuously practicing metacognitive exercises (Dean & Kuhn, 2003 ; Hyytine et al., 2019 ; Magno, 2010 ; Willingham, 2008 ). At the same time, they must recognize the weaknesses and strengths of the arguments of their peers in the debate in order to be self-critical if necessary, as well as to revising their own ideas and arguments to improve and reorient them, etc. ( self-regulation ). I see one of the keys of both scientific and critical thinking being the capacity or willingness to change one’s mind, without it being frowned upon. Indeed, quite the opposite since one assumes it to occur thanks to the arguments being enriched and more solidly founded. In other words, scientific and critical thinking and arrogance or haughtiness towards the rectification of ideas or opinions do not stick well together.
5 Final Remarks
For decades, scientific thinking and critical thinking have received particular attention from different disciplines such as psychology, philosophy, pedagogy, and specific areas of this last such as science education. The two types of knowledge represent intellectual processes whose development in students, and in society in general, is considered indispensable for the exercise of responsible citizenship in accord with the demands of today’s society (European Commission, 2006 , 2015 ; NRC, 2012 ; OECD, 2020 ). As has been shown however, the task of their conceptualization is complex, and teaching students to think scientifically and critically is a difficult educational challenge (Willingham, 2008 ).
Aware of this, and after many years dedicated to science education, I felt the need to organize my ideas regarding the aforementioned two types of thinking. In consulting the literature about these, I found that, in many publications, scientific thinking and critical thinking are presented or perceived as being interchangeable or indistinguishable; a conclusion also shared by Hyytine et al. ( 2019 ). Rarely have their differences, relationships, or common features been explicitly studied. So, I considered that it was a matter needing to be addressed because, in science education, the development of scientific thinking is an inherent objective, but, when critical thinking is added to the learning objectives, there arise more than reasonable doubts about when one or the other would be used, or both at the same time. The present work came about motivated by this, with the intention of making a particular contribution, but based on the relevant literature, to advance in the question raised. This converges in conceiving scientific thinking and critical thinking as two intellectual processes that overlap and feed into each other in many aspects but are different with respect to certain cognitive skills and in terms of their purpose. Thus, in the case of scientific thinking, the aim is to choose the best possible explanation of a phenomenon based on the available evidence, and it therefore involves the rejection of alternative explanatory proposals that are shown to be less coherent or convincing. Whereas, from the perspective of critical thinking, the purpose is to choose the most defensible idea/option among others that are also defensible, using both scientific and extra-scientific (i.e., moral, ethical, political, etc.) arguments. With this in mind, I have described a proposal to guide their development in the classroom, integrating them under a conception that I have called, metaphorically, a symbiotic relationship between two modes of thinking.
Critical thinking is mentioned literally in other of the curricular provisions’ subjects such as in Education in Civics and Ethical Values or in Geography and History (Royal Decree 217/2022).
García-Carmona ( 2021a ) conceives of them as activities that require the comprehensive application of procedural skills, cognitive and metacognitive processes, and both scientific knowledge and knowledge of the nature of scientific practice .
Kuhn ( 2021 ) argues that the relationship between scientific reasoning and metacognition is especially fostered by what she calls inhibitory control , which basically consists of breaking down the whole of a thought into parts in such a way that attention is inhibited on some of those parts to allow a focused examination of the intended mental content.
Specifically, Tena-Sánchez and León-Medina (2020) assume that critical thinking is at the basis of rational or scientific skepticism that leads to questioning any claim that does not have empirical support.
As discussed in the introduction, the inquiry-based approach is also considered conducive to addressing critical thinking in science education (Couso et al., 2020 ; NRC, 2012 ).
Epistemic skills should not be confused with epistemological knowledge (García-Carmona, 2021a ). The former refers to skills to construct, evaluate, and use knowledge, and the latter to understanding about the origin, nature, scope, and limits of scientific knowledge.
For this purpose, it can be very useful to address in class, with the help of the history and philosophy of science, that scientists get more wrong than right in their research, and that error is always an opportunity to learn (García-Carmona & Acevedo-Díaz, 2018 ).
Acevedo-Díaz, J. A., & García-Carmona, A. (2017). Controversias en la historia de la ciencia y cultura científica [Controversies in the history of science and scientific culture]. Los Libros de la Catarata.
Aragón-Méndez, M. D. M., Acevedo-Díaz, J. A., & García-Carmona, A. (2019). Prospective biology teachers’ understanding of the nature of science through an analysis of the historical case of Semmelweis and childbed fever. Cultural Studies of Science Education , 14 (3), 525–555. https://doi.org/10.1007/s11422-018-9868-y
Bailin, S. (2002). Critical thinking and science education. Science & Education, 11 (4), 361–375. https://doi.org/10.1023/A:1016042608621
Article Google Scholar
BBVA Foundation (2011). El Nobel de Física Sheldon L. Glashow no cree que los neutrinos viajen más rápido que la luz [Physics Nobel laureate Sheldon L. Glashow does not believe neutrinos travel faster than light.]. https://www.fbbva.es/noticias/nobel-fisica-sheldon-l-glashow-no-cree-los-neutrinos-viajen-mas-rapido-la-luz/ . Accessed 5 Februray 2023.
Bell, R. L. (2009). Teaching the nature of science: Three critical questions. In Best Practices in Science Education . National Geographic School Publishing.
Google Scholar
Blanco-López, A., España-Ramos, E., & Franco-Mariscal, A. J. (2017). Estrategias didácticas para el desarrollo del pensamiento crítico en el aula de ciencias [Teaching strategies for the development of critical thinking in the teaching of science]. Ápice. Revista de Educación Científica, 1 (1), 107–115. https://doi.org/10.17979/arec.2017.1.1.2004
Brigandt, I. (2016). Why the difference between explanation and argument matters to science education. Science & Education, 25 (3-4), 251–275. https://doi.org/10.1007/s11191-016-9826-6
Cáceres, M., Nussbaum, M., & Ortiz, J. (2020). Integrating critical thinking into the classroom: A teacher’s perspective. Thinking Skills and Creativity, 37 , 100674. https://doi.org/10.1016/j.tsc.2020.100674
Campanario, J. M., Moya, A., & Otero, J. (2001). Invocaciones y usos inadecuados de la ciencia en la publicidad [Invocations and misuses of science in advertising]. Enseñanza de las Ciencias, 19 (1), 45–56. https://doi.org/10.5565/rev/ensciencias.4013
Clouse, S. (2017). Scientific thinking is not critical thinking. https://medium.com/extra-extra/scientific-thinking-is-not-critical-thinking-b1ea9ebd8b31
Confederacion de Sociedades Cientificas de Espana [COSCE]. (2011). Informe ENCIENDE: Enseñanza de las ciencias en la didáctica escolar para edades tempranas en España [ENCIENDE report: Science education for early-year in Spain] . COSCE.
Costa, S. L. R., Obara, C. E., & Broietti, F. C. D. (2020). Critical thinking in science education publications: the research contexts. International Journal of Development Research, 10 (8), 39438. https://doi.org/10.37118/ijdr.19437.08.2020
Couso, D., Jiménez-Liso, M.R., Refojo, C. & Sacristán, J.A. (coords.) (2020). Enseñando ciencia con ciencia [Teaching science with science]. FECYT & Fundacion Lilly / Penguin Random House
Davidson, S. G., Jaber, L. Z., & Southerland, S. A. (2020). Emotions in the doing of science: Exploring epistemic affect in elementary teachers' science research experiences. Science Education, 104 (6), 1008–1040. https://doi.org/10.1002/sce.21596
Dean, D., & Kuhn, D. (2003). Metacognition and critical thinking. ERIC document. Reproduction No. ED477930 . https://files.eric.ed.gov/fulltext/ED477930.pdf
Díaz, C., & Cabrera, C. (2022). Desinformación científica en España . FECYT/IBERIFIER https://www.fecyt.es/es/publicacion/desinformacion-cientifica-en-espana
Dowd, J. E., Thompson, R. J., Jr., Schiff, L. A., & Reynolds, J. A. (2018). Understanding the complex relationship between critical thinking and science reasoning among undergraduate thesis writers. CBE—Life Sciences . Education, 17 (1), ar4. https://doi.org/10.1187/cbe.17-03-0052
Dwyer, C. P., Hogan, M. J., & Stewart, I. (2014). An integrated critical thinking framework for the 21st century. Thinking Skills and Creativity, 12 , 43–52. https://doi.org/10.1016/j.tsc.2013.12.004
Elliott, K. C., & McKaughan, D. J. (2014). Non-epistemic values and the multiple goals of science. Philosophy of Science, 81 (1), 1–21. https://doi.org/10.1086/674345
Ennis, R. H. (2018). Critical thinking across the curriculum: A vision. Topoi, 37 (1), 165–184. https://doi.org/10.1007/s11245-016-9401-4
Erduran, S. (2021). Respect for evidence: Can science education deliver it? Science & Education, 30 (3), 441–444. https://doi.org/10.1007/s11191-021-00245-8
European Commission. (2015). Science education for responsible citizenship . Publications Office https://op.europa.eu/en/publication-detail/-/publication/a1d14fa0-8dbe-11e5-b8b7-01aa75ed71a1
European Commission / Eurydice. (2011). Science education in Europe: National policies, practices and research . Publications Office. https://op.europa.eu/en/publication-detail/-/publication/bae53054-c26c-4c9f-8366-5f95e2187634
European Commission / Eurydice. (2022). Increasing achievement and motivation in mathematics and science learning in schools . Publications Office. https://eurydice.eacea.ec.europa.eu/publications/mathematics-and-science-learning-schools-2022
European Commission/Eurydice. (2006). Science teaching in schools in Europe. Policies and research . Publications Office. https://op.europa.eu/en/publication-detail/-/publication/1dc3df34-acdf-479e-bbbf-c404fa3bee8b
Fackler, A. (2021). When science denial meets epistemic understanding. Science & Education, 30 (3), 445–461. https://doi.org/10.1007/s11191-021-00198-y
García-Carmona, A. (2008). Relaciones CTS en la educación científica básica. II. Investigando los problemas del mundo [STS relationships in basic science education II. Researching the world problems]. Enseñanza de las Ciencias, 26 (3), 389–402. https://doi.org/10.5565/rev/ensciencias.3750
García-Carmona, A. (2014). Naturaleza de la ciencia en noticias científicas de la prensa: Análisis del contenido y potencialidades didácticas [Nature of science in press articles about science: Content analysis and pedagogical potential]. Enseñanza de las Ciencias, 32 (3), 493–509. https://doi.org/10.5565/rev/ensciencias.1307
García-Carmona, A., & Acevedo-Díaz, J. A. (2016). Learning about the nature of science using newspaper articles with scientific content. Science & Education, 25 (5–6), 523–546. https://doi.org/10.1007/s11191-016-9831-9
García-Carmona, A., & Acevedo-Díaz, J. A. (2016b). Concepciones de estudiantes de profesorado de Educación Primaria sobre la naturaleza de la ciencia: Una evaluación diagnóstica a partir de reflexiones en equipo [Preservice elementary teachers' conceptions of the nature of science: a diagnostic evaluation based on team reflections]. Revista Mexicana de Investigación Educativa, 21 (69), 583–610. https://www.redalyc.org/articulo.oa?id=14045395010
García-Carmona, A., & Acevedo-Díaz, J. A. (2017). Understanding the nature of science through a critical and reflective analysis of the controversy between Pasteur and Liebig on fermentation. Science & Education, 26 (1–2), 65–91. https://doi.org/10.1007/s11191-017-9876-4
García-Carmona, A., & Acevedo-Díaz, J. A. (2018). The nature of scientific practice and science education. Science & Education, 27 (5–6), 435–455. https://doi.org/10.1007/s11191-018-9984-9
García-Carmona, A. (2020). From inquiry-based science education to the approach based on scientific practices. Science & Education, 29 (2), 443–463. https://doi.org/10.1007/s11191-020-00108-8
García-Carmona, A. (2021a). Prácticas no-epistémicas: ampliando la mirada en el enfoque didáctico basado en prácticas científicas [Non-epistemic practices: extending the view in the didactic approach based on scientific practices]. Revista Eureka sobre Enseñanza y Divulgación de las Ciencias, 18 (1), 1108. https://doi.org/10.25267/Rev_Eureka_ensen_divulg_cienc.2021.v18.i1.1108
García-Carmona, A. (2021b). Learning about the nature of science through the critical and reflective reading of news on the COVID-19 pandemic. Cultural Studies of Science Education, 16 (4), 1015–1028. https://doi.org/10.1007/s11422-021-10092-2
Guerrero-Márquez, I., & García-Carmona, A. (2020). La energía y su impacto socioambiental en la prensa digital: temáticas y potencialidades didácticas para una educación CTS [Energy and its socio-environmental impact in the digital press: issues and didactic potentialities for STS education]. Revista Eureka sobre Enseñanza y Divulgación de las Ciencias, 17(3), 3301. https://doi.org/10.25267/Rev_Eureka_ensen_divulg_cienc.2020.v17.i3.3301
Gobert, J. D., Moussavi, R., Li, H., Sao Pedro, M., & Dickler, R. (2018). Real-time scaffolding of students’ online data interpretation during inquiry with Inq-ITS using educational data mining. In M. E. Auer, A. K. M. Azad, A. Edwards, & T. de Jong (Eds.), Cyber-physical laboratories in engineering and science education (pp. 191–217). Springer.
Chapter Google Scholar
Harlen, W. (2014). Helping children’s development of inquiry skills. Inquiry in Primary Science Education, 1 (1), 5–19. https://ipsejournal.files.wordpress.com/2015/03/3-ipse-volume-1-no-1-wynne-harlen-p-5-19.pdf
Hitchcock, D. (2017). Critical thinking as an educational ideal. In On reasoning and argument (pp. 477–497). Springer.
Hyytinen, H., Toom, A., & Shavelson, R. J. (2019). Enhancing scientific thinking through the development of critical thinking in higher education. In M. Murtonen & K. Balloo (Eds.), Redefining scientific thinking for higher education . Palgrave Macmillan.
Jiménez-Aleixandre, M. P., & Puig, B. (2022). Educating critical citizens to face post-truth: the time is now. In B. Puig & M. P. Jiménez-Aleixandre (Eds.), Critical thinking in biology and environmental education, Contributions from biology education research (pp. 3–19). Springer.
Jirout, J. J. (2020). Supporting early scientific thinking through curiosity. Frontiers in Psychology, 11 , 1717. https://doi.org/10.3389/fpsyg.2020.01717
Kanari, Z., & Millar, R. (2004). Reasoning from data: How students collect and interpret data in science investigations. Journal of Research in Science Teaching, 41 (7), 748–769. https://doi.org/10.1002/tea.20020
Klahr, D., Zimmerman, C., & Matlen, B. J. (2019). Improving students’ scientific thinking. In J. Dunlosky & K. A. Rawson (Eds.), The Cambridge handbook of cognition and education (pp. 67–99). Cambridge University Press.
Krell, M., Vorholzer, A., & Nehring, A. (2022). Scientific reasoning in science education: from global measures to fine-grained descriptions of students’ competencies. Education Sciences, 12 , 97. https://doi.org/10.3390/educsci12020097
Kuhn, D. (1993). Science as argument: Implications for teaching and learning scientific thinking. Science education, 77 (3), 319–337. https://doi.org/10.1002/sce.3730770306
Kuhn, D. (1999). A developmental model of critical thinking. Educational Researcher, 28 (2), 16–46. https://doi.org/10.3102/0013189X028002016
Kuhn, D. (2022). Metacognition matters in many ways. Educational Psychologist, 57 (2), 73–86. https://doi.org/10.1080/00461520.2021.1988603
Kuhn, D., Iordanou, K., Pease, M., & Wirkala, C. (2008). Beyond control of variables: What needs to develop to achieve skilled scientific thinking? Cognitive Development, 23 (4), 435–451. https://doi.org/10.1016/j.cogdev.2008.09.006
Kuhn, D., & Lerman, D. (2021). Yes but: Developing a critical stance toward evidence. International Journal of Science Education, 43 (7), 1036–1053. https://doi.org/10.1080/09500693.2021.1897897
Kuhn, D., & Modrek, A. S. (2022). Choose your evidence: Scientific thinking where it may most count. Science & Education, 31 (1), 21–31. https://doi.org/10.1007/s11191-021-00209-y
Lederman, J. S., Lederman, N. G., Bartos, S. A., Bartels, S. L., Meyer, A. A., & Schwartz, R. S. (2014). Meaningful assessment of learners' understandings about scientific inquiry—The views about scientific inquiry (VASI) questionnaire. Journal of Research in Science Teaching, 51 (1), 65–83. https://doi.org/10.1002/tea.21125
Lehrer, R., & Schauble, L. (2006). Scientific thinking and science literacy. In K. A. Renninger, I. E. Sigel, W. Damon, & R. M. Lerner (Eds.), Handbook of child psychology: Child psychology in practice (pp. 153–196). John Wiley & Sons, Inc.
López-Fernández, M. D. M., González-García, F., & Franco-Mariscal, A. J. (2022). How can socio-scientific issues help develop critical thinking in chemistry education? A reflection on the problem of plastics. Journal of Chemical Education, 99 (10), 3435–3442. https://doi.org/10.1021/acs.jchemed.2c00223
Magno, C. (2010). The role of metacognitive skills in developing critical thinking. Metacognition and Learning, 5 , 137–156. https://doi.org/10.1007/s11409-010-9054-4
McBain, B., Yardy, A., Martin, F., Phelan, L., van Altena, I., McKeowen, J., Pembertond, C., Tosec, H., Fratuse, L., & Bowyer, M. (2020). Teaching science students how to think. International Journal of Innovation in Science and Mathematics Education, 28 (2), 28–35. https://openjournals.library.sydney.edu.au/CAL/article/view/14809/13480
McIntyre, L. (2021). Talking to science deniers and sceptics is not hopeless. Nature, 596 (7871), 165–165. https://doi.org/10.1038/d41586-021-02152-y
Moore, C. (2019). Teaching science thinking. Using scientific reasoning in the classroom . Routledge.
Moreno-Fontiveros, G., Cebrián-Robles, D., Blanco-López, A., & y España-Ramos, E. (2022). Decisiones de estudiantes de 14/15 años en una propuesta didáctica sobre la compra de un coche [Fourteen/fifteen-year-old students’ decisions in a teaching proposal on the buying of a car]. Enseñanza de las Ciencias, 40 (1), 199–219. https://doi.org/10.5565/rev/ensciencias.3292
National Research Council [NRC]. (2012). A framework for K-12 science education . National Academies Press.
Network, I.-A. T. E. (2015). Critical thinking toolkit . OAS/ITEN.
Normand, M. P. (2008). Science, skepticism, and applied behavior analysis. Behavior Analysis in Practice, 1 (2), 42–49. https://doi.org/10.1007/BF03391727
Norris, S. P., Phillips, L. M., & Korpan, C. A. (2003). University students’ interpretation of media reports of science and its relationship to background knowledge, interest, and reading difficulty. Public Understanding of Science, 12 (2), 123–145. https://doi.org/10.1177/09636625030122001
Oliveras, B., Márquez, C., & Sanmartí, N. (2013). The use of newspaper articles as a tool to develop critical thinking in science classes. International Journal of Science Education, 35 (6), 885–905. https://doi.org/10.1080/09500693.2011.586736
Organisation for Economic Co-operation and Development [OECD]. (2019). PISA 2018. Assessment and Analytical Framework . OECD Publishing. https://doi.org/10.1787/b25efab8-en
Book Google Scholar
Organisation for Economic Co-operation and Development [OECD]. (2020). PISA 2024: Strategic Vision and Direction for Science. https://www.oecd.org/pisa/publications/PISA-2024-Science-Strategic-Vision-Proposal.pdf
Osborne, J., Pimentel, D., Alberts, B., Allchin, D., Barzilai, S., Bergstrom, C., Coffey, J., Donovan, B., Kivinen, K., Kozyreva, A., & Wineburg, S. (2022). Science Education in an Age of Misinformation . Stanford University.
Osborne, J. F., & Patterson, A. (2011). Scientific argument and explanation: A necessary distinction? Science Education, 95 (4), 627–638. https://doi.org/10.1002/sce.20438
Pols, C. F. J., Dekkers, P. J. J. M., & De Vries, M. J. (2021). What do they know? Investigating students’ ability to analyse experimental data in secondary physics education. International Journal of Science Education, 43 (2), 274–297. https://doi.org/10.1080/09500693.2020.1865588
Royal Decree 217/2022. (2022). of 29 March, which establishes the organisation and minimum teaching of Compulsory Secondary Education (Vol. 76 , pp. 41571–41789). Spanish Official State Gazette. https://www.boe.es/eli/es/rd/2022/03/29/217
Sagan, C. (1987). The burden of skepticism. Skeptical Inquirer, 12 (1), 38–46. https://skepticalinquirer.org/1987/10/the-burden-of-skepticism/
Santos, L. F. (2017). The role of critical thinking in science education. Journal of Education and Practice, 8 (20), 160–173. https://eric.ed.gov/?id=ED575667
Schafersman, S. D. (1991). An introduction to critical thinking. https://facultycenter.ischool.syr.edu/wp-content/uploads/2012/02/Critical-Thinking.pdf . Accessed 10 May 2023.
Sinatra, G. M., & Hofer, B. K. (2021). How do emotions and attitudes influence science understanding? In Science denial: why it happens and what to do about it (pp. 142–180). Oxford Academic.
Solbes, J., Torres, N., & Traver, M. (2018). Use of socio-scientific issues in order to improve critical thinking competences. Asia-Pacific Forum on Science Learning & Teaching, 19 (1), 1–22. https://www.eduhk.hk/apfslt/
Spektor-Levy, O., Eylon, B. S., & Scherz, Z. (2009). Teaching scientific communication skills in science studies: Does it make a difference? International Journal of Science and Mathematics Education, 7 (5), 875–903. https://doi.org/10.1007/s10763-009-9150-6
Taylor, P., Lee, S. H., & Tal, T. (2006). Toward socio-scientific participation: changing culture in the science classroom and much more: Setting the stage. Cultural Studies of Science Education, 1 (4), 645–656. https://doi.org/10.1007/s11422-006-9028-7
Tena-Sánchez, J., & León-Medina, F. J. (2022). Y aún más al fondo del “bullshit”: El papel de la falsificación de preferencias en la difusión del oscurantismo en la teoría social y en la sociedad [And even deeper into “bullshit”: The role of preference falsification in the difussion of obscurantism in social theory and in society]. Scio, 22 , 209–233. https://doi.org/10.46583/scio_2022.22.949
Tytler, R., & Peterson, S. (2004). From “try it and see” to strategic exploration: Characterizing young children's scientific reasoning. Journal of Research in Science Teaching, 41 (1), 94–118. https://doi.org/10.1002/tea.10126
Uskola, A., & Puig, B. (2023). Development of systems and futures thinking skills by primary pre-service teachers for addressing epidemics. Research in Science Education , 1–17. https://doi.org/10.1007/s11165-023-10097-7
Vallverdú, J. (2005). ¿Cómo finalizan las controversias? Un nuevo modelo de análisis: la controvertida historia de la sacarina [How does controversies finish? A new model of analysis: the controversial history of saccharin]. Revista Iberoamericana de Ciencia, Tecnología y Sociedad, 2 (5), 19–50. http://www.revistacts.net/wp-content/uploads/2020/01/vol2-nro5-art01.pdf
Vázquez-Alonso, A., & Manassero-Mas, M. A. (2018). Más allá de la comprensión científica: educación científica para desarrollar el pensamiento [Beyond understanding of science: science education for teaching fair thinking]. Revista Electrónica de Enseñanza de las Ciencias, 17 (2), 309–336. http://reec.uvigo.es/volumenes/volumen17/REEC_17_2_02_ex1065.pdf
Willingham, D. T. (2008). Critical thinking: Why is it so hard to teach? Arts Education Policy Review, 109 (4), 21–32. https://doi.org/10.3200/AEPR.109.4.21-32
Yacoubian, H. A. (2020). Teaching nature of science through a critical thinking approach. In W. F. McComas (Ed.), Nature of Science in Science Instruction (pp. 199–212). Springer.
Yacoubian, H. A., & Khishfe, R. (2018). Argumentation, critical thinking, nature of science and socioscientific issues: a dialogue between two researchers. International Journal of Science Education, 40 (7), 796–807. https://doi.org/10.1080/09500693.2018.1449986
Zeidler, D. L., & Nichols, B. H. (2009). Socioscientific issues: Theory and practice. Journal of elementary science education, 21 (2), 49–58. https://doi.org/10.1007/BF03173684
Zimmerman, C., & Klahr, D. (2018). Development of scientific thinking. In J. T. Wixted (Ed.), Stevens’ handbook of experimental psychology and cognitive neuroscience (Vol. 4 , pp. 1–25). John Wiley & Sons, Inc..
Download references
Conflict of Interest
The author declares no conflict of interest.
Funding for open access publishing: Universidad de Sevilla/CBUA
Author information
Authors and affiliations.
Departamento de Didáctica de las Ciencias Experimentales y Sociales, Universidad de Sevilla, Seville, Spain
Antonio García-Carmona
You can also search for this author in PubMed Google Scholar
Corresponding author
Correspondence to Antonio García-Carmona .
Additional information
Publisher’s note.
Springer Nature remains neutral with regard to jurisdictional claims in published maps and institutional affiliations.
Rights and permissions
Open Access This article is licensed under a Creative Commons Attribution 4.0 International License, which permits use, sharing, adaptation, distribution and reproduction in any medium or format, as long as you give appropriate credit to the original author(s) and the source, provide a link to the Creative Commons licence, and indicate if changes were made. The images or other third party material in this article are included in the article's Creative Commons licence, unless indicated otherwise in a credit line to the material. If material is not included in the article's Creative Commons licence and your intended use is not permitted by statutory regulation or exceeds the permitted use, you will need to obtain permission directly from the copyright holder. To view a copy of this licence, visit http://creativecommons.org/licenses/by/4.0/ .
Reprints and permissions
About this article
García-Carmona, A. Scientific Thinking and Critical Thinking in Science Education . Sci & Educ (2023). https://doi.org/10.1007/s11191-023-00460-5
Download citation
Accepted : 30 July 2023
Published : 05 September 2023
DOI : https://doi.org/10.1007/s11191-023-00460-5
Share this article
Anyone you share the following link with will be able to read this content:
Sorry, a shareable link is not currently available for this article.
Provided by the Springer Nature SharedIt content-sharing initiative
- Cognitive skills
- Critical thinking
- Metacognitive skills
- Science education
- Scientific thinking
- Find a journal
- Publish with us
- Track your research
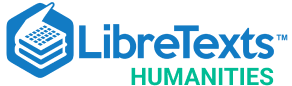
- school Campus Bookshelves
- menu_book Bookshelves
- perm_media Learning Objects
- login Login
- how_to_reg Request Instructor Account
- hub Instructor Commons
- Download Page (PDF)
- Download Full Book (PDF)
- Periodic Table
- Physics Constants
- Scientific Calculator
- Reference & Cite
- Tools expand_more
- Readability
selected template will load here
This action is not available.
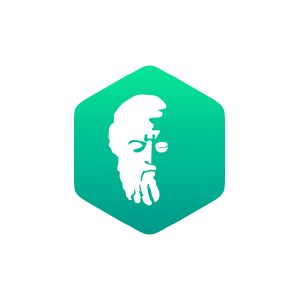
1: Knowledge and Skepticism
- Last updated
- Save as PDF
- Page ID 29951

- Golden West College via NGE Far Press
Philosophy 1
Philosophy (from Greek φιλοσοφία, philosophia , literally "love of wisdom") is the study of general and fundamental problems concerning matters such as existence, knowledge, values, reason, mind, and language. The term was probably coined by Pythagoras (c. 570 – c. 495 BC). Philosophical methods include questioning, critical discussion, rational argument and systematic presentation. Classic philosophical questions include: Is it possible to know anything and to prove it? What is most real? However, philosophers might also pose more practical and concrete questions such as: Is there a best way to live? Is it better to be just or unjust (if one can get away with it)? Do humans have free will?
Historically, "philosophy" encompassed any body of knowledge. From the time of Ancient Greek philosopher Aristotle to the 19th century, "natural philosophy" encompassed astronomy, medicine and physics. For example, Newton's 1687 Mathematical Principles of Natural Philosophy later became classified as a book of physics. In the 19th century, the growth of modern research universities led academic philosophy and other disciplines to professionalize and specialize. In the modern era, some investigations that were traditionally part of philosophy became separate academic disciplines, including psychology, sociology, linguistics and economics.
Other investigations closely related to art, science, politics, or other pursuits remained part of philosophy. For example, is beauty objective or subjective? Are there many scientific methods or just one? Is political utopia a hopeful dream or hopeless fantasy? Major sub-fields of academic philosophy include metaphysics ("concerned with the fundamental nature of reality and being"), epistemology (about the "nature and grounds of knowledge [and]...its limits and validity" ), ethics, aesthetics, political philosophy, logic, philosophy of science and the history of Western philosophy.
Since the 20th century professional philosophers contribute to society primarily as professors, researchers and writers. However, many of those who study philosophy in undergraduate or graduate programs contribute in the fields of law, journalism, politics, religion, science, business and various art and entertainment activities.
Introduction
Traditionally, the term "philosophy" referred to any body of knowledge. In this sense, philosophy is closely related to religion, mathematics, natural science, education and politics. Newton's 1687 "Mathematical Principles of Natural Philosophy" is classified in the 2000s as a book of physics; he used the term "natural philosophy" because it used to encompass disciplines that later became associated with sciences such as astronomy, medicine and physics.
Philosophy was traditionally divided into three major branches:
- Natural philosophy (" physics") was the study of the physical world ( physis, lit: nature);
- Moral philosophy (" ethics" ) was the study of goodness, right and wrong, beauty, justice and virtue ( ethos, lit: custom);
- Metaphysical philosophy ("logos") was the study of existence, causation, God, logic, forms and other abstract objects (" meta-physika" lit: "what comes after physics").
This division is not obsolete but has changed. Natural philosophy has split into the various natural sciences, especially astronomy, physics, chemistry, biology and cosmology. Moral philosophy has birthed the social sciences, but still includes value theory (including aesthetics, ethics, political philosophy, etc.). Metaphysical philosophy has birthed formal sciences such as logic, mathematics and philosophy of science, but still includes epistemology, cosmology and others.
Philosophical progress
Many philosophical debates that began in ancient times are still debated today. Colin McGinn and others claim that no philosophical progress has occurred during that interval. Chalmers and others, by contrast, see progress in philosophy similar to that in science, while Talbot Brewer argued that "progress" is the wrong standard by which to judge philosophical activity.
Historical overview
In one general sense, philosophy is associated with wisdom, intellectual culture and a search for knowledge. In that sense, all cultures and literate societies ask philosophical questions such as "how are we to live" and "what is the nature of reality". A broad and impartial conception of philosophy then, finds a reasoned inquiry into such matters as reality, morality and life in all world civilizations.
Western philosophy
Western philosophy is the philosophical tradition of the Western world and dates to Pre-Socratic thinkers who were active in Ancient Greece in the 6th century BC such as Thales (c. 624 – c. 546 BC) and Pythagoras (c. 570 – c. 495 BC) who practiced a "love of wisdom" ( philosophia ) and were also termed physiologoi (students of physis , or nature). Socrates was a very influential philosopher, who insisted that he possessed no wisdom but was a pursuer of wisdom. Western philosophy can be divided into three eras: Ancient (Greco-Roman), Medieval philosophy (Christian European), and Modern philosophy.
The Ancient era was dominated by Greek philosophical schools which arose out of the various pupils of Socrates, such as Plato who founded the Platonic Academy, and was one of the most influential Greek thinkers for the whole of Western thought. Plato's student Aristotle was also extremely influential, founding the Peripatetic school. Other traditions include Cynicism, Stoicism, Greek Skepticism and Epicureanism. Important topics covered by the Greeks included metaphysics (with competing theories such as atomism and monism), cosmology, the nature of the well-lived life (eudaimonia), the possibility of knowledge and the nature of reason (logos). With the rise of the Roman empire, Greek philosophy was also increasingly discussed in Latin by Romans such as Cicero and Seneca.
Medieval philosophy (5th – 16th century) is the period following the fall of the Roman empire and was dominated by the rise of Christianity and hence reflects Judeo-Christian theological concerns as well as retaining a continuity with Greco-Roman thought. Problems such as the existence and nature of God, the nature of faith and reason, metaphysics, the problem of evil were discussed in this period. Some key Medieval thinkers include St. Augustine, Thomas Aquinas, Boethius, Anselm and Roger Bacon. Philosophy for these thinkers was viewed as an aid to Theology ( ancilla theologiae ) and hence they sought to align their philosophy with their interpretation of sacred scripture. This period saw the development of Scholasticism, a text critical method developed in medieval universities based on close reading and disputation on key texts. The Renaissance (1355–1650) period saw increasing focus on classic Greco-Roman thought and on a robust Humanism.
Early modern philosophy in the Western world begins with thinkers such as Thomas Hobbes and René Descartes (1596–1650). Following the rise of natural science, Modern philosophy was concerned with developing a secular and rational foundation for knowledge and moved away from traditional structures of authority such as religion, scholastic thought and the Church. Major modern philosophers include Spinoza, Leibniz, Locke, Berkeley, Hume, and Kant. 19th-century philosophy is influenced by the wider movement termed the Enlightenment, and includes figures such as Hegel a key figure in German idealism, Nietzsche a famed anti-Christian, J.S. Mill who promoted Utilitarianism, Karl Marx who developed the foundations for Communism and the American William James. The 20th century saw the split between Analytic philosophy and Continental philosophy, as well as philosophical trends such as Phenomenology, Existentialism, Logical Positivism, Pragmatism and the Linguistic turn.

IMAGES
VIDEO
COMMENTS
Scientific thinking is a type of knowledge seeking involving intentional information seeking, including asking questions, testing hypotheses, making observations, recognizing patterns, and making inferences ( Kuhn, 2002; Morris et al., 2012 ). Much research indicates that children engage in this information-seeking process very early on through ...
Skepticism is a philosophy that traces to the ancient Greeks. It is based on the view that explanations of events are evaluated after collecting all relevant evidence and carefully considering all possibilities. Even then, the explanation should be considered only tentative. This, of course, is fully consistent with the role of hypotheses and ...
Scientific skepticism is a form of inquiry that uses scientific methods to investigate claims made in the absence of definitive proof. This type of skepticism is based on the premise that extraordinary claims require extraordinary evidence in order to be accepted as valid. ... Critical thinking is another important concept related to skepticism ...
A review of the research shows that critical thinking is a more inclusive construct than intelligence, going beyond what general cognitive ability can account for. For instance, critical thinking can more completely account for many everyday outcomes, such as how thinkers reject false conspiracy theories, paranormal and pseudoscientific claims ...
principles that guide the scientific enterprise. They include seeking conceptual (theoretical) understanding, posing empirically testable and refutable hypotheses, designing studies that test and can rule out competing counterhypotheses, using observational methods linked to theory that enable other scientists to verify their accuracy, and recognizing the importance of both independent ...
Both the scientific method and critical thinking are applications of logic and related forms of rationality that date to the Ancient Greeks. The full spectrum of critical/rational thinking includes logic, informal logic, and systemic or analytic thinking. This common core is shared by the natural sciences and other domains of inquiry share, and ...
Critical thinking and its importance. Critical thinking, defined here as "the ways in which one uses data and evidence to make decisions about what to trust and what to do" [], is a foundational learning goal for almost any undergraduate course and can be integrated in many points in the undergraduate curriculum.Beyond the classroom, critical thinking skills are important so that students ...
It's about being open to constructive criticism and new ideas. People who are sceptical do all of this as well—they challenge ideas and they withhold judgment until sufficient evidence is ...
What Is Scientific Inquiry and Why Teach It? Inquiry is at the heart of the scientific enterprise and, as such, demands a prominent position in science teaching and learning. The National Science Education Standards (NSES) refer to two important aspects of inquiry that are important to science instruction: Scientific inquiry refers to the ways ...
Critical Theory refers to a way of doing philosophy that involves a moral critique of culture. A "critical" theory, in this sense, is a theory that attempts to disprove or discredit a widely held or influential idea or way of thinking in society. Thus, critical race theorists and critical gender theorists offer critiques of traditional ...
What is Skepticism. The word "skepticism" comes from the ancient Greek skepsis, meaning "inquiry.". Skepticism is, therefore, not a cynical rejection of new ideas, as the popular stereotype goes, but rather an attitude of both open mind and critical sense. The ancient skeptics simply doubted that human beings can achieve certain ...
Skepticism. First published Sat Dec 8, 2001; substantive revision Wed Aug 31, 2005. Much of epistemology has arisen either in defense of or in opposition to various forms of skepticism. Indeed, one could classify various theories of knowledge by their responses to skepticism. For example, rationalists could be viewed as skeptical about the ...
These are cases of applying scientific skepticism. Skeptics value contributions of science but also those of logic and math that lead towards the best explanation. Skepticism can be applied to subjects such as history, art and literature, as well, by using critical thinking and respect for the evidence for any claims that are made.
It involves a deep knowledge of pseudoscience, the philosophy of science, mechanisms of deception, neuropsychological humility, scams, logic, and other aspects of critical thinking. This includes knowledge of the history of pseudoscience. Within skepticism, individuals also tend to focus their writing and speaking on their area of scientific ...
Skepticism in these domains does not entail that there is no God or that all moral claims are false. Rather, skepticism means that we can never be sufficiently justified in believing that there is a God or that moral claims are true. We simply can never know either way whether, for example, God exists.
Scientific thinking and critical thinking are two intellectual processes that are considered keys in the basic and comprehensive education of citizens. For this reason, their development is also contemplated as among the main objectives of science education. However, in the literature about the two types of thinking in the context of science education, there are quite frequent allusions to one ...
Philosophy (from Greek φιλοσοφία, philosophia, literally "love of wisdom") is the study of general and fundamental problems concerning matters such as existence, knowledge, values, reason, mind, and language. The term was probably coined by Pythagoras (c. 570 - c. 495 BC). Philosophical methods include questioning, critical ...
Dewey, who also used the term reflective thinking, connected critical thinking to a tradition of rational inquiry associated with modern science. From the turn of the 20th century, he and others working in the overlapping fields of psychology , philosophy , and educational theory sought to rigorously apply the scientific method to understand ...
The French philosopher Rene Descartes once wrote, "In order to seek truth, it is necessary once in the course of our life to doubt, as far as possible, all things.". Sometimes you need to ...
Answer and Explanation: 1. Become a Study.com member to unlock this answer! Create your account. View this answer. Critical thinking and skepticism are important to scientific inquiry because they prevent erroneous conclusions from being reached. Critical thinking... See full answer below.
Who's in the Video. Go to Profile. Thinking. Skepticism: Why critical thinking makes you smarter. Being skeptical isn't just about being contrarian. It's about asking the right questions of ...
Skeptics are not sure what has been proven true, to be true. They seek more proof possibly. These keep S.I. In a sort of checks & balances so the response or end goal stays true to science itself. Group-think, when everyone agrees on the solution without further inquiry, is very dangerous. It takes the solution down one path without ever ...
Erudite1. Critical thinking and skepticism are important to scientific inquiry because they help scientists to thoroughly examine all evidence before making their conclusions, this allows them to avoid errors. Critical thinking refers to the attitude of asking questions constantly, thoroughly examining information and evidence and making valid ...